Chapter 6 Ultrasound of the Brachial Plexus
The use of ultrasound for imaging the brachial plexus and its terminal branches has significantly increased since the first description of its use in anesthesiology for plexus blockade in 1989.1 With continuous improvement in imaging technology and evidence of the benefits of ultrasound for interventional approaches to, and diagnostic evaluation of, the brachial plexus, it is certain that the technology will increase its penetration into the routine clinical practice of anesthesiologists, neurologists, and musculoskeletal specialists in the coming years.
Equipment, Terminology, and Limitations of Ultrasound
The use of ultrasound for imaging of the brachial plexus has advantages over other imaging modalities, such as computed tomography (CT) and magnetic resonance imaging (MRI). Ultrasound equipment is relatively inexpensive and portable, and can be brought to the bedside for examination in patients who cannot readily be transported. The imaging of vascular and soft tissues around the plexus is excellent, and ultrasound allows for dynamic imaging with the upper extremity in various positions (as is done during imaging of the ulnar nerve at the elbow described in Chapter 5.) This dynamic imaging is ideal for interventional procedures with ultrasound guidance of needle placement, such as local anesthetic injection. Disadvantages of ultrasound imaging include the loss of resolution when imaging deeper structures requiring lower-frequency transducers, and the difficulty of imaging around bony structures, such as the intervertebral foramina, where bone will not allow penetration of ultrasound energy. An additional disadvantage is the requirements for knowledge, training, and perhaps certification in this new imaging modality for nonradiologists. The American and European Societies of Regional Anesthesia have made recommendations for ultrasound training curricula for residents and postgraduate practicing physicians.2
The terminology used for imaging and ultrasound-guided nerve block describes the probe-to-target orientation and the probe-to-needle orientation. Nerves and vascular structures are most easily located in a cross-sectional view, also known as short-axis. Because nerves display some anisotropy (see Chapter 1), a probe inclination of 90 degrees to the course of the target nerve produces the best image. After locating a nerve using the short-axis transducer position, rotation of the probe on its axis at 90 degrees produces a longitudinal or long-axis view (Fig. 6.1). Once a target nerve is identified in short-axis or long-axis view, a needle may be inserted using ultrasound guidance. If the needle is inserted perpendicular to the ultrasound plane such that only a bright spot is seen as the needle crosses the beam, this is known as out-of-plane. If the needle is inserted parallel to the ultrasound plane such that the tip and needle shaft are seen throughout the insertion, this is known as in-plane (Fig. 6.2). Both out-of-plane and in-plane techniques are used by anesthesiologists, each having advantages and disadvantages; neither has been proved to be superior.
Ultrasound Appearance of Tissues
The ultrasonographic appearance of normal nerves and other tissues has been well described.3 The epineurium and connective tissue within nerves appear hyperechoic, and nerve fascicles are relatively hypoechoic. In short-axis, nerves appear honeycomb like (Fig. 6.3), and in long-axis a hyperechoic epineurium is seen at the edges, with discontinuous internal hypoechoic tubular structures representing the fascicles (Fig. 6.4). This ultrasonographic appearance has been closely correlated to the histologic appearance.4 Transducer manipulation, particularly tilting, may also influence the hyper- or hypoechogenicity of nerve tissue, and this property is known as anisotropy. Pathologic nerves generally are enlarged, with a homogeneous hypoechoic appearance; the internal hyperechoic connective tissue pattern is lost and this is suggested to be because of peri- or intraneural edema.3 Tendon has an appearance most like nerve tissue, but with finer internal striations and greater anisotropy. Tendon may be distinguished from nerve by moving the ultrasound transducer proximal and distal along the target; nerve varies little, but tendon flattens and blends into muscle.
Normal muscle has a generally hypoechoic ultrasonographic appearance with internal hyperechoic connective tissue striations, and vascular structures are anechoic (blood-containing); arteries show pulsation and are difficult to compress, and veins are easily compressed and may show flow or size changes with respiration. Bone has a hyperechoic cortical surface and transmits little ultrasound energy such that shadowing is apparent deep to the bone surface (Fig. 6.5). Air is also a poor ultrasound conductor, and ultrasound imaging through air-containing structures is unsatisfactory.
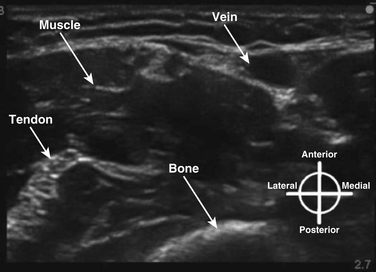
Fig. 6.5 Ultrasound appearance of nonneural tissues, with vein, muscle, tendon, and bone identified.
