30 Troubleshooting of Implantable Cardioverter-Defibrillators
Since its introduction into clinical practice in the early 1980s, the implantable cardioverter-defibrillator (ICD) has evolved from a treatment of last resort for aborted cardiac arrest to the treatment of choice for the management of resuscitated cardiac arrest and, more recently, for the primary prevention of sudden cardiac death. The original device (AID, Intec Systems, Pittsburgh) lacked programmability except for turning the device on or off. Its detection rate was fixed, and it had no diagnostic capabilities except to indicate that a charging cycle had been initiated. With the emergence of additional therapeutic capabilities, including bradycardia and antitachycardia pacing, the need for diagnostic capabilities and features has increased exponentially with growth in the rate of ICD implantation. The need to recognize and define the initiating events and resulting rhythms at the time of ICD intervention has led to the development of sophisticated telemetry features. Other requirements include diagnosing ICD malfunction noninvasively and evaluating the performance of the ICD components (e.g., battery and lead integrity). This chapter reviews the available methods for the diagnosis of ICD malfunction using the programmer and noninvasive techniques. Only conventional ICDs are discussed here; Chapter 31 discusses biventricular devices.
Troubleshooting Principles
Malfunction of ICDs is uncommon. The most common reasons for inappropriate therapy or the absence of expected therapy relate to inappropriate programming, lead-related complications, imperfect diagnostic specificity, insufficient understanding of the technical specifications of device function, and drug-device interactions. Device-device interactions and true component malfunctions now rarely occur.1
Analysis of ICD problems begins with identification of the problem, followed by analysis of its possible cause. Presentation falls into four general areas: multiple shocks, failure to convert ventricular tachycardia (VT) or ventricular fibrillation (VF), failure to detect VT or VF, and problems with pacing.2
Initial Evaluation and Tools
Physical Examination
The physical examination may be helpful in pinpointing the exact cause of ICD malfunction. For example, for a patient who reports that certain body positions or movements elicit ICD shocks, reproducing the precise maneuver or the exact circumstances known to elicit the shocks, while simultaneously telemetering the device, may prove the diagnosis of lead failure. Occasionally, patients are reluctant to allow the examiner to do this because the prospect of an ICD shock is psychologically threatening.3 In such circumstances, the ICD may be placed in a monitor mode, either by programming or using a magnet to suspend tachyarrhythmia detection, while assuring the patient that shock delivery will not occur. The assessment of recordings from the ICD in these situations is discussed later.
Because a common point of lead fracture occurs where transvenous ICD leads pass between the clavicle and first rib,4,5 manipulation of the device pocket or the lead entry point in the pectoral area may elicit electrical noise artifact, indicating lead conductor fracture.6,7 Similar artifacts may occur with a loose connection of the set screw to the lead terminal pin in the ICD pulse generator header. Again, make-break potentials can be demonstrated by noninvasive telemetry, as discussed later.
Other diagnostic clues may be provided by the physical examination, such as detection of an irregular pulse suggestive of atrial fibrillation, which may be readily confirmed by an electrocardiogram. Congestive heart failure is often associated with exacerbation of ventricular arrhythmias.8–10 Therefore, eliciting symptoms and signs of left ventricular decompensation suggests a possible cause for worsening arrhythmias.
Electrocardiographic Recordings
Objective evidence of the event precipitating ICD therapy, or of an arrhythmia with absence of the expected ICD response, can be extremely helpful and is usually diagnostic (Fig. 30-1). In the days before the availability of stored electrograms (EGMs), such evidence was rarely available unless the patient was hospitalized and monitored at the time of the event. Fortunately, this is now a problem of the past. Nevertheless, even when available, EGMs can sometimes be inconclusive or confusing. In addition, if multiple ICD detections have occurred for any reason, including lead failure, SVT, or a VT or VF storm, EGM documentation of the initial event leading to detection may be overwritten because of limited device memory.
Device Telemetry
The advent of device telemetry has revolutionized and greatly simplified analysis of arrhythmias and events suspected of representing ICD malfunction. Early devices (e.g., Ventak, Cardiac Pacemakers [CPI/Guidant], St. Paul, Minn.) were able to emit sounds or beeps synchronous with ventricular electrogram (VEGM) detection, enabling identification of oversensing or undersensing. When recorded with a phonocardiogram, a so-called “beep-o-gram” was produced. Although helpful, these crude attempts at telemetry were quickly supplanted by advances that enabled detailed information, such as R-R intervals and VEGMs, to be telemetered11 (Fig. 30-2). The complexity and sophistication of these diagnostic tools are increasing with the new generation of ICDs. The addition of the atrial channel has greatly simplified analysis of arrhythmic events (Fig. 30-3).
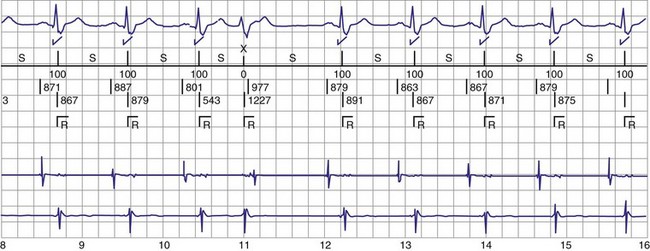
Figure 30-3 As in Figure 30-2, this tracing EGM recorded at a routine clinic follow-up visit shows the ventricular EGM (fourth tracing) as well as an atrial EGM (third tracing) from a dual-chamber Model V242 ICD (St. Jude Medical). The first and second tracings are, respectively, a surface electrocardiogram (ECG) and a marker channel. In the marker channel, the checks indicate a template match with sinus rhythm (100% registered below the horizontal marker line). The fourth beat, marked x, is a premature ventricular depolarization with a different morphology than sinus; it is logged as a 0% match. The next two rows of numbers are the P-P and R-R intervals. S indicates sinus rhythm, as defined by the ICD, as opposed to T or F if ventricular tachycardia or fibrillation were present, respectively. R indicates sensed ventricular activity. The data on atrial and ventricular EGM timing and ventricular EGM morphology allow this device to use discrimination algorithms to differentiate supraventricular from ventricular arrhythmias. It can also function as an atrial, ventricular, or dual-chamber pacemaker.
Other diagnostic information is now routinely available from all ICDs, including battery voltage, pacing and sensing lead impedances, charge times, capacitor re-formation times, high-voltage lead impedance, and frequency and timing of ventricular events. The finding of a depleted battery, lead impedance out of range (either too high or too low), and R-R intervals can lead to correct diagnoses. The latter is extremely important, because nonphysiologic intervals (short) raise the suspicion of make-brake electrical noise artifact. One device (Medtronic, Minneapolis) records information regarding both impedance changes (Fig. 30-4) and nonphysiologic R-R intervals.12
Newer ICDs from all major manufacturers are capable of remote device telemetry from the patient’s home.13,14 This capability offers two advantages over exclusively office-based interrogation. The first advantage is the ability to interrogate the ICD more quickly and easily after a shock. The second advantage is that most remote monitoring frequently assesses lead impedance changes and other parameters that may herald lead failure. The Lumos-T Reduces Routine Office Device Follow-Up Study (TRUST) showed that remote monitoring with automatic daily surveillance provides early detection of device events.15–17 Once a potential lead failure is identified by the device, the physician can be notified quickly, often quickly enough to intervene before lead failure produces a clinical event (i.e., shock for lead fracture). The CONNECT Trial demonstrated that remote follow-up of ICD significantly reduced the time from onset of clinical events (new-onset atrial or ventricular arrhythmias, lead or device integrity problems) to clinical decisions compared with usual outpatient clinic follow-up.18 Unfortunately, some device failures occur with adverse events happening so rapidly, such as inappropriate shocks, that early notification is impossible.19 Notably, even if mechanisms such as audible tones are available for patient notification, with increasing age, more patients are unable to hear the alerts.20
Radiographic Evidence
Radiographic evidence is helpful if lead malposition, dislodgment, or fracture is suspected.7 It is recommended, whenever possible, that chest radiographs taken immediately after ICD implantation be compared with radiographs obtained at the time of a problem. Such comparisons may reveal lead malposition and displacement when none is suspected. Radiographs can also be helpful in demonstrating conductor fracture or pin connectors improperly positioned in the header. Examining the radiographic “signature” of the device can help identify the device type when the patient is unaware of the ICD model or type. Knowledge of unique failure modes such as “migration” of set screws (travel of the right ventricular fixation screw into the channel lumen during shipment) in particular families of ICDs is of course desirable.21
Figures 30-5 and 30-6 show examples of lead dislodgment. Because the distal end of the lead is close to the tricuspid anulus (Fig. 30-5, A), both atrial and ventricular signals are recorded (B). This leads to “double-counting” such that, for a given heart rate, the ventricular channel registers twice the actual heart rate, satisfying the high rate detection requirement and leading to administration of a shock. Figure 30-6 shows a similar example, but the electrical artifacts are more disorganized and irregular, presumably because the lead is more free floating. Figure 30-7 shows an example of disruption of a transvenous ICD lead as it passes between the clavicle and first rib. Care must be taken to learn the appearance of the welds of the springs, because they can be mistaken for fracture if their usual appearance of being offset from the central axis of the lead is not appreciated22 (Fig. 30-8). An unusual cause of lead failure, and sometimes failure of telemetry, occurs in twiddler’s syndrome; in these cases, a lead is twisted and fractured or the ICD is inverted23,24 (Fig. 30-9). Lead perforation can occur subacutely or rarely chronically, leading to several problems, including undersensing of ventricular arrhythmias, failure to convert ventricular arrhythmias, and failure of pacing as the lead tip exits the myocardium (Fig. 30-10).
