CHAPTER 13 Treatment of Leg Telangiectasias with Laser and High-Intensity Pulsed Light
With proper use, lasers are very safe and have not been associated with long-term side effects. Most of the laser radiation used in medicine is within or near the range of visible light in the electromagnetic spectrum. Therefore, its radiant energy level is at a much longer wavelength than that of the high-energy ionizing radiation associated with X-rays and radiation therapy; as such, it is not associated with commonly perceived radiation hazards.1 In addition to determining the specificity of absorption, each laser’s emitted wavelength also dictates the depth of its penetration (Fig. 13.1). (A more thorough discussion of laser physics can be found in other sources.1,2)
Lasers have been used to treat leg telangiectasias for various reasons.3 First, lasers have a futuristic appeal. By virtue of their advanced technology, lasers are perceived as ‘state-of-the-art.’ The general public often equates ‘high tech’ with treatment safety and superiority. Unfortunately, as described later, this perception by both the general public and the physician has often resulted in unanticipated adverse sequelae (scarring and pain) and higher costs; lasers cost considerably more to purchase and maintain than a needle, syringe, and sclerosing solution.
In addition, lasers have theoretical advantages compared with sclerotherapy for treating leg telangiectasias. Sclerotherapy-induced pigmentation is caused by hemosiderin deposition through extravasated red blood cells (RBCs) (see Chapter 8). Laser coagulation of vessels should not have this effect. In the rabbit ear model, approximately 50% of vessels treated with an effective concentration of sclerosing solution demonstrated extravasated erythrocytes, compared with a 30% incidence when treated with the flashlamp-pumped pulsed dye laser (PDL) (Goldman MP, unpublished observations). Furthermore, telangiectatic matting (TM), which occurs in a significant percentage of sclerotherapy-treated patients, has also not been seen after laser treatment of vascular lesions. Finally, specific allergenic effects of sclerosing solutions are not a concern when treating telangiectasias with a laser.
Optical properties of blood are mainly determined by the absorption and scattering coefficients of its various oxyhemoglobin components. Oxyhemoglobin has three major absorption peaks at 418, 542, and 577 nm. A less selective and broader absorption peak spans from approximately 750 to 1100 nm. Figure 13.2 shows the oxyhemoglobin absorption and scattering coefficient depth of penetration into blood.4 The main feature to note in the curve is the strong absorption at wavelengths below 600 nm with less absorption at longer wavelengths. However, a vessel 1 mm in diameter absorbs more than 67% of light even at wavelengths longer than 600 nm. This absorption is even more significant for blood vessels 2 mm in diameter. Therefore, use of a light source above 600 nm would result in deeper penetration of thermal energy without negating absorption by oxyhemoglobin in vessels greater than 1 mm in diameter. This is because the absorption coefficient in blood is higher than that of surrounding tissue for wavelengths between 600 and 1000 nm (Figs 13.2 and 13.3). Shorter wavelengths heat only the portion of the vessel wall closest to the skin surface, which can result in incomplete thrombosis.5 The only caveat is that wavelengths greater than 900 nm are less specific and also target water, making higher fluences required to produce desired effects on oxyhemoglobin, the desired chromophore.6 However, these higher fluences can cause unnecessary damage to surrounding tissue unless adequate cooling measures are employed.
Patients seek treatment for a leg vein largely for cosmetic reasons.7 Bernstein8 has evaluated the clinical characteristics of 500 consecutive patients presenting for laser removal of lower extremity spider veins. Patients ranged in age from 20 to 70 years and had had noticeable spider veins for an average of 14 years. Twenty-eight percent of patients had leg veins less than 0.5 mm in diameter; 39% of patients had veins less than 1.5 mm in diameter. Fifty-six percent of patients who had had sclerotherapy (not stated how this was performed) developed TM. Any treatment that is effective should be relatively free of adverse sequelae. With recent advances, lasers or IPL have become methods for treating telangiectatic vessels with a minimum of adverse effects. However, for these advanced treatments to be effective and safe, they must be used appropriately.
As detailed in Chapter 8, sclerotherapy has a number of potential adverse effects. Up to 30% of patients treated with sclerotherapy develop postsclerosis pigmentation9 and/or TM.10 As discussed above, at least one study determined that TM developed in 56% of patients who presented for laser treatment of leg veins.8 These adverse effects can occur even with optimal treatment but are more common when an excessive inflammatory reaction occurs. To minimize risks of an inflammatory response, lasers and IPL act by producing thermal damage with the ultimate goal being vaporization of the targeted vessel. When used with appropriate fluences, pulse durations, and epidermal cooling, the thermal effects of lasers and IPL present minimal inflammatory response compared with chemical irritation of the vessel wall through sclerotherapy.
An understanding of the appropriate target vessel for each laser and/or IPL is important so that treatment is tailored to the appropriate target. As detailed elsewhere in this book, most telangiectasias arise from reticular veins. Therefore, the single most important concept for the treating physician is that feeding reticular veins must be treated completely before treating the telangiectasia. This minimizes adverse sequelae and enhances therapeutic results. When no apparent connection exists between deep collecting or reticular vessels, telangiectasia may arise from a terminal arteriole or arteriovenous anastomosis.11 In this latter scenario, the telangiectasia may be treated without consideration of underlying forces of hydrostatic pressure. Failure to treat ‘feeding’ reticular veins and short follow-up periods after the use of lasers may give inflated values to the success of laser treatment.12 This chapter reviews and evaluates the use of these nonspecific and specific laser and light systems in the treatment of leg venules and telangiectasias (Table 13.1).
Histology of Leg Telangiectasia
Venules in the upper and middle dermis typically maintain a horizontal orientation. The diameter of the postcapillary venule ranges from 12 to 35 µm.13 Collecting venules range from 40 to 60 µm in the upper and middle dermis and enlarge to 100 to 400 µm in diameter in the deeper tissues. Histologic examination of simple telangiectasia demonstrates dilated blood channels in a normal dermal stroma with a single endothelial cell lining, limited muscularis, and adventitia.14,15 Most leg telangiectasias measure from 26 to 225 µm in diameter.14 Electron microscopic examination of ‘sunburst’ varicosities of the leg has demonstrated that these vessels are widened cutaneous veins.14 They are found 175 to 382 µm below the stratum granulosum. The thickened vessel walls are composed of endothelial cells covered with collagen and muscle fibers. Elastic fibers are also present.
Alternatively, arteriovenous anastomoses may be involved in the pathogenesis of telangiectasia. They have been demonstrated in 1 of 26 biopsy specimens of leg telangiectasias.11 Red telangiectasias have been found to have an oxygen saturation of 76%, compared with blue telangiectasias, which have an oxygen concentration of 69%.16 Thus each type of telangiectasia may have a slightly different optimal absorption wavelength based on its color in addition to its relative size and depth.
Laser Treatment of Leg Telangiectasia
The optimal light source would have a wavelength specific for the vessel treated and would be able to penetrate to the depth of the vessel through its entire diameter. This wavelength has been proposed to be between 600 and 900 nm. Ideally, a light source should have a pulse duration that would allow the light energy to build up in the target vessel so that its entire diameter is thermocoagulated. Optimal pulse durations have been calculated for various diameter blood vessels (Table 13.2).
Diameter of vessel (mm) | Thermal Relaxation Time (sec) |
---|---|
0.1 | 0.01 |
0.2 | 0.04 |
0.4 | 0.16 |
0.8 | 0.6 |
2.0 | 4.0 |
Presented by R.A. Anderson at the Annual Meeting of the North American Society of Phlebology, Washington, DC, November, 1996.
During the process of delivering a sufficient packet of energy to thermocoagulate the target vessel, the overlying epidermis and perivascular tissue should be unharmed. This requires some form of epidermal cooling. A number of different laser and IPL systems have been developed toward this end, as discussed in subsequent sections. In addition to the information presented in the following sections, the reader is encouraged to refer to an excellent summary of various laser treatments for leg veins by Kunishige et al.6
Carbon dioxide laser
The carbon dioxide (CO2) laser has been used for obliterating venules and telangiectatic vessels.17–21 One recent case report described the successful treatment of matted telangiectasias with fractional photothermolysis.22 The patient underwent five successive monthly treatments with the 1550 Fraxel SR laser (Solta Medical, Hayward, Calif.), at energies ranging from 10 to 12 mJ, to an area on her medial thigh. At 6 months after the final treatment session, the target areas showed more than 75% improvement. However, since the natural history of matted telangiectasias is usually to spontaneously resolve over the course of a year, it is uncertain how much of the improvement seen in the aforementioned case report was actually due to ‘the tincture of time’ as opposed to the CO2 laser treatment.