Supraclavicular Anatomy
The ultrasonographic appearance of the brachial plexus is best understood by first reviewing the gross anatomic three-dimensional (3D) appearance of the elements of the plexus and adjacent structures. With this knowledge, the expected appearance in sequential two-dimensional anatomic “cuts” of the brachial plexus can be predicted, and correlated with the ultrasound images at the same location. A review paper has recently shown the correlations of anatomic cross section, histology, and ultrasound appearance of the brachial plexus.5 Many of the gross anatomic cross-sectional images included here as figures were made after access of the brachial artery and basilic vein at the elbows of unembalmed cadavers. Fluid infused under pressure was used to distend and identify vessels during ultrasound imaging. Later, injection of liquid red and blue latex into the arterial and venous systems was performed prior to freezing and sectioning for cross-sectional specimens.
The roots exit the cervical spine sandwiched between the anterior scalene muscle, originating from the anterior tubercles of the transverse processes of C3-6 and inserting on the first rib between the subclavian artery and vein, and the middle scalene muscle, originating from the posterior tubercles of the transverse processes of C2-7 and inserting on the first rib posterior to the plexus (Fig. 6.6). Anterior to the anterior scalene muscle and deep to the sternocleidomastoid muscle is the carotid sheath containing the common carotid artery and internal jugular vein. The formation of the upper trunk from C5 and C6, the middle trunk as the continuation of C7, and the lower trunk from C8 and T1 are commonly described at the lateral edge of the scalene muscles. The three trunks cross the first rib closely approximated to the posterior surface of the subclavian artery, and this is where the plexus is most compact and readily and completely blocked by injection of local anesthetic; this is known as a supraclavicular block (see following section). Each trunk separates into anterior and posterior divisions, and these divisions may occur proximal (Fig. 6.7), on, or distal to the first rib.
The cross-sectional anatomic appearance of the neck can be predicted from the previously described relationships, and correlated with the ultrasound appearance. If an anatomic section is made, or a high-frequency ultrasound probe positioned short-axis to the course of the brachial plexus roots at approximately the level of the cricoid cartilage (C6 level) (Fig. 6.8), the trachea is seen anteriorly, adjacent to the (usually) homogeneous hyperechoic thyroid gland. Posterolateral to the thyroid is the carotid sheath, and the common carotid artery, internal jugular vein, and vagus nerve may be identified. Posterolateral to the carotid sheath, the scalene muscles and brachial plexus are located deep to the sternocleidomastoid. The cross-sectional appearance of the scalene muscles is characteristic and helps identify the cervical roots passing between them (Fig. 6.9). The best ultrasound image is usually obtained with a modest caudad inclination of the transducer such that it is perpendicular to the plexus. The roots appear as round or oval hypoechoic structures with few internal echoes. A significantly higher fascicle–to–connective tissue ratio above the clavicle compared with below has been demonstrated (Fig. 6.10), and because the epineurium and connective tissue separating the hypoechoic fascicles is hyperechoic, this may explain the relative hypoechoic appearance of brachial plexus elements above the clavicle compared with a hyperechoic appearance distal to the clavicle (Fig. 6.11).6 The transverse processes of the cervical spine can be identified by their hyperechoic cortical edge and shadowing deep to their surface, with the root seen between the anterior and posterior tubercles (Fig. 6.12). The characteristic absence of the anterior tubercle of C7 has been used to identify the level of the roots imaged.7 The vertebral artery and vein are often seen adjacent to the C7 transverse process and can also be useful in identifying root level (Fig. 6.13). Once the root level is determined, the probe may be rotated 90 degrees to achieve a long-axis view and visualize the root exit zones (Fig. 6.14). The phrenic nerve is sometimes seen adjacent to the C5 root, diverging from it anteriorly and traveling just superficial to the anterior scalene fascia as the transducer is moved caudad (Fig. 6.15).8
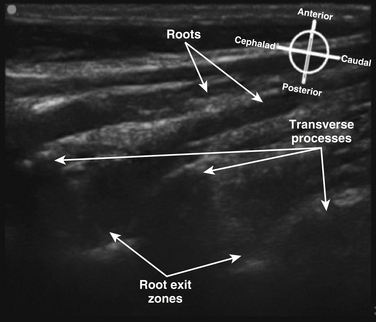
Fig. 6.14 Ultrasound long-axis view of the brachial plexus roots in the neck, achieved by 90-degree rotation of the probe after obtaining the short-axis view shown in Figure 6.11. The roots curve out from the transverse processes and become more superficial distally.