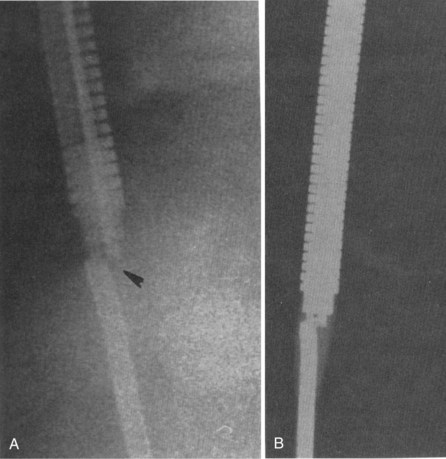
Figure 30-8 Lead fracture.
(From Kratz JM, Schabel S, Leman RM: Pseudo fracture of a defibrillating lead. Pacing Clin Electrophysiol 18:2225-2226, 1985.)
Multiple Shocks
Multiple shocks may be the result of incessant VT/VF, repetitive VT/VF, oversensing of T waves, electromagnetic interference, electrical noise artifacts from a fractured conductor or myopotentials (in the case of insulation failure), or SVT, including sinus tachycardia, atrial fibrillation, atrial flutter, or another mechanism (Table 30-1). Sometimes the ICD itself can be proarrhythmic (Box 30-1).
Cause | Management |
---|---|
Incessant/repetitive VT or VF | Judicious use of magnet and/or programming ICD off. |
Supraventricular tachycardia (SVT) | Reprogram; treat arrhythmia; slow ventricular response to AF. |
Change in substrate | Treat precipitating factor. |
Ischemia | Revascularize; administer drug therapy. |
Drug change | Reprogram, or change drug. |
Sensing Malfunction | |
Lead failure (conductor or insulation) | Replace lead. |
Loose connection (e.g., set screw) | Reoperate, and reseat screw. |
T-wave sensing | Reprogram as feasible. |
P-wave sensing | Reprogram as feasible, but often requires reoperation. |
External Signals | |
Electromagnetic interference (EMI) | Avoid cause. |
Device-device interaction | Reprogram, or reoperate. |
AF, Atrial fibrillation; VF, ventricular fibrillation; VT, ventricular tachycardia.
Box 30-1
Classification of ICD-Induced Proarrhythmia
Modified from Pinski SL, Fahy GJ: The proarrhythmic potential of implantable cardioverter-defibrillators. Circulation 92:1651-1664, 1995.
The occurrence of multiple shocks leads to myriad problems. Shocks are poorly tolerated by patients, families, and referring physicians and can create long-lasting fear and distrust of the ICD requiring professional help. Furthermore, shocks lead to significant increases in cost of therapy by repeated hospitalizations and testing sessions; shocks may jeopardize the physician-patient relationship. ICD shocks, both appropriate and inappropriate, have also been associated with increased mortality.25–27 The occurrence of repetitive shocks for whatever reason represents a medical emergency.
Perhaps the most difficult causes of multiple shocks to treat are incessant VT and VF. On the one hand, delivered therapy is appropriate; on the other hand, it is not only a horrible experience for patients but also reflects a substrate problem. ICDs do not treat heart disease, only its consequences. Initial management centers on the administration of drug therapy,8,9,28,29 and the ICD usually should be disabled by programming or magnet application. Although antiarrhythmic drugs may be useful, particularly intravenous amiodarone, the judicious use of β-blockers has been found to be extremely beneficial.9,29 If VT or VF episodes are repetitive or incessant despite drug therapy, it is often advisable to intubate and sedate the patient, disable the ICD, and use an external defibrillator to manage the arrhythmia events. Intra-aortic balloon counterpulsation has been used on occasion with success. With the increased efficacy of radiofrequency ablation, incessant arrhythmias may be so treated.30
Oversensing of P waves, T waves, and even atrial flutter may lead to repetitive shocks.31–33 Atrial oversensing in the ventricle is uncommon; it is usually seen with integrated bipolar leads when the proximal spring is close to or straddling the tricuspid valve31,32 (Fig. 30-11). P-wave sensing may also be seen when a lead is dislodged to the right atrioventricular junction, as in Figure 30-5. More often, T waves are sensed because of their large size relative to the R wave, but sometimes simply because the T wave is huge, as in a patient with hypertrophic cardiomyopathy (Fig. 30-12). Rarely, T-wave oversensing occurs during rate-related bundle branch block,34 hyperglycemia,35 and the Brugada syndrome.36
First reported in the mid-1990s, myopotentials can also be sensed and interpreted as a ventricular arrhythmia, leading to inappropriate shock therapy. These may arise from the pectoral muscles37,38 or from the diaphragm.39,40 The latter has occurred with integrated bipolar leads that presumably lie near the respiratory muscles (Fig. 30-13). An unusual case of inappropriate shock therapy caused by pectoral muscle myopotential oversensing involving an integrated bipolar lead occurred with high-voltage pin reversal in the device header41 (Fig. 30-14).
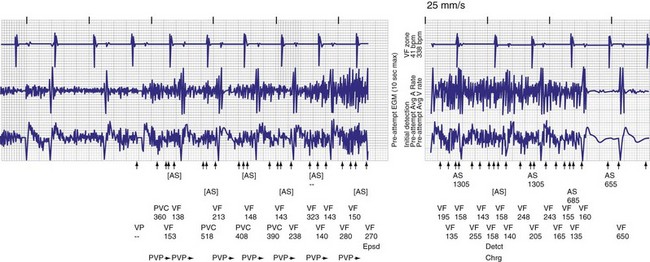
Figure 30-14 Myopotential sensing.
(From Allison JS, Epstein AE, Plumb VJ: Electrical noise artifact: from without or within? J Cardiovasc Electrophysiol 18:332-333, 2007.)
Oversensing can often be rectified by altering the sensitivity or refractory period of the ICD. However, if T waves are large and R waves are small, changing the sensitivity will not suffice. If the sensitivity is decreased, VF may not be identified (Fig. 30-15). Similarly, lockouts imposed by the device to ensure arrhythmia detection limits programming of device refractory periods. One manufacturer provides greater programmability to overcome these problems. Because ICDs, in contrast to pacemakers, vary their sensitivity to identify ventricular activity, altering the amplitude and timing at which sensitivity is adjusted can address this problem.
For the patient with hypertrophic cardiomyopathy shown in Figure 30-12, the R wave was greater than 25 mV and the T wave greater than 4 mV. If the sensitivity were decreased significantly, VF might not be reliably sensed. Furthermore, because of prolonged repolarization and sensing of the terminal portion of the T wave, prolonging the ventricular refractory period would not suffice to correct this problem. In this case, avoidance of T-wave oversensing was achieved by altering the threshold start, the decay delay, and the sensitivity. Alternatively, on rare occasions an ICD lead may need to be repositioned or a separate sensing lead placed in a different area of the right ventricle.
In anticipation of T-wave oversensing in the future, one approach that may be useful is to test VF detection at low sensitivity at implantation. If oversensing occurs, sensitivity may be decreased without the need for retesting of defibrillation sensing. On the other hand, whenever sensitivity is decreased from a level that has not been tested and shown to provide adequate detection of VF, it is imperative to retest VF detection with an electrophysiologic study to demonstrate adequate sensing (see Fig. 30-15).