The rationale for using the CO2 laser in the treatment of telangiectasia is to produce ‘precise’ vaporization without significant damage to tissue structures adjacent to the penetrating laser beam. Also, since the CO2 laser does not specifically target melanin, it can be used on those of darker skin types, a subgroup of the population that cannot be safely treated with the pulse dye laser. However, with the CO2 laser, the epidermis and the dermis overlying the blood vessel are destroyed. CO2 laser disruption of vessels has also been reported to cause occasional brisk bleeding from the vessel, which required pressure bandages for 48 hours.20 Pain during treatment is moderate to severe, but of short duration. Most reported studies demonstrate unsatisfactory cosmetic results. Treated areas show multiple hypopigmented punctate scars with either minimum resolution of the treated vessel or neovascularization adjacent to the treatment site (Fig. 13.4). Because of this nonselective action, the CO2 laser is of no advantage over the electrodesiccation needle and has not been used successfully in treating leg telangiectasia.
Argon laser
The argon laser with output at 488 nm and 511 nm has wavelengths somewhat preferentially absorbed by hemoglobin and to a lesser, although significant, extent by water and melanin (Fig. 13.5). Its relatively short wavelength, combined with a spot size of 1 mm, prevents its penetration much beyond 0.5 mm. When the patient is pigmented or tanned, epidermal melanin will selectively absorb the laser energy, preventing penetration below the epidermis. Thus, the argon laser does not have ideal parameters for treating leg veins (Fig. 13.6).
Being continuous in nature, argon lasers do not allow for selective vessel heating, so scarring commonly occurs.23 Specifically, argon laser treatment of telangiectasia or superficial varicosities of the lower extremities may cause purple or depressed scars. In a report of 38 patients treated by Apfelberg et al,18 49% had either poor or no results from treatment, and only 16% had excellent or good results. In addition, almost half of the patients had hemosiderin bruising. In another series, Dixon et al24 noted significant improvement in only 49% of patients. They speculated that after initial improvement, incomplete thrombosis, recanalization, or new vein formation produced reappearance of the vessels after 6 to 12 months.
In an effort to enhance therapeutic success with leg vein sclerotherapy, the argon laser has been used to interrupt the telangiectasia every 2 to 3 cm before injection of a sclerosing agent.25 Eleven of 16 patients completed treatment. Two patients developed punctate depigmented scars, and three patients developed hyperpigmentation. However, 93.7% of patients were reported to have ‘satisfactory’ results.
Cooling the skin simultaneously with argon or tunable dye (577 nm, 585 nm) laser treatment has been demonstrated to produce improvement in 67% of leg telangiectasia 1 mm in diameter.26 This may be caused by temperature-related vasomotor changes in blood flow.27
Contact probe delivery
Directing the laser energy to the target vessel produces another method for more selective delivery of nonspecific laser energy. Keller28 reported on the use of a microcontact argon laser probe to treat ‘spray telangiectasias’ of the leg. Fifteen patients were treated with this device using an argon laser energy of 1 to 2 W with a pulse duration of 0.1 second. This treatment was performed as ‘spot welding’ along the course of the blood vessel; 100% effectiveness and no notable complications were reported. We have not achieved the same success rate as Keller,29 and further reports of this novel form of therapy have not occurred. Thus, at present, argon laser therapy apparently is a satisfactory method for treating selected facial telangiectasia but is much less effective in treating leg telangiectasia. Box 13.1 summarizes the disadvantages of the argon laser treatment.
Krypton triphosphate and frequency-doubled Nd:YAG (532 nm)
Modulated krypton triphosphate (KTP) lasers have been reported to be effective at removing leg telangiectasia, using pulse durations between 1 and 50 ms (see Table 13.1). The 532-nm wavelength is one of the hemoglobin absorption peaks (see Fig. 13.5). Although this wavelength does not penetrate deeply into the dermis (about 0.75 mm), relatively specific damage (compared with the argon laser) can occur in the vascular target by selection of an optimal pulse duration, enlargement of the spot size, and addition of epidermal cooling.
Effective results have been achieved by tracing vessels with a 1-mm projected spot. Typically, the laser is moved between adjacent 1-mm spots with vessels traced at 5 to 10 mm/second. Immediately after laser exposure, the epidermis is blanched. Lengthening of the pulse duration to match the diameter of the vessel is attempted to optimize treatment (see Table 13.2). With this method, West and Alster30 utilized this method to treat 12 patients with leg telangiectasia. They used a 10-ms pulse at 15 J/cm2 with a 1-mm handpiece at a repetition rate of two pulses per second. The average improvement was 25% to 50% (Fig. 13.7).
Quintana et al31 treated 19 leg veins that were less than 1.5 mm in diameter with the Laserscope KTP Dermastat (Laserscope, San Jose, Calif.). They used a 2-mm diameter handpiece at a fluence of 13 to 15 J/cm2 given at a rate of 10 to 15 milliseconds. Patients were treated at 4- to 6-week intervals on four separate visits, with results examined 6 months after the last visit. Of the 28% of patients evaluated, 15% percent achieved 100% clearance, 40% had 75% clearance, 35% had 50% clearance, and 10% had 25% clearance. No scarring was reported, and 25% had transient hyperpigmentation. Therefore, this laser is somewhat effective but requires multiple treatments.
We and others have found the long-pulse 532-nm laser (frequency-doubled Nd:YAG) (VersaPulse, Lumenis, Palo Alto, Calif.) to be effective in treating leg veins less than 1 mm in diameter that are not directly connected to a feeding reticular vein.32 When used with a 4°C chilled tip, a fluence of 12 to 15 J/cm2 is delivered as a train of pulses in a 3- to 4-mm diameter spot size in order to trace the vessel until spasm or thrombosis occurs. Some overlying epidermal scabbing is noted with hypopigmentation not uncommonly occurring in dark-skinned patients. Individual physicians report considerable variation in results. Usually, more than one treatment is necessary for maximum vessel improvement, with only rare reports of 100% resolution of the leg vein (Fig. 13.8). A summary of important clinical evaluations of this technology follows.
McMeekin33 treated 18 sites of leg veins ranging from 0.5 to 1.1 mm in diameter in 10 patients, using a VersaPulse with a 3-mm diameter spot, 5.5°C cooling, at 12 and 16 J/cm2 with one to three passes over each vessel at 2 Hz. He followed the patients for 1 year after a single treatment. Overall, 44% of patients had a greater than 50% clearance from a single treatment. Six percent of patients had complete clearance, 88% had partial clearance, and 6% had no change. Of the partial clearance group of patients, more cleared at 16 J/cm2 than at 12 J/cm2. At 16 J/cm2, 37% cleared 25% to 50%, 25% cleared 50% to 75%, and 37% cleared 75% to 100%. Ninety-four percent developed hyperpigmentation that took up to 6 months to resolve. One patient developed blisters and hypopigmented atrophic scars.
Bernstein et al34 achieved similar efficacy in a study of 15 women with leg telangiectasia less than 0.75 mm in diameter at 27 sites, using a 10-ms pulse duration 532-nm laser at 16 J/cm2 with a 3-mm diameter spot size at 4 Hz and using a chill tip with three passes over each vein twice, 6 weeks apart. Computer-based image analysis demonstrated more than 75% clearing. Ten of the 27 sites (37%) cleared completely after one treatment. Two of 15 patients (13.3%) reported blistering with minimal hyperpigmentation noted 6 weeks after treatment.
A longer pulse duration of 20 to 50 ms, accompanied by an increase in spot size to 5 mm with a higher total fluence of 20 J/cm2 became available, with improvement in efficacy and a reduction in pigment changes. Narukar35 evaluated patients with leg veins less than 1.5 mm in diameter, treated with 2- to 50-ms pulses up to 40 J/cm2 with a 3- to 6-mm diameter spot size and a 4°C chill tip. He treated patients at 6- to 8-week intervals two to three times. A 45% clearance was found. Interestingly, a 68% clearance was found in patients whose previous sclerotherapy showed a good response. A 32% clearance occurred in patients whose vessels responded poorly to sclerotherapy. At 2-month follow-up, 2% of patients had TM, 4% had hyperpigmentation, and 2% had hypopigmentation.
Massey and Katz36 bettered Narukar’s results using a spot size of 5 mm, a pulse width of 50 ms, fluences of 18 to 20 J/cm2, and a 1.5-Hz repetition rate. A 75%–100% reduction was achieved in 68% of vessels less than 1 mm and in 44% of vessels 1 to 2 mm after two treatments. These results were obtained with multiple passes to achieve vessel clearance. Hypopigmentation and mild hyperpigmentation, lasting 6 to 8 weeks, were noted in 20% of patients. No scarring was observed. Krause37 claimed similar results using a 2-mm diameter spot size. A 2-mm diameter spot size is claimed to produce a narrower band of either hypo- or hyperpigmentation.
A 532-nm KTP laser was also evaluated in a multipulse mode emission (three stacked pulses of 100 ms, 30 ms, 30 ms, and a delay between pulses of 250 ms), a fluence of 60 J/cm2, and a 0.75-mm collimated spot without cooling (Virdis Derma, Quantel Medical, France).38 On average, with one treatment, 53% of leg veins 0.5 to 1 mm in diameter were cleared, 78% with two treatments, 85% after three treatments, and 93% 6 weeks after a fourth treatment, all 6 weeks apart. It is suggested that the multipulse mode increases intravascular heating in a manner similar to the multipulse mode of IPL (see below) while allowing cooling of the epidermis. Hypopigmentation lasting ‘a few months’ was observed in 18% of patients and TM occurred in 7% of patients.