The sensing of external electrical activity may lead to false detection. Because of the increased use of ICDs and relatively low lead failure rates, the most common cause (after lead failure, discussed later) is probably electrocautery42,43 (Fig. 30-16). Other causes include electronic surveillance systems,42,44 razors,45 radiofrequency handheld remote controls,46 ungrounded electrical tools,42 fluid in a connector port,47 transcutaneous electrical nerve stimulation (TENS; Fig. 30-17),48 personal digital assistants (PDAs),49 and even slot machines50 and washing machines.51
A common cause of multiple shocks is lead failure.6,28,52–60 When make-break potentials are recorded by the ICD, the rapid electrical activity is interpreted as VF. This is one of the easiest and most characteristic problems encountered (Fig. 30-18). Note the nonphysiologic (extremely rapid) electrical activity that is the signature of this phenomenon. Lead failure may become apparent after shocks.55
ICD leads have a higher failure rate than pacemaker leads because they are an order of magnitude more complex. In addition, some models have especially high failure rates, including both epicardial leads53 and endocardial leads.54–56 One reported case had a 37% failure rate (confidence interval, 24%-54%) at 68.6 months; the study showed that failure may not become apparent until late follow-up, but also that a “signature” of failure is the occurrence of electrical noise artifact after shock delivery.55 Failure is not predicted by R-wave changes or changes in pacing characteristics. However, combining the occurrence of an abnormal impedance with a counter showing R-R intervals shorter than 140 msec can identify ICD lead failure with high sensitivity and specificity.12
The most recent lead to manifest a higher-than-anticipated failure rate is the Medtronic 6949.56 Conductor fracture causing make-break potentials interpreted as VF is the most frequent mode of failure for this lead, although high-voltage conductor failure has also been reported. Both inappropriate shocks and inhibition of pacing can occur. As expected, changes in lead impedance and nonphysiologic R-R intervals precede lead failure. For patients with the Medtronic 6949 lead, recommended changes to device programming improved early detection of lead failure and minimized inappropriate shocks. Early detection changes included narrowing the acceptable range for normal lead impedance and tracking the number of sensing integrity counter (SIC) events.57 Lead impedances falling outside the established limits or too many SIC events triggered both an audible patient alarm and physician notification by the patient’s remote monitor. Because the make-break signals usually generated by a lead fracture tend to be nonsustained in character, increasing the number of R-R intervals to trigger device detection of a sustained ventricular arrhythmia serves to minimize inappropriate ICD shocks. The safety of such an extension in arrhythmia detection time is discussed later. However, as previously discussed, despite these programming changes, lead failure can occur quickly, and inappropriate ICD shocks might still be delivered.18
Supraventricular arrhythmias conducting to the ventricles above the ICD detection rate are common causes of multiple shocks.61 In this instance, device therapy does not reflect malfunction, but rather an appropriate response to tachycardia. The incidence of inappropriate shocks is higher in patients receiving an ICD for primary prevention than in those receiving secondary prevention.62,63 In the Sudden Cardiac Death in Heart Failure Trial (SCD-HeFT), 829 patients with class II or III congestive heart failure received an ICD for primary prevention. Of those, 259 (31%) received an ICD shock, and 32% of the shocks were inappropriate. Therefore, 10% of the population receiving a prophylactic ICD received inappropriate shocks.64
Atrial fibrillation (AF) is the most common cause of multiple shocks in patients undergoing ICD implantation for primary prevention.63 AF can be recognized by its EGM characteristics; the VEGM most often has the same morphology as in sinus rhythm (or AF, if it is the baseline) and is irregularly irregular (Fig. 30-19). In contrast, polymorphic VT, although irregularly irregular, has varying EGM morphologies (Fig. 30-20).
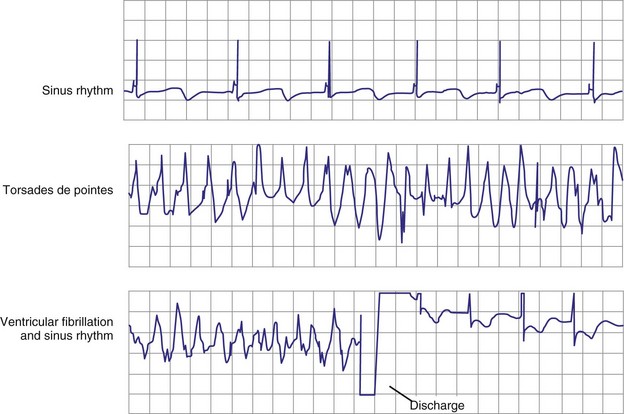
Figure 30-20 This stored electrogram (EGM) is from a 12-year-old boy with the familial long QT syndrome who had an ICD implanted after resuscitation from a cardiac arrest. After he collapsed in school, ICD interrogation showed sinus rhythm (note long QT even on EGM) followed by torsades de pointes ventricular tachycardia (VT), characterized by a polymorphic and constantly changing morphology, and degeneration to ventricular fibrillation (VF). The VF was terminated by a shock, which is labeled on the third tracing. In contrast to the tracing shown in Figure 30-1, there was no monomorphic VT preceding VF.
(From Moss AJ, Daubert JP: Images in clinical medicine: Internal ventricular defibrillation. N Engl J Med 342:398, 2000.)
Detection of atrial arrhythmias may be managed by both drug therapy and device programming. Beta-adrenergic blockers are a mainstay of therapy to decrease atrioventricular (AV) conduction and are antiarrhythmic themselves. Antiarrhythmic drugs may be prescribed to decrease the frequency of arrhythmia recurrence. Sotalol65 and azimilide66 have been efficacious in this regard, and although they avoid the organ toxicities associated with amiodarone, azimilide was never market released because it caused neutropenia. ICD programming to avoid inappropriate therapies for supraventricular arrhythmias depend in part on whether a single- or dual-lead system is present.67–69 For single-lead systems, detection enhancements to recognize rate stability and sudden onset are useful. For dual-lead systems, these parameters are used in addition to functions that assess AV relationships.
It is controversial whether the addition of an atrial lead improves tachycardia discrimination.70–72 Multiple reports beginning in the 1990s, when stored EGMs became available, consistently show that the most common cause of inappropriate shocks is SVT.61 Despite the presence of an atrial lead, the risk of inappropriate shocks from atrial arrhythmias remains about 20% to 30%. In the Antiarrhythmics Versus Implantable Defibrillators (AVID) study, in which single-chamber ICDs were primarily used, the incidence of shocks was 11% for AF and 9% for other SVTs.62 In a randomized trial that compared the incidence of inappropriate therapies from single-chamber versus dual-chamber ICDs, 21% of dual-chamber patients received inappropriate therapy despite having detection enhancements enabled. Theuns et al.71 randomly assigned patients with dual-chamber ICDs to single- or dual-chamber detection. Overall, there was no difference in atrial and ventricular arrhythmia discrimination between the two settings. Figure 30-19 shows an EGM recorded from a patient in whom AF triggered an ICD shock despite a 100% template match with a supraventricular rhythm; despite the match, the timer withholding therapy expired, overriding the discriminator.
Multiple shocks may also be a proarrhythmic response to drugs or to the ICD itself. Repetitive VT and VF can be a consequence of antiarrhythmic drug proarrhythmia. In addition, attention to the history may reveal the ingestion of a cardiac stimulant.73,74 Proarrhythmia may also be caused by the ICD itself.75–77 Pinski and Fahy76 review types of device proarrhythmia (see Box 30-1). A common cause of VT induction is undersensing of an ectopic beat, followed by a paced beat and subsequent induction of the ventricular arrhythmia (Fig. 30-21). Himmrich et al.77 studied patients with a history of tachycardia caused by right ventricular backup pacing and randomized them to no pacing or to VVI backup pacing at 60 bpm. No further pacemaker-induced tachycardias occurred in patients with pacing turned off, but did occur in five of six patients with pacemaker turned on. The induced arrhythmias were both monomorphic and polymorphic VTs.
A recent development in programmability to decrease the delivery of shock therapy for VT is the option for antitachycardia pacing (ATP) for extremely rapid VTs. Previously it was believed that such an approach would not only be inefficacious but also unsafe. In a prospective randomized trial comparing ATP with simple shock therapy for fast VTs, however, Wathen et al.78,79 showed that, for VTs in the range of 188 to 250 beats per minute, ATP was effective in terminating 81% (72% adjusted). Also, empirical ATP was equally safe and associated with improved quality of life compared with shock therapy for fast VTs.
The morbidity of inappropriate shocks, both from supraventricular arrhythmias and from nonsustained ventricular arrhythmias, led to further efforts to define the most appropriate programming for patients receiving ICDs for primary prevention. Wilkoff et al.80 showed that empirical programming designed to reduce ICD shocks for SVT and nonsustained VTs as well as empirical ATP performed at least as well as programming tailored to the individual patient by the implanting physician, in terms of reducing unnecessary shocks and avoiding undertreatment of sustained VTs. The authors then extended these findings to a more diverse group of patients undergoing ICD implantation.81 The effectiveness of longer detection times (30 of 40 beats) was further tested in terms of avoiding unnecessary shocks, as well as the safety of such a detection time in terms of arrhythmic syncope and undertreated ventricular arrhythmias. Compared with a control group of patients with devices programmed to treat much slower arrhythmias, the longer detection times and use of ATP did reduce the number of patients receiving inappropriate therapy, but at a slight increase in the number of patients experiencing arrhythmic syncope.
A strategy of prophylactic catheter ablation was recently shown to reduce ICD therapy by 65% in a group of patients with ischemic cardiomyopathy who received an ICD after presenting with sustained ventricular arrhythmias.82 The generalizability of this finding to patients with nonischemic cardiomyopathy and prior sustained ventricular arrhythmias, or patients receiving ICDs for primary prevention, has not been examined.
Other Presentations
Failure to Convert Ventricular Tachycardia or Ventricular Fibrillation
Problems related to the myocardial substrate, ICD programming, or ICD function are the usual reasons for failure to convert an arrhythmia. Changes in substrate may include ischemia resulting in polymorphic ventricular arrhythmias or VF, worsened heart failure that causes new arrhythmias or conversion to incessant ones, and any combination of events that may increase the pacing, cardioversion, or defibrillation thresholds (Table 30-2).
TABLE 30-2 Nonconversion of Ventricular Tachycardia (VT) or Ventricular Fibrillation (VF)
Cause | Management |
---|---|
Problems with VT therapy | For both VT and VF problems: |
Pacing algorithm | Change therapy prescription. |
Elevated pacing/cardioversion threshold | Decrease time to therapy. Reverse polarity. Change current path. Change waveform. Change drugs that affect rate or DFT. Treat myocardial substrate. |
Incessant VT | |
Problems with VF therapy | |
Elevated defibrillation threshold | |
Fixed voltage/pulse width Incessant VF | |
Faulty lead system: Dislodgment, fracture, loose connector | Revise lead system |
ICD failure | Replace ICD. |
Battery depletion | Replace ICD. |
ICD-pacemaker interaction | Revise system or reprogram if feasible. |
Abandoned electrode shunting energy | Extract extra lead. |
DFT, Defibrillation threshold.
Problems with therapy most often include the programming of ineffective pacing or shock therapies. ATP may be either underaggressive or overaggressive, resulting in failure of conversion or arrhythmia acceleration.75–77 If ventricular arrhythmias persist, they may become self-perpetuating because of ischemia and myocardial stretch exacerbated by hemodynamic compromise. These factors, as well as the arrhythmia duration itself, may also elevate the defibrillation threshold (DFT).83 Other factors that may affect shock efficacy relate not only to the amount of delivered energy but also to the shock waveform. For example, efficacy may be improved by altering the tilt of the shock waveform. Evidence indicates that a 50%/50% tilt is more efficacious than the nominal 65%/65% waveform.84 St. Jude Medical devices allow for optimization of the biphasic waveform by varying the duration of each phase of the shock to accommodate the individual patient’s DFT. These observations are not transferable to the waveforms of all manufacturers. For Medtronic devices, a randomized comparison of biphasic waveforms with 65%/65% versus 42%/42% tilt found no difference in the DFT.85
A related factor is the position of the ICD lead in the right ventricle. Although adequate defibrillation can occur with many different lead positions, failure to convert VF can sometimes be addressed by moving the lead to a more apical position that then encompasses more of the heart in the shock vector.86 Likewise, the efficacy of placement of a subcutaneous lead or array on lowering the DFT can be influenced by the placement of the array and its relation to the heart.87 Lead perforation can lead to inadequate defibrillation by altering the shock vector (see Fig. 30-10).
Therapy may also fail if there is component failure of either the ICD (uncommon but reported)1 or any of its leads. In the past, defective crystal oscillators led to rapid ventricular pacing, VF, and inability to deliver shock therapy.88 More recently, electrical overstress (EOS) has led to ICD failure.89 EOS is the damage to electrical components caused by high voltages or currents that arc within a pulse generator. In addition, recent occurrences of disabling of ICDs have been caused not only by electromagnetic interference but also by health care professionals unaware that magnet application might permanently inactivate tachycardia detection.90
Although interactions between separately implanted pacemakers are less common today, in the past they did interfere with ICD function. Although it was more common for a pacemaker to inhibit detection by asynchronously pacing during VF62 (Fig. 30-22), pacing may also reinitiate VT or VF after ICD therapy.
Antiarrhythmic drugs are often used in conjunction with ICD therapy (Box 30-2). These agents not only have beneficial effects of decreasing arrhythmia occurrence, but also may affect ICD detection and therapy (Figs. 30-23 and 30-24). Sodium channel blockers and amiodarone may increase the DFT, rendering therapy ineffective.91–93
Box 30-2
Effects of Antiarrhythmic Drugs on ICDs
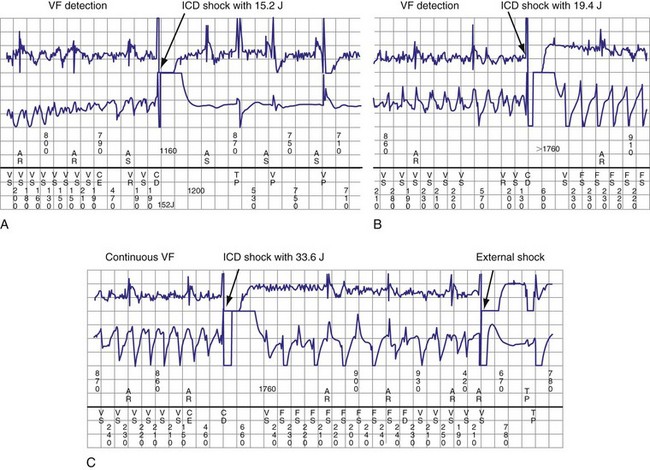
Figure 30-24 Amiodarone increasing defibrillation threshold (DFT).
(From Rajawat YS, Patel VV, Gerstenfeld EP, et al: Advantages and pitfalls of combining device-based and pharmacologic therapies for the treatment of ventricular arrhythmias: Observations from a tertiary referral center. Pacing Clin Electrophysiol 27:1670-1681, 2004.)
Because remote monitoring has not been available until recently for ICD follow-up94,95 and warning features (e.g., beeping by the ICD on achievement of the elective replacement indicator),96 battery depletion may also prevent delivery of effective therapy.
Failure to Detect Ventricular Tachycardia or Ventricular Fibrillation
The first question to ask when an arrhythmia is not detected is whether the ICD system is activated. Is a magnet over the device, or has one been used to permanently turn it off?90 Second, if the arrhythmia is ongoing, is its rate below the ICD detection rate? Has a new drug been started (e.g., amiodarone or a class IC agent) that has slowed the tachycardia rate below the detection rate (see Fig. 30-24)? Has a new drug provoked a new VT, such as torsades de pointes VT? Is the battery depleted?
The most common reasons for nondetection are inappropriate programming (Table 30-3). Lead dysfunction may also prevent arrhythmia detection. As previously noted, the presence of a second device (e.g., pacemaker) may lead to detection inhibition if sensing of separate pacemaker stimulus artifacts is interpreted by the ICD as a “normal rhythm.” VT and VF may be undersensed97–99 (see Fig. 30-15). VF nondetection or underdetection is usually caused by low-amplitude VF signals (i.e., below sensitivity of device). This is more common with integrated bipolar leads than with dedicated bipolar leads.78 As mentioned, lead perforation can result in both undersensing of ventricular arrhythmias and failure of pacing as the lead tip exits the myocardium (see Fig. 30-10).