In a study by Woo et al,39 a 532-nm Nd:YAG at 20 J/cm2 delivered as a 50-ms pulse through a contact cooling and 5-mm diameter spot was compared with a 595-nm PDL at 25 J/cm2 with a pulse duration of 40 ms, cryogen spray cooling, and a 3- × 10-mm elliptical spot. After one treatment with the 532-nm Nd:YAG there was 50% to 75% improvement in 2 of 10 patients and more than 75% improvement in 3 of 10 patients. There was better improvement in the PDL-treated patients, with 6 of 10 having 50% to 75% improvement.
In another study, a 532-nm diode laser with a 1-mm diameter spot at fluences of 2 to 32 J/cm2 was compared with a 1064-nm Nd:YAG laser at 1- to 20-ms pulses through a 3-mm diameter spot at 130 to 160 J/cm2 in the treatment of TM vessels less than 0.3 mm in diameter that did not respond to sclerotherapy.40 Two to three passes were needed to close the vessels with each laser. Overall, 39% of vessels treated with the 532-nm laser and 55% of those treated with the 1064-nm laser had better than 50% lightening.
Copper bromide 578 nm
Forty-six women with red leg telangiectasias less than 1.5 mm in diameter were treated with 1 minute of precooling to the skin followed by laser pulses at 50 to 55 J/cm2 through a 1.5-mm diameter spot size generated with a 300-ms pulse, with a 75-ms delay between pulses.41 Up to three treatments were given at 6-week intervals. An average of 1.7 treatments produced greater than 75% improvement in 72% of patients. Treatments were given through a circulating cooling window at 1 to 4°C. Problems with this new technology are the long warm-up time of the laser (15–20 minutes) and the long time required to treat the vessels with a 1.5-mm diameter spot size.
Flashlamp-pulsed dye laser, 585 or 595 nm
The PDL has been demonstrated to be highly effective in treating cutaneous vascular lesions consisting of very small vessels, including PWSs, hemangiomas, and facial telangiectasia.42 The depth of vascular damage is estimated to be 1.5 mm at 585 nm and 15 to 20 µm deeper at 595 nm. Therefore, penetration to the typical depth of superficial leg telangiectasia may be achieved.43 However, telangiectasia over the lower extremities has not responded as well, with less lightening and more post-therapy hyperpigmentation.44 This may be due to the larger diameter of leg telangiectasia as compared with dermal vessels in PWS and larger diameter feeding reticular veins, as described previously.
Vessels that should respond optimally to PDL treatment are predicted to be red telangiectasia less than 0.2 mm in diameter, particularly those vessels arising as post-sclerotherapy TM. This is based on the time of thermocoagulation produced by this relatively short pulse laser system (see Table 13.2). The PDL produces vascular injury in a histologic pattern that is different than that produced by sclerotherapy. In the rabbit ear vein, approximately 50% of vessels treated with an effective concentration of sclerosant demonstrated extravasated RBCs, whereas with PDL treatment, extravasated RBCs were apparent in only 30% of vessels treated (unpublished observations). Thus, the PDL may produce less post-therapy pigmentation because of a decreased incidence of extravasated RBCs (Figs 13.9–13.13).
The etiology of TM is unknown but has been thought to be related either to angiogenesis45 or to a dilatation of existing subclinical blood vessels by promoting collateral flow through arteriovenous anastomoses.46 One or both of these mechanisms may occur. Obstruction of outflow from a vessel (which is the end result of successful sclerotherapy) is one of the most important factors contributing to angiogenesis.47 In addition, endothelial damage leads to the release of histamine and other mast cell factors and vasokines, which promote both the dilatation of existing blood vessels and angiogenesis.48,49 Sclerotherapy by its mechanism of endothelial destruction provides the means for new blood vessel formation to occur. Indeed, it is remarkable that physicians do not see a higher incidence of post-treatment TM associated with sclerotherapy.
TM has not been reported to be a side effect of argon, PDL, or other laser treatment of any other vascular disorders. This may be due to the production of intravascular fibrin that occurs during laser treatment.50–52 Fibrin develops through thermal alteration of fibrin complexes or proteolytic cleavage of fibrinogen. Fibrin deposition has been demonstrated to promote angiogenesis.48 Sclerotherapy-induced vascular injury has not been associated with the appearance of fibrin strands (unpublished observations). This is explained by limitation of angiogenesis by factors other than those associated with the absence of fibrin deposition, or by intravascular consumption of fibrin-promoting factors in laser treatment of cutaneous vascular disease.
Another possible mechanism for absence of TM in laser-treated blood vessels is a decrease in perivascular inflammation. Rabbit ear vein treatment with the PDL relatively decreases perivascular inflammation compared with vessels treated with sclerotherapy alone.52 Multiple factors associated with inflammation have been demonstrated to promote both a dilatation of existing blood vessels and angiogenesis.49
Polla et al44 treated 35 superficial leg telangiectasias with the PDL. The exact laser parameters were not given, except that vessels were treated an average of 2.1 times with a maximum of four separate treatments. These vessels were described as being either red–purple and raised, or blue and flat. No mention was made regarding the association of reticular or varicose veins or vessel diameter. Fifteen percent of treated vessels had greater than 75% clearing, with 73% of treated areas showing little response to treatment. The only lesions that responded at all were red–pink tiny telangiectasia. Almost 50% of the treated patients developed persistent hypopigmentation or hyperpigmentation.
Goldman and Fitzpatrick53 treated 30 female patients with red leg telangiectasias of less than 0.2 mm in diameter. Thirteen of 101 telangiectatic patches were noted to have an associated reticular ‘feeding’ vein between 2 and 3 mm in diameter that was not treated. Seven patients with 25 patches of TM after previous sclerotherapy were also treated.
As hypothesized, TM and persistent pigmentation did not occur with PDL treatment of leg telangiectasia. Post-PDL hyperpigmentation completely resolved within 4 months. There were no episodes of cutaneous ulceration, thrombophlebitis, or other complications. However, hypopigmentation occurred in some patients with tanned skin (Fig. 13.14). The laser impact sites usually remained hypopigmented for years and in many cases were thought to be permanent. With PDL treatment, the most effective fluence appears to be between 7.0 and 8.0 J/cm2. With these parameters, approximately 67% of telangiectatic patches completely faded within 4 months (Figs 13.15–13.18).
There appears to be no difference in the response to PDL treatment between linear leg telangiectasias and TM vessels. In the seven patients with 25 sites treated (mentioned earlier in this section), 72% of the treated sites completely faded at laser fluences between 6.5 and 7.5 J/cm2. Matted vessels did not respond to treatment in only one patient with four areas of TM. Less than 100% resolution occurred in 16% of treated areas (Fig. 13.19).
Like TM vessels, essential telangiectasia represents a network of fine red telangiectasia usually less than 0.2 mm in diameter. This condition responds well to the PDL at fluences of 7 to 7.25 J/cm2.54 Treatment, however, is tedious, with more than 2000 5-mm diameter pulses sometimes necessary to cover the entire affected area.
The reason for greater efficacy of treatment in Goldman and Fitzpatrick’s report in comparison with others44,55 may be due to the rigid criteria by which patients were selected for treatment. Patients who responded well to treatment had red telangiectasia less than 0.2 mm in diameter without associated ‘feeding’ reticular veins.
Many physicians have found that vessel location may affect treatment outcome, with vessels on the medial thigh being the most difficult to completely eradicate. However, with the PDL, vessel location appears to be unrelated to treatment outcome if telangiectatic patches with untreated associated reticular veins are excluded. In addition, there appears to be no obvious difference in efficacy between telangiectatic patches that are treated with compression and those that are not (Fig. 13.20). Sadick et al56 conducted a study which further supported the notion that graduated compression stocking use for 7 days starting immediately after treatment of class I-II venulectasia with PDL yielded no additional therapeutic efficacy.
Long-pulse flashlamp-pumped pulsed dye laser
In an effort to thermocoagulate larger diameter blood vessels, a second generation of PDLs with a longer pulse duration, lengthened to 1.5 to 40 ms, and longer wavelength, increased to 595 to 600 nm, was released in 19966 (see Table 13.1). This theoretically permits more thorough heating of a larger vessel at a greater depth. Specifically, this new-generation PDL is able to treat vessels 1 mm in width and 1 mm in depth.57One study using a 595-nm PDL at 1.5 ms found more than 50% clearance of leg veins at a fluence of 15 J/cm2 and approximately 65% clearance using a fluence of 18 J/cm2.58 In this limited study of 18 patients, vessels ranging in diameter from 0.6 to 1 mm were treated with an elliptical spot size of 2 mm × 7 mm through a transparent hydrogel-based wound dressing. No adverse sequelae were noted at the 5-month follow-up visit (Fig. 13.21).