TABLE 30-3 Nondetection of Ventricular Tachycardia (VT) or Ventricular Fibrillation
Cause | Management |
---|---|
Improper/phantom programming (e.g., is system activated?) | Reprogram. |
VT below detection rate: | |
New VT | Reprogram detection rate. |
VT slowed by drug(s) | Change drugs. |
Detection enhancements | Disable; reprogram. |
Undersensing: | |
Deterioration of R wave | Increase sensitivity. |
Oversensing of pacemaker stimulus artifacts | Decrease sensitivity; reposition lead. |
Magnet application | Remove magnet. |
Battery depletion | Replace device. |
Detection enhancements were developed to decrease the frequency of inappropriate shocks caused by SVT (Table 30-4); the literature is mixed on their efficacy. However, the requirement that criteria other than rate must be satisfied before initiation of arrhythmia therapy increases the possibility of falsely failing to detect a VT. Swerdlow et al.99 identified six mechanisms of underdetection of VT caused by algorithms to discriminate VT from supraventricular rhythms and nonsustained VT: (1) onset not satisfied (e.g., gradual onset, especially slower ones in sinus tachycardia), (2) stability not satisfied (e.g., irregular-cycle VT length not satisfying a rate stability criterion to exclude a false diagnosis of AF with a rapid ventricular response as VT or VF), (3) isolated long intervals, (4) VT-VF competition, (5) failure to reconfirm VT, and (6) post-VF suspension of VT detection. Currently, another reason for nondetection is morphology of the endocardial EGM similar to that of supraventricular origin. Importantly, the degree of morphology “match” used to distinguish between the template “normal” EGM and the “arrhythmia” EGM is both programmable and as a nominal value less than 100%, often much less, to account for rate-related changes in EGM morphology.100,101 Experience with these algorithms, however, suggests that there may be errors in classification resulting from morphology changes with time, after shocks, or caused by bundle branch block.102,103
TABLE 30-4 Detection Enhancements
Manufacturer | Dual-Chamber Discrimination Enhancements |
---|---|
Biotronik (Berlin) | SMART Detection Algorithm: Ventricular versus atrial rates, P-P and R-R stability, AV relationship and multiplicity, and suddenness of onset |
ELA Medical (Sorin Group, Milan) | PARAD Algorithm: R-R stability, P-R association, and ventricular acceleration |
Guidant (Boston Scientific, Natick, Mass.) | Suddenness of onset, rate stability, atrial fibrillation rate and stability, and ventricular rate versus atrial rates, Rhythm ID |
Medtronic (Minneapolis, Minn.) | PR Logic Algorithm: Pattern of A-V and V-A intervals; atrial and ventricular rates; evidence for atrial fibrillation, AV dissociation, and ventricular R-R irregularity; analysis for R-wave sensing in atrial channel; VEGM morphology |
St. Jude Medical (St. Paul, Minn.) | Suddenness of onset, AV association and relation of atrial and ventricular rates, interval stability, and VEGM morphology |
AV, Atrioventricular; VEGM, ventricular electrogram.
A newly recognized cause of underdetection is the application of algorithms to smooth and stabilize the ventricular rate during pacing.104–107 The common denominator of this mechanism of underdetection is intradevice interaction between pacing parameters and tachycardia detection, which usually leads to the undersensing of ventricular events during blanking periods while pacing. For example, during rate smoothing, to avoid ventricular sensing of atrial stimuli, after each atrial stimulus the ventricular channel is blanked for a fixed interval. In addition, after each ventricular stimulus, the ventricular channel is blanked again. When rate smoothing is activated to decrease symptoms secondary to varying heart rates, ventricular blanking after rapid atrial pacing can lead to nondetection of VT.
Problems with Pacing
Current ICDs provide both bradycardia and antitachycardia pacing therapies. Pacing for either indication may fail because of lead dysfunction or a change in the pacing threshold resulting from a change in substrate or physiologic milieu, such as addition of a drug (e.g., flecainide) that increases pacing threshold.93 Radiofrequency ablation may cause not only inappropriate shock therapy but also inhibition of pacing. Although theoretically radiofrequency energy may alter the lead-tissue interface and increase the pacing threshold, this has not been observed.108 However, magnetic resonance imaging (MRI) can increase the pacing threshold and alter component function.109
As in arrhythmia nondetection, the most common cause of abnormal pacing for bradycardia is inappropriate programming (Table 30-5). Pacing may be inhibited by T-wave110 and even far-field P-wave sensing in the ventricular channel. As described earlier, oversensing usually leads to inappropriate shock therapy rather than inhibition of pacing.31–33,111–113 Programming may be both the cause and the remedy for sensing problems. Certain St. Jude Medical ICDs permit separate sensitivity programming for bradycardia and tachycardia detection. This can be an important consideration for patients who are pacemaker dependent and at risk for ventricular oversensing of either P waves or noncardiac electrical signals.
Cause | Management |
---|---|
Increased pacing threshold | Reprogram, occasionally replace lead. |
T-wave sensing inhibits ventricular pacing | Reprogram ventricular refractory period or sensitivity. |
R-wave sensing inhibits atrial pacing | Reprogram atrial refractory period or sensitivity. |
Atrial electrical activity inhibits ventricular pacing | Decrease sensitivity or replace lead. |
Faulty lead system | Revise lead system. |
A serious cause of abnormal pacing is lead failure, as discussed earlier with regard to the production of electrical noise artifact and inappropriate shocks.6,28,52–60 ICD leads are complex, with more components than simple pacing leads. This complexity increases the chance for failure. Failure may occur in the conductor, insulation, or connector pins. The fault may be in the header, the yoke, or body of the lead within the vascular system. With subclavian approaches to lead implantation, crush is possible.4,5 Although the devices are large, “twiddling” may occur23,24 (see Fig. 30-9). Also, leads may become dislodged (see Figs. 30-5 and 30-6).
Other Issues
As in all fields of medicine, technologic advances and progress in defibrillation and pacing bring new and unanticipated problems. The use of MRI has expanded, and although its use generally is contraindicated in patients with an ICD, studies now report on its safety. A completely protected ICD has yet to be developed. Although some clinicians suggest MRI scanning may be safe with newer devices,109,114–116 it is probably premature to make this a general conclusion.117,118 Not only have interactions been reported,120 but series purporting device safety have included only small numbers of patients with relatively few devices. Indeed, specific device programming protocols designed to minimize device malfunction are used at centers evaluating the safety and efficacy of MRI scanning on ICD patients.109,114–116,119 To extrapolate these findings to the general population would require much greater numbers. Furthermore, even proponents of MRI safety with ICDs acknowledge that “minimal” changes in pacing threshold and battery function may occur.109 Although a small change in pacing threshold or battery function may be acceptable most of the time, it can be disastrous if safety margins are small, as when batteries are near their end of life or in patients with borderline pacing thresholds. Safety concerns such as those listed must be carefully weighed against the usefulness of information obtained from the MRI scan. Interactions with new scanners having field strengths greater than 1.5 tesla remain unknown.
Current ICDs are well shielded from external shocks. Nevertheless, cardioversion or defibrillation may injure the device. Therefore, whenever external shocks are given, paddles must be placed as far from the ICD as possible. In an emergency situation, patient resuscitation obviously takes precedence over saving the device. In addition, other medical equipment may injure an ICD, specifically shock wave lithotripsy.121 The beam should always be aimed away from the ICD if possible.
Importantly, psychological issues must be considered when taking care of patients with ICDs, and psychological problems certainly fall into the realm of troubleshooting.122,123 With patients who have received shocks, imagined shocks, and “phantom shocks,” the shock may cause concern for them and their families. Explanation is usually satisfactory, but sometimes fear of shocks can be debilitating, similar to a posttraumatic stress disorder. Indeed, patients receiving ICD shocks can have a worse prognosis than ICD patients who have not received an ICD shock.25–27 Besides counseling, drug therapy may be warranted to decrease anxiety and depression. Further investigation is needed to determine ways to reduce the morbidity associated with ICD shocks. The combination of a benzodiazepine and serotonin reuptake inhibitor is especially useful.
1 Hauser RG, Kallinen L. Deaths associated with implantable cardioverter defibrillator failure and deactivation reported in the United States Food and Drug Administration Manufacturer and User Facility Device Experience Database. Heart Rhythm. 2004;4:399-405.
2 Epstein AE. Approach to the patient with suspected implantable cardioverter-defibrillator system malfunction. J Cardiovasc Electrophysiol. 1998;2:330-333.
3 Ballas SL, Rashidi R, McAlister H, et al. The use of beep-o-grams in the assessment of automatic implantable cardioverter defibrillator sensing function. Pacing Clin Electrophysiol. 1989;12:1737-1745.
4 Magney JE, Parsons JA, Flynn DM, Hunter DW. Pacemaker and defibrillator lead entrapment: case studies. Pacing Clin Electrophysiol. 1995;18:1509-1517.
5 Gallik DM, Ben-Sur U, Gross JN, Furman S. Lead fracture in cephalic versus subclavian approach with transvenous implantable cardioverter defibrillator systems. Pacing Clin Electrophysiol. 1996;19:1089-1094.
6 Lawton JS, Ellenbogen KA, Wood MA, et al. Sensing lead-related complications in patients with transvenous implantable cardioverter-defibrillators. Am J Cardiol. 1996;78:647-651.
7 Gupta A, Zegel HG, Dravid VS, et al. Value of radiography in diagnosing complications of cardioverter defibrillators implanted without thoracotomy in 437 patients. AJR Am J Roentgenol. 1997;168:105-108.
8 Exner DV, Pinski SL, Wyse DG, et al. Electrical storm presages nonsudden death: the Antiarrhythmics versus Implantable Defibrillators (AVID) Trial. AVID Investigators. Circulation. 2001;103:2066-2071.
9 Credner SC, Klingenheben T, Mauss O, et al. Electrical storm in patient with transvenous implantable cardioverter-defibrillators: incidence, management and prognostic implications. J Am Coll Cardiol. 1998;32:1909-1915.