Another study evaluated the use of the 595-nm long-pulse PDL on 35 sites of lower extremity spider veins in 15 subjects.59 This new laser utilized 8 pulselets spread over the selected pulse duration, up to 40 ms. Treatments were administered three times, at 6-week intervals, using a 3- × 10-mm spot size, an average fluence of 20.4 J/cm2, and a dynamic cooling device. At 8 weeks following the final treatment, clearance rates ranged from 65% to 75% when measured by the treating physician, and approximately 40% to 50% when assessed by blinded observers. Thus, results were strengthened by the fact that not only did the treating physician determine subject improvement scores but also an additional three separate physicians, who were blinded as to which photographs were pre- or post-treatment, assessed improvement in each case as well. Of note, one subject developed severe post-treatment hyperpigmentation, which persisted long enough to delay subsequent treatment by 6 weeks. At 8 weeks following the final treatment, 9 of 28 sites receiving three treatments showed residual hyperpigmentation as assessed by the treating physician; blinded observers using digital photographic assessment noted a 25% incidence of hyperpigmenation at this same time point. From our experience, it is highly likely that this hyperpigmentation would continue to resolve completely over the ensuing weeks to months.
Lee and Lask60 treated 25 women with leg telangiectasias less than 1 mm in diameter with the long-pulse PDL (LPDL) (Sclerolaser, Candela Corp., Wayland, Mass.). Each patient had four areas treated; two at a wavelength of 595 nm with fluences of 15 or 20 J/cm2, with two additional areas treated with a 600-nm wavelength at 15 or 20 J/cm2, respectively. A maximum of three treatments were performed at 6-week intervals. All patients had improvement. The 595-nm wavelength at 20 J/cm2 gave the best results. Treatment response was variable and unpredictable, with some patients having complete resolution and some having only slight improvement. Three patients had superficial scabbing that resolved without apparent scarring. Most patients experienced purpura and hyperpigmentation that resolved after several weeks. Reasons for the variable efficacy were not reported.
West and Alster30 treated 12 patients with leg telangiectasia with a 590- or 595-nm pulse at 15 J/cm2. An average improvement of 75% occurred in their patients. Hyperpigmentation persisted at the 12-week follow-up period in 71% of patients.
Bernstein et al61 demonstrated similar results in their study of 10 women with skin type I and II and having leg telangiectasias less than 1.5 mm in diameter treated with a 1.5-ms, 595-nm LPDL at fluences of 15 and 20 J/cm2 three times at each site at 6-week intervals. Patients were treated through a hydrogel dressing that resulted in a 9% energy loss. Computer-based image analysis demonstrated at least 50% clearing in 80% of treated areas. Twenty percent of treated sites had hypopigmentation, and 40% had hyperpigmentation 6 weeks after the final treatment.
Reichert57 performed the largest study on the use of the LPDL by evaluating 80 patients with more than 250 treatment sites of telangiectasia not associated with feeding reticular veins. Treatment parameters were a 1.5-ms pulse at 16 to 22 J/cm2 with a 2 mm × 7 mm or a 7-mm diameter spot at a 590-nm wavelength for red telangiectasias than 0.5 mm in diameter and a 595- to 600-nm wavelength for larger vessels. Ice cooling of the skin was performed both before and after treatment. A hydrogel was used during treatment and was cooled to 8°C when fluences exceeded 20 J/cm2. A clearance rate of almost 100% was achieved at the 4- to 6-month follow-up time after one to two treatments in 95% of vessels up to 0.5 mm in diameter. Eighty percent of telangiectasias 0.5 to 1 mm in diameter faded in about 80% of sites after four treatments. Hyperpigmentation was present for ‘months’ in 40% of treated sites, with 10% having hypopigmentation. ‘Frequent epidermal sloughing followed by crusting and gradual re-epithelization over 2 to 3 weeks’ was reported when high fluences were used. Although this study had poor statistical and evaluation analysis, it does indicate that with optimal technique, specific types of leg telangiectasia will respond to the LPDL.
Hohenleutner et al62 treated 87 patients with telangiectasias that were less than 1 mm in diameter with the LPDL using either ice cube or gel cooling. They did not treat feeding reticular veins when they were of ‘no hemodynamic significance’. Vessels greater than 1 mm in diameter did not respond to treatment parameters and were excluded from study. They found that cooled Vigilon gel decreases fluence by 35% in addition to decreasing skin temperature by 5°C for 1 minute. Ice cube cooling produced a 15°C decrease in skin temperature for 1 minute. With ice cube cooling, greater than 95% clearance occurred in 20% of patients with veins that were less than 0.5 mm in diameter, and no veins between 0.5 and 1 mm in diameter treated at 600 nm with 18 J/cm2 achieved greater than 95% clearing. A 50% to 95% clearance occurred in 82% of veins that were less than 0.5 mm in diameter and in 50% of veins between 0.5 and 1 mm in diameter at a fluence of 20 J/cm2. Hyperpigmentation and/or hypopigmentation occurred in 32% of treated areas and resolved within 6 months. When fluence was increased to 20 J/cm2, hyperpigmentation occurred in 48% of treated areas. Thus, cooling with ice cubes enhances clinical efficacy of this laser; multiple treatments may enhance efficacy even further. This has led to the development of the dynamic cooling LPDL discussed below.
Twenty-seven women with leg telangiectasias that were less than 1 mm in diameter were evaluated in one clinical study. Each patient had three areas treated. There was no difference in vessel response between a 4-ms 16-J/cm2 pulse, a 4-ms 20-J/cm2 pulse, and a 1.5-ms 14- or 16-J/cm2 pulse. Little or no improvement was seen in 50% and 33% of patients, respectively, after one treatment. Hyperpigmentation lasting about 12 weeks was seen in 40% to 67% of treated veins. Hypopigmentation lasting about 12 weeks occurred in 20% to 27% of veins.63 It is unclear why this specific study proved much less effective than previous studies on similar telangiectasia. The authors of this study were particularly objective in their treatment evaluation, with subtle improvements being less noticeable in photographic analysis by independent evaluators.
Polla64 evaluated the Candela LPDL on 40 patients with leg veins 0.05 to 1.5 mm in diameter. He used a 6- or 20-ms pulse with a 7- or 10-mm diameter spot at 10 to 13 J/cm2 and 6 to 7 J/cm2, respectively, with a dynamic cooling device (DCD) setting of 30 ms, 10 ms delay. One to seven treatments were performed at 3-week intervals. Optimal results were obtained after two sessions, with 8% having total clearance and 67% having clearance above 40%. All patients had purpura for 7 to 10 days; 33% had pigmentation for less than 2 months and 15% for over 2 months.
Weiss and Weiss65 had similar results using the Cynosure LPDL on 20 patients with sclerotherapy-resistant TM. They performed a single treatment with a 20-ms pulse and a 7-mm diameter spot at 7 J/cm2 for a total of three stacked pulses with simultaneous cold air cooling. Eighteen of 20 patients had at least 50% improvement at 3 months post-treatment. Purpura occurred in only 25% of patients and lasted 10 days.
A longer pulse duration of 40 ms was used on 10 patients with leg telangiectasia up to 1 mm in diameter at 595 nm with DCD cooling at 25 J/cm2.66 Six patients had 50% to 75% improvement and 2 of 10 had hyperpigmentation which lasted over 3 months.
Finally, a study using the DCD LPDL was performed on 14 Asian patients with 38 leg veins, distinguishing between veins less than 0.2 mm in diameter, 0.2 to 1 mm in diameter, and 1 to 2 mm in diameter.67 Vessels less than 0.2 mm in diameter were treated twice at 8-week intervals with 1.5- or 3-ms pulses through a 3- × 10-mm elliptical spot at 4 to 25 J/cm2. These vessels demonstrated total resolution. Vessels between 0.2 and 1 mm in diameter were treated with a pulse duration of 3 to 10 ms, with 91% having greater than 75% improvement. Vessels between 1 and 2 mm in diameter were treated with a pulse duration of 10 to 20 ms, with 55% of veins having better than 50% clearing. All treatments were performed with DCD. Mild hyperpigmentation was present in nearly 50% of treated areas at 3-month follow-up.
Our experience is similar to that reported above. We utilize the LPDL at pulse durations matching the thermal relaxation time of the leg veins as detailed by Kono et al.67 The energy fluence used is just enough to produce vessel purpura and/or spasm. Like Weiss and Weiss,65 we use stacked pulses to achieve this clinical endpoint. We have used both LPDL systems and find them comparable. Because of the necessity for multiple treatments and the significant occurrence of long-lasting hyperpigmentation, like Weiss and Weiss65 and Kono et al,67 we reserve the use of the LPDL for sclerotherapy-resistant, red telangiectasias that are less than 0.2 mm in diameter.
Long-pulse alexandrite (755 nm)
A long-pulse alexandrite laser was developed to treat hair. It soon became apparent that the wavelength, fluence, and pulse duration could also be used for telangiectasia (see Table 13.1). The 755-nm wavelength should penetrate 2 to 3 mm beneath the epidermis. This laser has been reported to be effective in thermocoagulating blood vessels in clinical and histologic studies.68,69,70 One study of leg telangiectasia in 28 patients treated three times every 4 weeks at fluences ranging from 15 to 30 J/cm2 found that a single-pulse technique with 20 J/cm2, 5-ms pulse duration yielded the best resolution when combined with sclerotherapy using 23.4% hypertonic saline (HS)69 (Fig. 13.22). When this technique was used without epidermal cooling with a chill tip at 4°C, focal crusting and scabbing was noted. With laser treatment alone, telangiectasias smaller than 0.2 mm in diameter improved by 23%, vessels between 0.4 and 1 mm improved by 48%, and telangiectasias of 1 to 3 mm improved by 32%.
Kauvar and Lou71 treated 20 women with 54 patches of leg veins measuring 0.3 to 2 mm in diameter with a single treatment of a 3-ms alexandrite laser at 60 to 80 J/cm2 through an 8-mm diameter spot with dynamic epidermal cooling. Multiple passes were given until vessel clearance (averaging 1.9 passes). At 12-week follow-up, 65% of 51 treated areas showed greater than 75% clearance. Hyperpigmentation was observed in 35% of treated areas.
An additional study using an alexandrite laser at 90 J/cm2 with a 3-ms pulse through a 3- × 10-mm spot size and dynamic cooling with an 80-ms cryogen spray was performed on leg telangiectasia 0.3 to 1.3 mm in diameter.72 The pain of treatment ranged from mild to severe. Almost all treated areas had purpura, edema, and erythema. Most vessels showed clearance of 50% to 75%, with hyperpigmentation in 15 of 20 subjects at 12 weeks. The authors speculated that the high pigmentation rate, three times that of Kauvar and Lou’s study,71 was due to the increased fluence used.
Ross et al73 set out to determine the optimal fluence and pulse width for the treatment of leg telangiectasias with the long pulse 755 nm alexandrite laser. Fifteen patients with leg telangiectasias ranging in diameter from 0.2 to 1.0 mm were treated with pulse durations ranging from 3 to 100 milliseconds. For each pulse duration, test spots were performed to determine optimal radiant exposures which ellicited persistent bluing and/or immediate stenosis as clinical endpoints. The optimal settings for each patient were then used to treat larger areas of similar-sized vessels. Follow-up evaluations were performed 12 weeks after the treatment. Overall, the optimal pulse duration was 60 ms for most patients, with a clearance of approximately 65% after the single treatment session. Furthermore, the average radiant exposure necessary for vessel closure was 89 J/cm2. Using these optimal long pulse alexandrite settings not only achieved satisfactory vessel clearance but also resulted in minimal side effects. A study by Eremia et al,74 comparing the alexandrite laser to the 810-nm diode laser and the 1064-nm Nd:YAG laser in the treatment of leg telangiectasia 0.3 to 3 mm in diameter on 30 women, did not show as promising results as those previously mentioned. These authors found that the 3- × 10-mm spot size was difficult to use and the study was performed with an 8-mm diameter spot with 60 to 70 J/cm2, a 3-ms pulse, and an 80–100-ms cryogen spray after an 80-ms delay. With the alexandrite laser, greater than 75% improvement occurred in only 33% of sites and greater than 50% improvement in 58% of sites after two treatments. Ten of 22 patients developed TM, pain was a significant problem, and almost all patients demonstrated marked post-treatment inflammation for 1 to 2 weeks. The 1064-nm Nd:YAG and 810-nm diode lasers were better tolerated, having little to no adverse effects, with greater than 75% improvement occurring in 88% of the Nd:YAG-treated veins and 29% of the diode-treated veins. The 1064-nm Nd:YAG was used with a 6-mm diameter spot size, 150 J/cm2, with a 25-ms pulse duration for small vessels and a 100-ms pulse for larger vessels, with a 30-ms post-contact cryogen spray.
Diode lasers
Multiple diode-pumped lasers are now available, including a 532-nm, an 800-nm, and an 810-nm (gallium-arsenide) laser (see Table 13.1). Diode lasers generate coherent monochromatic light through excitation of small diodes. These devices are therefore lightweight and portable, with a relatively small desktop footprint. Dierickx et al75 evaluated an 800-nm diode laser (LightSheer, Lumenis, Santa Clara, Calif.) on eight areas of leg veins. The laser was used at 15 to 40 J/cm2 given in 5- to 30-ms pulses as double or triple pulses separated by a delay of 2 seconds. Veins were treated every 4 weeks for three sessions and evaluated 2 months after the last treatment. Optimal parameters were 30-ms pulses at 40 J/cm2. At these parameters, vessels 0.4 to 1 mm in diameter showed 100% clearing in 22%, 75% clearing in 42%, and 50% clearing in 32%. Trelles et al76 evaluated both the subjective as well as the objective efficacy of an 800-nm diode laser for leg vein clearance in 10 women of various ages and skin types. Investigators used a sequence of five to eight stacked pulses with a pulse duration of 50 ms, a delay of 50 ms, and a 3-mm spot size. Treatments were administered at 2 month intervals until complete clearance occurred, and final efficacy was assessed 6 months following each patient’s final treatment. To reach complete clearance, 50% of patients needed three treatment sessions and the remaining 50% needed less than three. Although treated leg veins varied from 1 to 4 mm in diameter, the best results were ultimately seen in those vessels that had initially measured 3 to 4 mm. Treatment of vessels located on the thigh as well as those in patients with Fitzpatrick skin type III also yielded superior efficacy. Of note, no correlation was found between patient age and efficacy of treatment.
Garden et al77 used an 810-nm diode laser with a 750-µm spot size at 40 W and 50-ms pulses for a total of 453 J/cm2 of fluence delivered. Twelve patients with 58 vessels 0.2 to 0.5 mm in diameter were treated with three to four passes until vessel spasm occurred. Patients were retreated every 2 to 4 weeks. There was a mean clearance of 60% after 2.2 treatments. Eighteen vessels had greater than 70% clearance after three treatments. When a scanner was incorporated into the diode laser so that 15- to 20-mm-long passes could be given, efficacy increased. In 11 patients treated with the scanner diode in two sessions 2 to 4 weeks apart, 18% of vessels had 75% to 90% clearance, 21% had 50% to 75% clearance, 18% had 25% to 50% clearance, and 36% had up to 25% clearance.
In another study, 35 patients with spider leg veins were treated with an 810-nm diode laser with a 12-mm diameter spot, 60-ms pulse duration and 80 to 100 J/cm2 with a cooled handpiece.78 Fifteen of the 35 patients had complete disappearance of the spider veins. Six months after the second laser treatment, 12 patients with partial or no response had dropped out of the study and 7 patients had had a relapse in their leg veins, with an additional patient having a relapse at 1-year follow-up. Two of the 35 patients had scarring. EMLA cream was applied to treatment sites 1 hour prior to the procedure to limit perioperative pain.
A 940-nm diode laser has also been used in the treatment of blue leg telangiectasias less than 1 mm in diameter without Doppler evidence of refluxing feeding veins.79 Twenty-six patients were treated with 300 to 350 J/cm2 with a 40 to 70-ms pulse and 1-mm diameter spot, with a clearance of greater than 50% in 20 patients and greater than 75% in 12 patients. Slight textural changes were seen in five patients and pigmentation taking several months to resolve in four patients. No cooling was provided except for ice packs after treatment. In a follow-up of these patients 1 year later, 75% of patients had greater than 75% clearance.80
These outstanding long-term results were not seen in a separate study using the same laser but with a variety of pulse durations (10–100 ms) and fluences (200–1000 J/cm2) through a 0.5-mm diameter spot for vessels less than 0.4 mm in diameter, a 1-mm diameter spot for vessels 0.4 to 0.8 mm in diameter, and a 1.5-mm diameter spot for vessels 0.8 to 1.4 mm in diameter.81 Fluences were adapted to have complete vessel clearance without epidermal blanching. No cooling device was used and patients were evaluated at 1 year. The largest diameter vessels had the highest clearance rates, with only 13% of vessels that were less than 0.4 mm in diameter clearing by more than 75% as opposed to 88% of vessels that were 0.8 to 1.4 mm in diameter. Laser therapy was more painful than sclerotherapy in 31 of 46 patients, with equal efficacy noted by the patients who had had both forms of treatment.