10 Whang W, Mittleman MA, Rich DQ, et al. Heart failure and the risk of shocks in patients with implantable cardioverter defibrillators: results from the Triggers of Ventricular Arrhythmias (TOVA) study. TOVA Investigators. Circulation. 2004;109:1386-1391.
11 Marchlinski FE, Callans DJ, Gottlieb CD, et al. Benefits and lessons learned from stored electrogram information in implantable defibrillators. J Cardiovasc Electrophysiol. 1995;6:832-851.
12 Gunderson BD, Patel AS, Bounds CA, et al. An algorithm to predict implantable cardioverter-defibrillator lead failure. J Am Coll Cardiol. 2004;44:1898-1902.
13 Jung W, Rillig A, Birkemeyer R, et al. Advances in remote monitoring of implantable pacemakers, cardioverter-defibrillators and cardiac resynchronization therapy systems. J Interv Card Electrophysiol. 2008;23:73-85.
14 Orlov MV, Szombathy T, Chaudhry GM, Haffajee CI. Remote surveillance of implantable cardiac devices. Pacing Clin Electrophysiol. 2009;32:928-939.
15 Varma N. Rationale and design of a prospective study of the efficacy of a remote monitoring system used in implantable cardioverter defibrillator follow-up: the Lumos-T Reduces Routine Office Device Follow-Up Study (TRUST). Am Heart J. 2007;154:1029-1034.
16 Varma N, Epstein AE, Irimpen A, et al. Early detection of ICD events using remote monitoring: the TRUST trial [abstract]. Heart Rhythm. 2009;6:S125.
17 Varma N, Epstein AE, Schweikert RA, et al. Evaluation of efficacy and safety of remote monitoring for ICD follow-up: the TRUST trial [abstract]. Circulation. 2008;118:2316.
18 Crossley GH et al: The Clinical Evaluation of Remote Notification to Reduce Time to Clinical Decision (CONNECT) trial: the value of remote monitoring. Abstract presented at American College of Cardiology’s 59th Annual Scientific Session’s Late Breaking Clinical Trials, 2010.
19 Kallinen LM, Hauser RG, Lee KW, et al. Failure of impedance monitoring to prevent adverse clinical events caused by fracture of a recalled high-voltage implantable cardioverter-defibrillator lead. Heart Rhythm. 2008;5:775-779.
20 Simons EC, Feigenblum DY, Nemirovsky D, Simons GR. Alert tones are frequently inaudible among patients with implantable cardioverter-defibrillators. Pacing Clin Electrophysiol. 2009;32:1272-1275.
21 Prickett RA, Saavedra P, Ali MF, et al. Implantable cardioverter-defibrillator malfunction due to mechanical failure of the header connection. J Cardiovasc Electrophysiol. 2004;14:1095-1099.
22 Kratz JM, Schabel S, Leman RM. Pseudo fracture of a defibrillating lead. Pacing Clin Electrophysiol. 1985;18:2225-2226.
23 Beauregard LAM, Russo AM, Heim J, et al. Twiddler’s syndrome complicating automatic defibrillator function. Pacing Clin Electrophysiol. 1995;18:735-738.
24 Boyle NG, Anselme F, Monahan KM, et al. Twiddler’s syndrome variants in ICD patients. Pacing Clin Electrophysiol. 1998;21:2685-2687.
25 Moss AJ, Greenberg H, Case RB, Zareba W, et al. Long-term clinical course of patients after termination of ventricular tachyarrhythmia by an implanted defibrillator. Multicenter Automatic Defibrillator Implantation Trial-II (MADIT-II) Research Group. Circulation. 2004;110:3760-3765.
26 Poole JE, Johnson GW, Hellkamp AS, Anderson J, et al. Prognostic importance of defibrillator shocks in patients with heart failure. N Engl J Med. 2008;359:1009-1017.
27 Sweeney MO, Sherfesee L, DeGroot PJ, et al. Differences in effects of electrical therapy type for ventricular arrhythmias on mortality in implantable cardioverter-defibrillator patients. Heart Rhythm. 2010;7:353-360.
28 Miller JM, Hsia HH. Management of the patient with frequent discharges from implantable cardioverter defibrillator devices. J Cardiovasc Electrophysiol. 1996;7:278-285.
29 Nademanee K, Taylor R, Bailey WE, et al. Treating electrical storm: sympathetic blockade versus advance cardiac life support–guided therapy. Circulation. 2000;102:742-747.
30 Marchlinski FE, Callans DJ, Gottlieb CD, Zado E. Linear ablation lesions for control of unmappable ventricular tachycardia in patients with ischemic and nonischemic cardiomyopathy. Circulation. 2000;101:1288-1296.
31 Peters W, Kowallik P, Wittenberg G, et al. Inappropriate discharge of an implantable cardioverter-defibrillator during atrial flutter and intermittent ventricular antibradycardia pacing. J Cardiovasc Electrophysiol. 1997;8:1167-1174.
32 Scaglione J, Socas AG, De Palma C, Kreutzer E. Inappropriate single chamber ICD discharges due to supraventricular tachycardia with high degree atrioventricular block. J Interv Card Electrophysiol. 2004;11:73-76.
33 Kelly PA, Mann DE, Damle RS, Reiter MJ. Oversensing during ventricular pacing in patients with a third-generation implantable cardioverter-defibrillator. J Am Coll Cardiol. 1994;23:1531-1534.
34 Coyne RF, Man DC, Sarter BH, et al. Implantable cardioverter-defibrillator oversensing during periods of rate-related bundle branch block. J Cardiovasc Electrophysiol. 1997;8:807-811.
35 Krishen A, Shepard RK, Leffler JA, et al. Implantable cardioverter defibrillator T wave oversensing caused by hyperglycemia. Pacing Clin Electrophysiol. 2001;24:1701-1703.
36 Porres JM, Brugada J, Marco P, et al. T wave oversensing by a cardioverter defibrillator implanted in patient with the Brugada syndrome. Pacing Clin Electrophysiol. 2004;27:1563-1565.
37 Sandler MJ, Kutalek SP. Inappropriate discharge by an implantable cardioverter defibrillator: recognition of myopotentials sensing using telemetered intracardiac electrograms. Pacing Clin Electrophysiol. 1994;17:665-671.
38 Karanam S, John RM. Inappropriate sensing of pectoral myopotentials by an implantable cardioverter-defibrillator system. Pacing Clin Electrophysiol. 2004;27:688-689.
39 Sweeney MO, Ellison KE, Shea JB, Newell JB. Provoked and spontaneous high-frequency, low-amplitude, respirophasic noise transients in patients with implantable cardioverter-defibrillators. J Cardiovasc Electrophysiol. 2001;12:402-410.
40 Schulte B, Sperzel J, Carlsson J, et al. Inappropriate arrhythmia detection in implantable defibrillator therapy due to oversensing of diaphragmatic myopotentials. J Interv Card Electrophysiol. 2001;5:487-493.
41 Allison JS, Epstein AE, Plumb VJ. Electrical noise artifact: from without or within? J Cardiovasc Electrophysiol. 2007;18:332-333.
42 Pinski SL, Trohman RG. Interference in implanted cardiac devices. Part 1. Pacing Clin Electrophysiol. 2002;25:1367-1381.
43 Casavant D, Haffajee C, Stevens S, Pacetti P. Aborted implantable cardioverter-defibrillator shock during facial electrosurgery. Pacing Clin Electrophysiol. 1998;21:1325-1326.
44 Matthew P, Lewis C, Neglia J, et al. Interaction between electronic article surveillance systems and implantable defibrillators: insights from a fourth-generation ICD. Pacing Clin Electrophysiol. 1997;20:2857-2859.
45 Seifert T, Block M, Borggrefe M, Breithardt G. Erroneous discharge of an implantable cardioveter-defibrillator caused by an electric razor. Pacing Clin Electrophysiol. 1995;18:1592-1594.
46 Man KC, Davidson T, Langberg JJ, et al. Interference from a hand-held radiofrequency remote control causing discharge of an implantable defibrillator. Pacing Clin Electrophysiol. 1993;16:1756-1758.
47 Heif C, Podczeck A, Krohner K, et al. Cardioverter discharges following sensing of electrical artifact due to fluid penetration in the connector port. Pacing Clin Electrophysiol. 1995;18:1589-1591.
48 Crevenna R, Stix G, Pleiner J, et al. Electromagnetic interference by transcutaneous neuromuscular electrical stimulation in patients with bipolar sensing implantable cardioverter defibrillators: a pilot safety study. Pacing Clin Electrophysiol. 2003;26:626-629.