Finally, a combination diode laser with radiofrequency (RF) delivered at levels up to 100 J/cm3 has been used to treat leg telangiectasia. Chess82 treated 25 patients with 35 leg veins 0.3 to 5 mm in diameter with a 915-nm diode laser at fluences ranging between 60 and 80 J/cm2 as well as RF energy at 100 J/cm3 through a 5- × 8-mm elliptical spot size, with 5°C contact cooling, in up to three sessions every 4 to10 weeks. He found 77% of treated sites exhibited greater than 75% improvement at 6 months. The average discomfort rating was 7 out of 10. Three sites on three different patients developed eschar formation without permanent scarring. In another study, leg telangiectasia 1 to 4 mm in diameter was treated with 60 to 80 J/cm2 fluence and 100 J/cm3 RF energy through a 5- × 8-mm elliptical spot size with 5°C contact cooling in three separate sessions at 2- to 4-week intervals.83 Overall, 75% of vessels had greater than 50% improvement and 30% had greater than 75% improvement at 2-month follow-up. Almost no complications were noted to occur. Another study evaluated the efficacy of a combined 900-nm diode laser with a bipolar RF device in the treatment of 1 to 4 mm leg veins in 40 patients with skin types II-IV.84 At the beginning of the study, each patient was examined to ensure the saphenous vein and its collaterals were competent, without perforating or reticular vessels. Treatments were administered at 2 week intervals, for a maximum of three treatments. The diode laser reached an average fluence of 60 J/cm2 and had a 250 ms exposure time. Radiofrequency energy was delivered at 100 J/cm3, with 5°C contact cooling. The majority of patients required one or two treatment sessions. Clinical photography coupled with computer generated data minimized subjectivity in follow-up improvement assessments. During 6-month post-treatment assessments, 82.5% of subjects achieved over a 50% clearance in target vessels. Interestingly, treatments on thicker vessels (>2 mm) and those performed on patients of darker skin types showed the greatest efficacy. However, even in those patients with lighter, Type II, skin, a vessel clearance rate of greater than 50% was seen in 66%. The post-treatment telangiectatic matting that developed in less than 10% of patients had completely or almost completely resolved in each by the 6-month assessment. Overall, very few side effects were noted.
Finally, a more recent study evaluated the dermal histologic and immunohistochemical changes induced by exposure of leg telangiectasias to either a combination 915-nm diode laser and RF device or to a 1064-nm Nd:YAG laser.85 Three patients with 0.1 to 2.0-mm telangiectasias each had one leg treated with the combination diode and RF device, and the opposite leg treated with the Nd:YAG laser. Punch biopsies from treated areas were taken 7 days after laser/RF exposure. Tissue from each treatment type showed intermediate-sized vessels with complete thrombosis and hemorrhage within the dermis as well as the subcutis; focal full-thickeness necrosis was seen in the overlying epidermis. In a single treatment session of both the combination diode and RF device as well as the Nd:YAG, each yielded an average of 50% to 75% clinical clearing. Thus, when comparing results from both treatment modalities, the similar degree of improvement in the clinical appearance of the telangiectasias was supported by histologic examination of tissue specimens.
Fiber-guided laser coagulation
A stronger endoluminal laser has been used to treat larger diameter veins, including the great saphenous vein (GSV). Bone Salat86 treated 44 patients, 38 with incompetent GSVs, with endoluminal laser thermocoagulation. The fiber is inserted through either a phlebectomy or a percutaneous approach into the GSV. The first laser used in this treatment was the 810-nm LaserLite A100 diode laser (Diomed, Andover, Mass.) with energy emission powers of 0.5 to 30 W. Using an optical fiber of 300 to 600 µm in diameter, the practitioner used 5 to 10 W with 3- to 4-second pulses to thermocoagulate the vessel. Vessels ranged from 7 to 22.5 mm in diameter. Treated limbs were then compressed with bandages or graduated compression stockings and evaluated at 1 and 2 weeks and at 3 to 6 months with duplex. No adverse effects were reported. All but one patient had resolution of reflux.
High-Intensity Pulsed Light
The high-intensity pulsed light (IPL) source was developed as an alternative to lasers to maximize efficacy in treating leg veins (PhotoDerm VL, ESC/Sharplan now Lumenis, Santa Clara, Calif.). This device permits sequential rapid pulsing, longer duration pulses, and penetrating longer wavelengths compared with other laser systems (Fig. 13.23).
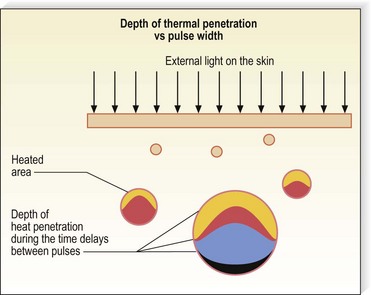
Figure 13.23 Diagram of the effect of repetitive pulses of the PhotoDerm light source on a 2-mm vessel 1 mm below the epidermis.
(Courtesy Shimon Eckhouse, PhD, Energy Systems Corporation, Inc, Newton, Mass.)
Theoretically, a phototherapy device that produces a noncoherent light as a continuous spectrum longer than 550 nm should have multiple advantages over a single-wavelength laser system. First, both oxygenated and deoxygenated hemoglobin absorb at these wavelengths. Second, blood vessels located deeper in the dermis are affected. Third, thermal absorption by the exposed blood vessels should occur with less overlying epidermal absorption, since the longer wavelengths penetrate deeper and are absorbed less by the epidermis, including melanin (Fig. 13.24).
With the theoretical considerations just mentioned, IPL emitting in the 515- to 1000-nm range was used at varying energy fluences (5–90 J/cm2) and various pulse durations (2–25 ms) to treat venectasias 0.4 to 2.0 mm in diameter. This IPL allows treatment through a quartz crystal of 8 mm × 35 mm or 8 mm × 15 mm (up to 2.8 cm2) that can be decreased in size to match the clinical area of treatment. Clinical trials using various parameters with the IPL, including multiple pulses of variable duration, demonstrated efficacy ranging from over 90% to total clearance in vessels less than 0.2 mm in diameter, 80% in vessels 0.2 to 0.5 mm in diameter, and 80% in vessels 0.5 to 1 mm in diameter.87–89 The incidence of adverse sequelae was minimal, with hypopigmentation occurring in 1% to 3% of patients, but resolving within 4 to 6 months. Tanned or darkly pigmented Fitzpatrick type III patients were likely to develop hypopigmentation and hyperpigmentation in addition to blistering and superficial erosions. However, these all cleared over a few months. Treatment parameters found to be most successful for vessels less than 0.2 mm in diameter ranged from a single pulse of 22 J/cm2 in 3 ms to a double pulse of 35 to 40 J/cm2 given in 2.4 and 4.0 ms with a 10-ms delay. Vessels between 0.2 and 0.5 mm were treated with the same double-pulse parameters or with a 3.0- to 6.0-ms pulse at 35 to 45 J/cm2 with a 20-ms delay time. Vessels above 0.5 mm were treated with triple pulses of 3.5, 3.1, and 2.6 ms with pulse delays of 20 ms at a fluence of 50 J/cm2 or with triple pulses of 3, 4, and 6 ms with a pulse delay of 30 ms at a fluence of 55 to 60 J/cm2. The choice of a cut-off filter was based on skin color, with light-skinned patients using a 550-nm filter and darker-skinned patients using a 570- or 590-nm filter (Figs 13.25 and 13.26).
Weiss and Weiss90 have reported increased efficacy by increasing the pulse durations to a maximum of 10 ms as two consecutive pulses separated by a 20-ms delay with a 570-nm cut-off filter and fluences of 70 J/cm2. They have achieved response rates of 74% with two-treatment sessions, with an 8% incidence of temporary hypopigmentation or hyperpigmentation. By combining a shorter pulse (2.4–3 ms) with a longer pulse (7–10 ms), it is theoretically possible to ablate smaller and larger vessels overlying one another in the dermis (Fig. 13.27).
In a European multicenter study, 40 women with leg telangiectasias up to 1 mm in diameter were treated with various parameters.87 For vessels less than 0.2 mm in diameter, a 3-ms pulse of 22 J/cm2 was used. A double pulse of 2.4 ms each, separated by 20 ms, with 35 J/cm2 was given for vessels 0.2 mm to 0.5 mm in diameter. A triple pulse of 3.5, 3.1, and 2.6 ms was used with delays of 20 ms and a fluence of 50 J/cm2 for vessels 0.5 mm to 1 mm in diameter. A clearance rate of 92% occurred in vessels less than 0.2 mm in diameter, 80% clearing in vessels less than 0.5 mm in diameter, and 81% clearing in vessels less than 1 mm in diameter. Vessels did not recur in the 1-year follow-up period. Eleven patients had temporary hyperpigmentation. One patient had temporary hypopigmentation, and two patients had a nonscarring blister.
Schroeter and Neumann91 have reported a similar success rate in treating 40 patients with leg telangiectasia. They used a single pulse of 3 to 4 ms with a 550-nm cut-off filter between 22 and 28 J/cm2 for veins less than 0.2 mm in diameter. A 570-nm cut-off filter was used with a double-pulse technique of 2.4 ms each with a 10- to 20-ms delay between pulses at 24 to 30 J/cm2 for vessels between 0.2 and 2 mm in diameter. With these parameters they achieved over 80% clearance that was maximal at 1-month follow-up. Even with these parameters, which are more conservative than those used by Weiss or Goldman, hyperpigmentation was seen in 20 of the 40 patients, with two of 40 having blistering and three of 40 having hypopigmentation.
Although most authors have reported satisfactory results with IPL in treating leg telangiectasia, this opinion is not universal. Green92 has not seen effective results when he used the IPL and attributes this to having a different objectivity in evaluating his patients compared with those authors previously discussed. He reported low clearance rates with unacceptable side effects.93
Treatment of essential telangiectasia, especially on the legs, is efficiently accomplished with the IPL (see Figs 13.25–13.27). A variety of parameters have been shown to be effective. We recommend testing a few different parameters during the first treatment session and using the most efficient and least painful parameter on subsequent treatments. One study of 14 patients, one of whom had leg telangiectasia, found that a single pulse of 30 J/cm2 delivered through a 550-nm cut-off filter in 5 ms was 100% effective.94 Many other parameters, such as those in Figure 13.12, are also very effective.
One of the most rewarding conditions to treat is essential telangiectasia. This condition, described previously, responds well to PDL, but this can be time consuming and expensive. With IPL, large areas of involvement can be treated quickly and effectively with many different parameters94 (Fig. 13.28).
Fortunately, for those who do not play musical instruments, there are now dozens of IPLs available from many different manufacturers (see Table 13.1).
ND:YAG Laser, 1064 nm
The Nd:YAG laser, 1064 nm, is probably the most effective laser available to treat leg telangiectasia and was first used for this purpose in 1987.18 The average depth of penetration in human skin is 0.75 mm, and reduction to 10% of the incident power occurs at a depth of 3.7 mm.95 A narrow therapeutic fluence range, which reduces both its efficacy and safety, is associated with the 1,064-nm Nd:YAG laser.70 This narrow range may be largely attributed to the formation of methemoglobin (metHb), an oxidized form of hemoglobin that appears during laser-induced blood vessel heating.96 At 1064-nm, metHb shows a significantly stronger absorption than either hemoglobin or oxyhemoglobin. Theoretically, this metHb formation effect is less pronounced at 755 nm.
Apfelberg et al18 treated leg telangiectasia with the Nd:YAG laser equipped with a 1.5-mm sapphire contact probe. Treatment complications included linear hypopigmentation and depressions overlying the skin of the treated vessel. Re-treatment was needed at 6-week intervals. In addition, the cost is high because the disposable sapphire tips are expensive.
The Nd:YAG Q-switched laser modified with filters to treat vessels at 532, 585, 650, and 1064 nm 7 days after sclerotherapy treatment was reported by Cisneros et al.97 With a standard Q-switched Nd:YAG laser, disk-shaped colorant cartridges are inserted into the distal laser arm to produce emitted wavelength of 585 and 660 nm with the cartridges lasting about 50,000 pulses before needing replacement. Fifty percent of the power is lost as the light passes through the cartridges with the laser being used at 2 Hz, 4 to 12 J/cm2 with a 1- to 4-mm diameter pulse. The sclerotherapy solution used was polidocanol (POL) 0.5%.
The 585-nm wavelength was used on the smallest vessels, 532-nm on reticular veins, 1064-nm on deeper blue vessels, and 650-nm and/or 532-nm wavelengths on post-treatment hyperpigmentation. With this technique 54 patients were treated. Two treatment sessions were required to improve 49 patients. Three treatments were required to completely eliminate vessels in 25% of the male patients and 29% of female patients. Ten percent of men and women had small persistent telangiectasia remaining after three treatments. Thirty percent of patients had hyperpigmentation that resolved with treatment. Careful evaluation of this technique fails to disclose the benefit of using a Nd:YAG laser with sclerotherapy. The authors did not separate benefits of the two treatments from each other and, in reality, as described in Chapter 12, sclerotherapy treatment alone was probably responsible for treatment efficacy. Careful review of the photographs indeed shows linear hypopigmentation over treated areas. This experience with different laser wavelengths and pulse durations directed at different color and sized blood vessels has been termed the ‘bimodal or dual wavelength’ approach to treating leg veins.98,99
In an effort to deliver laser energy to the depths of leg veins (often 1–2 mm beneath the epidermis) with thermocoagulation of vessels 1 to 3 mm in diameter, 1064-nm lasers with pulse durations of between 1 and 250 ms have been developed. However, because of the poor absorption of Hb and HbO2 with a 1064-nm wavelength, higher fluences must be used. Depending on the amount of energy delivered, the epidermis must be protected to minimize damage to pigment cells and keratinocytes. Three mechanisms are available to minimize epidermal damage through heat absorption. First, the longer the wavelength, the less energy will be absorbed by melanocytes or melanosomes (Fig. 13.29). This will allow darker skin types to be treated with minimum risks to the epidermis caused by a decrease in melanin interaction. Second, delivering the energy with a delay in pulses greater than the thermal relaxation time for the epidermis (1–2 ms) allows the epidermis to cool conductively between pulses. This cooling effect is enhanced by the application of cold gel on the skin surface, which conducts away epidermal heat more efficiently than air. Finally, the epidermis can be cooled directly to allow the photons to pass through without generating sufficient heat to cause damaging effects.
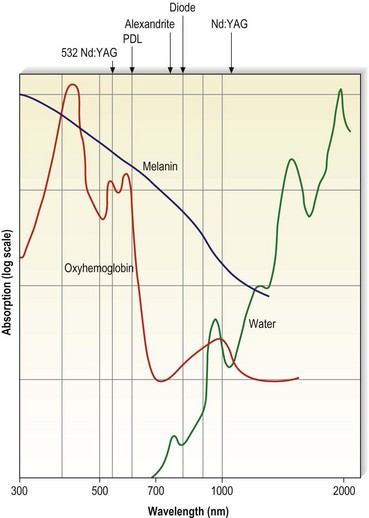
Figure 13.29 Oxyhemoglobin, water, and melanin absorption curves as a function of wavelength.
(Adapted from Boulnois JL: Lasers Med Sci 1:47, 1986.)
Epidermal cooling can be given in many different ways. The simplest method is continuous contact cooling with chilled water, which can be circulated in glass, sapphire, or plastic housings. The laser impulse is given through the transparent housing, which should be constructed to ensure that the laser’s effective fluence is not diminished. The benefit of continuous contact cooling is its simplicity. The disadvantage is that the cooling effect continues throughout the time that the device-crystal is in contact on the skin. This results in a variable degree and depth of cooling determined by the length of time the cold housing is in contact with the skin. This nonselective and variable depth and temperature of cooling may necessitate additional treatment energy so that the cooled vessel will heat up sufficiently to thermocoagulate (Fig. 13.30).
Yet another method for cooling the skin is to deliver a cold spray of refrigerant to the skin that is timed to precool the skin before laser penetration and also to postcool the skin to minimize thermal back-scattering from the laser-generated heat in the target vessel. The authors have termed this latter effect ‘thermal quenching’ (Fig. 13.31). This method reproducibly protects the epidermis and superficial nerve endings. In addition, it acts to decrease the perception of thermal-laser epidermal pain by providing another sensation (cold) to the sensory nerves. Finally, it allows an efficient use of laser energy because of the relative selectivity of the cooling spray that can be limited to the epidermis. The millisecond control of the cryogen spray prevents cooling of the deeper vascular targets and is given in varying amounts so that epidermal absorption of heat is counteracted by exposure to cryogen.
Since the target vessel poorly absorbs 1064-nm wavelength (see Fig. 13.29), a much higher fluence is necessary to cause thermocoagulation. Whereas a fluence of 10 to 20 J/cm2 is sufficient to thermocoagulate blood vessels when delivered at 532 nm or 585 nm, a fluence of 70 to 150 J/cm2 is required to generate sufficient heat absorption at 1064 nm. Various 1064-nm lasers are currently available that meet the criteria for selectively thermocoagulating blood vessels, such as Lumenis One and Vasculite (Lumenis, Santa Clara, Calif.), Varia (CoolTouch Corp., Roseville, Calif.), Lyra (Laserscope, San Jose, Calif.), GentleYAG (Candela, Wayland, Mass.), SmartEpil II (Cynosure, Chelmsford, Mass.), Harmony (Orion Lasers, Fla.), Profile (Sciton, Palo Alto, Calif.), Mydon (WaveLight, Erlangen, Germany), and CoolGlide (Cutera, Burlingame, Calif.) (see Table 13.1). All long-pulse 1064-nm Nd:YAG lasers are not the same. Variables include the spot size, laser output in both fluence as well as how the extended time of the laser pulse is generated, pulse duration, and epidermal cooling. In addition, although many claims are made by the laser manufacturers, few well-controlled peer-reviewed medical studies are available. Because of the variability between the 1064-nm Nd:YAG lasers, a review of the clinical studies with each system will be presented separately.