49 Tri JL, Trusty JM, Hayes DL. Potential for personal digital assistant interference with implantable cardiac devices. Mayo Clin Proc. 2004;79:1527-1530.
50 Madrid A, Sánchez A, Bosch E, et al. Dysfunction of implantable defibrillators caused by slot machines. Pacing Clin Electrophysiol. 1997;20:212-214.
51 Sabaté X, Moure C, Nicholás J, et al. Washing machine associated 50 Hz detected as ventricular fibrillation by an implanted cardioverter-defibrillator. Pacing Clin Electrophysiol. 2001;24:1281-1283.
52 Lawton JS, Wood MA, Gilligan DM, et al. Implantable transvenous cardioverter-defibrillator leads: the dark side. Pacing Clin Electrophysiol. 1996;19:1273-1278.
53 Brady PA, Friedman PA, Trusty JM, et al. High failure rate for an epicardial implantable cardioverter-defibrillator lead: implications for long-term follow-up of patients with an implantable cardioverter-defibrillator. J Am Coll Cardiol. 1998;31:616-622.
54 Stambler BS, Wood MA, Damiano RJ, et al. Sensing/pacing lead complications with a newer generation implantable cardioverter-defibrillator: worldwide experience from the Guardian ATP 4210 Multicenter Clinical Trial. J Am Coll Cardiol. 1994;23:123-132.
55 Ellenbogen KA, Wood MA, Shepard RK, et al. Detection and management of an implantable cardioverter-defibrillator lead failure: Incidence and clinical implications. J Am Coll Cardiol. 2003;41:73-80.
56 Hauser RG, Kallinen LM, Almquist AK, et al. Early failure of a small-diameter high-voltage implantable cardioverter-defibrillator lead. Heart Rhythm. 2007;4:892-896.
57 Swerdlow CD, Gunderson BD, Ousdigian KT, et al. Downloadable algorithm to reduce inappropriate shocks caused by fractures of implantable cardioverter-defibrillator leads. Circulation. 2008;118:2122-2129.
58 Dorwarth U, Frey B, Dugas M, et al. Transvenous defibrillation leads: high incidence of failure during long-term follow-up. J Cardiovasc Electrophysiol. 2003;14:38-43.
59 Hauser RG, Cannom D, Hayes DL, et al. Long-term structural failure of coaxial polyurethane implantable cardioverter defibrillator leads. Pacing Clin Electrophysiol. 2002;25:879-882.
60 Kettering K, Mewis C, Dörnberger V, et al. Long-term experience with subcutaneous ICD leads: a comparison among three different types of subcutaneous leads. Pacing Clin Electrophysiol. 2004;27:1355-1361.
61 Grimm W, Flores BF, Marchlisnski FE. Electrocardiographically documented unnecessary, spontaneous shocks in 241 patients with implantable cardioverter-defibrillators. Pacing Clin Electrophysiol. 1992;15:1667-1673.
62 Klein RC, Raitt MH, Wilkoff BL, et al. Analysis of implantable cardioverter defibrillator therapy in the Antiarrhythmics versus Implantable Defibrillators (AVID) Trial. The AVID Investigators. J Cardiovasc Electrophysiol. 2003;14:940-948.
63 Daubert JP, Zareba W, Cannom DS, et al. Frequency and mechanism of inappropriate implantable cardioverter-defibrillator therapy in MADIT-II. J Am Coll Cardiol. 2004;43:132A.
64 Bardy GH, Lee KL, Mark DB, et al. Amiodarone or an implantable cardioverter-defibrillator for congestive heart failure. The Sudden Cardiac Death in Heart Failure Trial (SCD-HeFT) Investigators. N Engl J Med. 2005;352:225-237.
65 Pacifico A, Hohnloser SH, Williams JH, et al. The d,l-Sotalol Implantable Cardioverter-Defibrillator Study Group. N Engl J Med. 1999;340:1855-1862.
66 Singer I, Al-Khalidi H, Niazi I, et al. Azimilide decreases recurrent ventricular tachyarrhythmias in patients with implantable cardioverter defibrillators. J Am Coll Cardiol. 2004;43:39-43.
67 Swerdlow CD, Chen PS, Kass RM, et al. Discrimination of ventricular tachycardia from sinus tachycardia and atrial fibrillation in a tiered-therapy cardioverter-defibrillator. J Am Coll Cardiol. 1994;23:1342-1355.
68 Dorian P, Philippon F, Thibault B, et al. Randomized controlled study of detection enhancements versus rate-only detection to prevent inappropriate therapy in a dual-chamber implantable cardioverter-defibrillator. The ASTRID Investigators. Heart Rhythm. 2004;1:540-547.
69 Hintringer F, Deibl M, Berger T, et al. Comparison of the specificity of implantable dual chamber defibrillator detection algorithms. Pacing Clin Electrophysiol. 2004;27:976-982.
70 Swerdlow CD. Supraventricular tachycardia–ventricular tachycardia discrimination algorithms in implantable cardioverter-defibrillators: state-of-the-art review. J Cardiovasc Electrophysiol. 2001;12:606-612.
71 Theuns DA, Klootwijk AP, Goedhart DM, Jordaens LJ. Prevention of inappropriate therapy in implantable cardioverter-defibrillators: results of a prospective, randomized study of tachyarrhythmia detection algorithms. J Am Coll Cardiol. 2004;44:2362-2367.
72 Theuns DA, Rivero-Ayerza M, Boersma E, Jordaens L. Prevention of inappropriate therapy in implantable defibrillators: A meta-analysis of clinical trials comparing single-chamber and dual-chamber arrhythmia discrimination algorithms. Int J of Cardiology. 2008;125:352-357.
73 Goldstein DS, Epstein AE. A shocking case of pseudoephedrine use. J Interv Card Electrophysiol. 1999;3:343-344.
74 McBride BF, Guertin D, White CM, Kluger J. Inappropriate implantable cardioverter-defibrillator discharge following consumption of a dietary weight loss supplement. Pacing Clin Electrophysiol. 2004;27:1317-1320.
75 Johnson NJ, Marchlinski FE. Arrhythmias induced by device antitachycardia therapy due to diagnostic nonspecificity. J Am Coll Cardiol. 1991;18:1418-1425.
76 Pinski SL, Fahy GJ. The proarrhythmic potential of implantable cardioverter-defibrillators. Circulation. 1995;92:1651-1664.
77 Himmrich E, Przibille O, Zellerhoff C, et al. Proarrhythmic effect of pacemaker stimulation in patients with implanted cardioverter-defibrillators. Circulation. 2003;108:192-197.
78 Wathen MS, Sweeney MO, GeGroot PJ, et al. Shock reduction using antitachycardia pacing for spontaneous rapid ventricular tachycardia in patients with coronary artery disease. The PainFREE Rx Investigators. Circulation. 2001;104:796-801.
79 Wathen MS, GeGroot PJ, Sweeney MO, et al. Prospective randomized multicenter trial of empirical antitachycardia pacing versus shocks for spontaneous rapid ventricular tachycardia in patients with implantable cardioverter-defibrillators: pacing fast ventricular tachycardia reduces shock therapies (PainFREE Rx II) Trial results. Circulation. 2004;110:2591-2596.
80 Wilkoff BL, Ousdigian KT, Sterns LD, Wang ZJ, et al. A comparison of empiric to physician-tailored programming of implantable cardioverter-defibrillators: results from the prospective randomized multicenter EMPIRIC trial. J Am Coll Cardiol. 2006;48:330-339.
81 Wilkoff BL, Williamson BD, Stern RS, Moore SL, et al. Strategic programming of detection and therapy parameters in implantable cardioverter-defibrillators reduces shocks in primary prevention patients: results from the PREPARE (Primary Prevention Parameters Evaluation) study. J Am Coll Cardiol. 2008;52:541-550.
82 Reddy VY, Reynolds MR, Neuzil P, Richardson AW, et al. Prophylactic catheter ablation for the prevention of defibrillator therapy. N Engl J Med. 2007;357:2657-2665.
83 Windecker S, Ideker RE, Plumb VJ, et al. The influence of ventricular fibrillation duration on defibrillation efficacy using biphasic waveforms in humans. J Am Coll Cardiol. 1999;33:33-38.
84 Sweeney MO, Natale A, Volosin KJ, et al. Prospective randomized comparison of 50%/50% versus 65%/65% tilt biphasic waveform on defibrillation in humans. Pacing Clin Electrophysiol. 2001;24:60-65.
85 Shepard RK, DeGroot PJ, Pacifico A, et al. Prospective randomized comparison of 65%/65% versus 42%/42% tilt biphasic waveform on defibrillation thresholds in humans. J Interv Card Elecrophysiol. 2003;8:221-225.
86 Usui M, Walcott GP, KenKnight BH, Walker RG, et al. Influence of malpositioned transvenous leads on defibrillation efficacy with and without a subcutaneous array electrode. Pacing Clin Electrophysiol. 1995;18:2008-2016.
87 Baker JH, Epstein AE, Voshage-Stahl L. A prospective, randomized evaluation of a nonthoracotomy implantable cardioverter-defibrillator lead system. Endotak/PRX Investigator Group. Pacing Clin Electrophysiol. 1997;20:72-78.
88 Carpenter CM, Galvin J, Guy M, McGovern BA. Runaway pacemaker in an implantable cardioverter defibrillator. J Cardiovasc Electrophysiol. 1998;9:1008-1011.
89 Hauser R, Hayes DL, Almquist AK, et al. Unexpected ICD pulse generator failure due to electronic circuit damage caused by electrical overstress. Pacing Clin Electrophysiol. 2001;24:1046-1054.
90 Rasmussen MJ, Friedman PA, Hammill SC, Rea RF. Unintentional deactivation of implantable cardioverter-defibrillators in health care settings. Mayo Clin Proc. 2002;77:855-859.
91 Brode SE, Schwartzman D, Callans DJ, et al. ICD-antiarrhythmic drug and ICD-pacemaker interactions. J Cardiovasc Electrophysiol. 1997;8:830-842.
92 Singer I, Guarnieri T, Kupersmith J. Implanted automatic defibrillators: effects of drugs and pacemakers. Pacing Clin Electrophysiol. 1988;11:2250-2262.
93 Rajawat YS, Patel VV, Gerstenfeld EP, et al. Advantages and pitfalls of combining device-based and pharmacologic therapies for the treatment of ventricular arrhythmias: observations from a tertiary referral center. Pacing Clin Electrophysiol. 2004;27:1670-1681.
94 Anderson MH, Paul VE, Jones S, et al. Transtelephonic interrogation of the implantable cardioverter-defibrillator. Pacing Clin Electrophysiol. 1992;15:1144-1150.
95 Joseph GK, Wilkoff BL, Dresing T, et al. Remote interrogation and monitoring of implantable cardioverter-defibrillators. J Interv Card Electrophysiol. 2004;11:161-166.
96 Becker R, Ruf-Richter J, Senges-Becker JC, et al. Patient alert in implantable cardioverter-defibrillators: toy or tool? J Am Coll Cardiol. 2004;44:95-98.
97 Sarter BH, Callans DJ, Gottlieb CD, et al. Implantable defibrillator diagnostic storage capabilities: evolution, current status, and future utilization. Pacing Clin Electrophysiol. 1998;21:1287-1298.
98 Natale A, Sra J, Axtell K, et al. Undetected ventricular fibrillation in transvenous implantable cardioverter-defibrillators: prospective comparison of different leads system-device combinations. Circulation. 1996;93:91-98.
99 Swerdlow CD, Ahern T, Chen PS, et al. Underdetection of ventricular tachycardia by algorithms to enhance specificity in a tiered-therapy cardioverter-defibrillator. J Am Coll Cardiol. 1994;24:416-424.
100 Swerdlow CD, Brown ML, Lurie K, et al. Discrimination of ventricular tachycardia from supraventricular tachycardia by a downloaded wavelet-transform morphology algorithm: a paradigm for development of implantable cardioverter-defibrillator detection algorithms. J Cardiovasc Electrophysiol. 2002;13:432-441.
101 Theuns DA, Rivero-Ayerza M, Goedhart DM, et al. Evaluation of morphology discrimination for ventricular tachycardia diagnosis in implantable cardioverter-defibrillators. Heart Rhythm. 2006;3:1332-1338.
102 Duru F, Bauersfeld U, Rahn-Schonbeck M, Candinas R. Morphology discriminator feature for enhanced ventricular tachycardia discrimination in implantable cardioverter defibrillators. Pacing Clin Electrophysiol. 2000;23:1365-1374.
103 Unterberg C, Stevens J, Vollmann D, et al. Long-term clinical experience with the EGM width detection criterion for differentiation of supraventricular and ventricular tachycardia in patients with implantable cardioverter defibrillators. Pacing Clin Electrophysiol. 2000;23:1611-1617.
104 Shivkumar K, Feliciano Z, Boyle NG, Wiener I. Intradevice interaction in a dual-chamber implantable cardioverter-defibrillator preventing ventricular tachycardia detection. J Cardiovasc Electrophysiol. 2000;11:1285-1288.
105 Glickson M, Beeman AL, Luria DM, et al. Impaired detection of ventricular tachyarrhymias by a rate-smoothing algorithm in dual-chamber implantable-defibrillators: intradevice interactions. J Cardiovasc Electrophysiol. 2002;13:312-318.
106 Wood MA, Ellenbogen KA, Shepard RK, Clemo HS. Atrioventricular sequential pacing by an implantable cardioverter-defibrillator during sustained ventricular tachycardia: what is the mechanism? J Cardiovasc Electrophysiol. 2002;13:1309-1310.
107 Grönefeld GC, Israel CW, Padmanabhan V, et al. Ventricular rate stabilization for the prevention of pause dependent ventricular tachyarrhythmias: results from a prospective study in 309 ICD recipients. Pacing Clin Electrophysiol. 2002;25:1708-1714.
108 Chin MC, Rosenqvist M, Lee MA, et al. The effect of radiofrequency catheter ablation on permanent pacemakers: an experimental study. Pacing Clin Electrophysiol. 1990;13:23-29.
109 Martin ET, Coman JA, Shellock FG, et al. Magnetic resonance imaging and cardiac pacemaker safety at 1.5 tesla. J Am Coll Cardiol. 2004;43:1315-1324.
110 Cossú SF, Hsia HH, Simson MB, et al. Inappropriate pauses during bradycardia pacing in a third-generation implantable cardioverter-defibrillator. Pacing Clin Electrophysiol. 1997;20:2271-2274.
111 Sticherling C, Klingenheben T, Hohnloser SH. An unusual cause of ICD shock. Pacing Clin Electrophysiol. 1997;20:2265-2267.
112 Hsu SS, Mohib S, Schroeder A, Deger FT. T wave oversensing in implantable cardioverter-defibrillators. J Interv Card Electrophysiol. 2004;11:67-72.
113 Mann DE, Damle RS, Kelly PA, et al. Comparison of oversensing during bradycardia pacing in two types of implantable cardioverter-defibrillator systems. Am Heart J. 1998;136:658-663.
114 Nazarian S, Roguin A, Zviman MM, et al. Clinical utility and safety of a protocol for noncardiac and cardiac magnetic resonance imaging of patients with permanent pacemakers and implantable cardioverter-defibrillators at 1.5 tesla. Circulation. 2006;114:1277-1284.
115 Gimbel JR. Magnetic resonance imaging of implantable cardiac rhythm devices at 3.0 tesla. Pacing Clin Electrophysiol. 2008;31:795-801.
116 Naehle CP, Strach K, Thomas D, et al. Magnetic resonance imaging at 1.5-T in patients with implantable cardioverter-defibrillators. J Am Coll Cardiol. 2009;54:549-555.
117 Levine GN, et al. Safety of magnetic resonance imaging in patients with cardiovascular devices: an American Heart Association scientific statement from the Committee on Diagnostic and Interventional Cardiac Catheterization, Council on Clinical Cardiology, and Council on Cardiovascular Radiology and Intervention. Endorsed by the American College of Cardiology Foundation, North American Society for Cardiac Imaging, and Society for Cardiovascular Magnetic Resonance. Circulation. 2007;116:2878-2891.
118 Faris OP, Shein M. Food and Drug Administration perspective: magnetic resonance imaging of pacemaker and implantable cardioverter-defibrillator patients. Circulation. 2006;114:1232-1233.
119 Nazarian S, Halperin HR. How to perform magnetic resonance imaging on patients with implantable cardiac arrhythmia devices. Heart Rhythm. 2009;6:138-143.
120 Anfinsen OG, Berntsen RF, Aass H, et al. Implantable cardioverter-defibrillator dysfunction during and after magnetic resonance imaging. Pacing Clin Electrophysiol. 2002;25:1400-1402.
121 Vassolas G, Roth RA, Venditti FJ. Effect of extracorporeal shock wave lithotripsy on implantable cardioverter-defibrillator. Pacing Clin Electrophysiol. 1993;16:1245-1248.
122 Sears SF, Conti JB. Quality of life and psychological functioning of ICD patients. Heart. 2002;87:488-493.
123 Schron EB, Exner DV, Yao Q, et al. Quality of life in the Antiarrhythmics versus Implantable Defibrillators Trial: impact of therapy and influence of adverse symptoms and defibrillator shocks. The AVID Investigators. Circulation. 2002;105:589-594.