Vasculite
The Vasculite was the first long-pulsed, 1064-nm laser to be approved by the Food and Drug Administration (FDA) for vascular treatment. The Nd:YAG 1064-nm laser is pulsed with IPL technology. Individual pulses up to 16 ms in length can be delivered as single, double, or triple synchronized pulses with a total maximum fluence of 150 J/cm2. The laser beam is generated in the handpiece and delivered through a sapphire crystal 6, 9, or 3 × 6 mm in diameter. Weiss and Weiss,100,101 Sadick,102 and Goldman103 have reported excellent results in treating leg telangiectasias from 0.1 to 3 mm in diameter. Application of a cool gel to the skin (cooling of the crystal is unnecessary with the most advanced version, Lumenis 1, which is thermokinetically cooled to 4°C) and synchronization of the pulses, allows epidermal cooling and protection. In addition, synchronized timing between pulses can be tailored to thermal relaxation times of blood vessels.
Weiss and Weiss101 treated 30 patients who had been dissatisfied with previous leg vein treatments with either sclerotherapy or other laser lights or IPLs. A single 14- to 16-ms pulse at 110 to 130 J/cm2 was given to treat vessels 1 to 3 mm in diameter. A double pulse of 7 ms separated by 20 to 30 ms at a fluence of 90 to 120 J/cm2 was used to treat vessels 0.6 to 1 mm in diameter. A triple synchronized pulse of 3 to 4 ms at a fluence of 80 to 110 J/cm2 was used to treat vessels 0.3 to 0.6 mm in diameter. Immediate contraction of the vessel was used as an endpoint of treatment followed by urtication. Immediate bruising from vessel rupture occurred in 50% of vessels. At 3 months after treatment the majority of sites had improved by over 75% (Fig. 13.32). Hyperpigmentation was noted in 28% of patients at the 3-month follow-up period. In short, this report demonstrated successful treatment on otherwise difficult vessels and mirrors the authors’ experience. Weiss and Weiss104 also reported on 3-year results in the treatment of leg telangiectasias 0.3 to 3 mm in diameter at slightly higher fluences of 110 to 150 J/cm2. They found an average of 75% improvement in 2.38 treatments. Sixteen percent of patients developed pigmentation which resolved at 6 months and 4% developed TM.
Sadick105 reported on 12-month follow-up in 25 patients with leg veins, with a fluence of 120 J/cm2 given through a 6-mm diameter spot in a 7-ms double pulse to vessels 0.2 to 2 mm in diameter and as a single pulse of 14 ms and a fluence of 130 J/cm2 to vessels 2 to 4 mm in diameter. Using these parameters, 64% of patients could achieve 75% or greater clearance in three treatments. Two of the 25 treated patients who had less than 25% vessel clearance developed a recurrence of the veins within 6 to 12 months. Sixteen percent of patients developed pigmentation which lasted 4 months and 8% developed TM.
Slightly higher fluences were used by Trelles et al106 in treating 40 patients with leg veins up to 4 mm in diameter: 130 J/cm2 given as a double pulse of 7 ms and 140 J/cm2 given as a 14-ms triple pulse was used on vessels less than 2 mm and 2 to 4 mm in diameter, respectively. They used a computerized objective assessment tool to determine efficacy. The percent improvement was not noted, only that 80% of patients were satisfied with treatment.
CoolTouch varia
The CoolTouch Varia combines a multiple train of pulses to generate a pulse width from 10- to 300-ms bursts. Fluences of up to 150 J/cm2 can be generated. A 3- to 10-mm diameter beam is delivered through a fiberoptic cable. Dynamic cooling is given with a cryogen spray that can be delivered before, during, and/or after the laser pulse. The cooling spray can be varied from 5 to 200 ms and can be given in 5- to 30-ms bursts in 5-ms intervals before and/or after the laser pulse. In this manner, in the treatment of larger or deeper vessels, the postcooling quenching cryogen spray can be given 20 to 30 ms after the laser pulse to coincide with conduction of heat absorbed by the vessel propagating back to the epidermis. More superficial and smaller vessels require a shorter delay in the postlaser cooling spray of 5 ms. The authors have found this laser to be therapeutically beneficial in treating leg telangiectasias 0.1 to 2 mm in diameter (Fig. 13.33). A comparative study of two long-pulsed 1064-nm Nd:YAG lasers was performed on 11 patients with leg telangiectasia without (or with previously treated) feeding reticular veins. The CoolTouch Varia was used with a 6-mm diameter spot size at a fluence of 135 J/cm2 with a 25-ms pulse and precooling of 5 ms, with post cooling of 15 ms. The CoolGlide laser was used with a 5-mm diameter spot, 25-ms pulse at 200 J/cm2 and contact cooling. Both lasers produced comparable clearing of 75% in all treated vessels. However, the CoolGlide laser was significantly more painful.107
Two papers were published on the same 23 of 30 leg vein patients (completing the study) treated with the CoolTouch Varia.108,109 Greater than 75% improvement was noted at 85% of treated sites. Transient pigmentation was noted in 6 of 23 patients, with TM in 1 of 23 patients. Fluences of 150 J/cm2 were used for all diameter veins with a 25-ms pulse duration on veins less than 1.5 mm in diameter and 50 to 100 ms on veins 1.5 to 3 mm in diameter. Patients received up to two treatments 4 to 6 weeks apart. One to three passes were required to blanch the targeted vessels. Laser spot diameters and the time of pre- and/or postcooling was not noted in either of the two papers. Patients who had previously had treatment with non-HS sclerotherapy preferred sclerotherapy over laser because of increased pain with laser.
A direct comparison of the CoolTouch Varia with sclerotherapy utilizing sodium tetradecyl sulfate (STS) was performed on 20 patients with size-matched superficial leg telangiectasia 0.5 to 1.5 mm in diameter.110 Laser treatments were given through a 5.5-mm diameter spot at 125–150 J/cm2 with a 25-ms pulse duration. Precooling ranged from 0 to 5 ms and postcooling ranged from 20 to 50 ms with a delay of 5 to 20 ms. The endpoint of laser treatment was vessel contraction. Sclerotherapy with STS 0.25% was followed by 48-hours’ wear of 20- to 30-mmHg graduated compression stockings. Sclerotherapy-treated patients had a significantly better response in fewer treatments with comparable adverse effects.
The CoolTouch Varia is especially useful in treating periorbital telangiectasia and reticular veins. Although these veins may be treated with sclerotherapy (see Chapter 12), we have had near 100% success without adverse effects in treating these vessels with the CoolTouch Varia (Fig. 13.34).
CoolGlide
The CoolGlide can deliver fluences up to 100 J/cm2 through a 10-mm diameter spot. The pulse duration can be varied continuously from 10 to 100 ms. Unlike the other systems, which can deliver each burst at a 1-Hz speed, the CoolGlide can deliver pulses at 2 Hz. Cooling is provided by a contact system that glides in front of the laser beam so that 2 cm of skin is precooled before the laser aperture glides over the treatment site. The authors have also found this system to be effective in treating leg telangiectasias 0.1 to 3 mm in diameter (Fig. 13.35).107 However, the lack of effective, reproducible cooling can lead to the production of epidermal scars, more so than with the other 1064-nm laser systems, as well as an increase in procedural pain (Fig. 13.36).
Fifteen women with 21 sites of leg telangiectasia 0.25 to 4 mm in diameter were treated twice, separated by an interval of 6 to 8 weeks, with the CoolGlide using a 7-mm spot, fluence of 90 to 160 J/cm2 and pulse durations of 10 to 50 ms.111 Significant improvement was seen in 71% of sites but hyperpigmentation was present in 61% of sites at 3-month follow-up. A second study on 20 patients with reticular veins 1 to 3 mm in diameter was performed using 100 J/cm2 and a 50-ms pulse without mention of the laser spot diameter.112 Although 66% of the vessels cleared by more than 75% with one treatment at 3 months, pain was significant, especially without the use of EMLA cream applied for 1 hour. Unfortunately, longer follow-up was not reported.
Lyra
The Lyra long-pulse 1064-nm Nd:YAG laser was used to treat 20 patients with leg telangiectasias 0.5 to 5 mm in diameter with 100 to 200 J/cm2 at 50 to 100 ms with a 3- to 5-mm diameter spot and one to four treatments.113 Comparable telangiectasias on the same patient received a single sclerotherapy treatment with STS 0.6%. No compression was used. Even at these parameters with excessive concentration of STS without compression, and four laser treatments versus one sclerotherapy treatment, adverse effects and treatment efficacy were not statistically different between the two treatment modalities. Patient surveys found that 35% preferred laser and 45% preferred sclerotherapy.
Sadick114 also evaluated the Lyra with a 30- to 50-ms pulse duration, 1.5-mm diameter spot, 400 to 600 J/cm2 for red vessels and a 50- to 60-ms pulse, 1- to 3-mm diameter spot, 250 to 370 J/cm2 for blue vessels through a 4°C cold window for three treatments. At 6 months, 80% of vessels had greater than 75% clearance. This was a limited study on 10 patients. Two of the 10 had pigmentation lasting up to 6 months and TM occurred in 1 patient. Moderate discomfort was experienced by all patients.
Quantel medical multipulse mode
The most recent development in long-pulse 1064-nm Nd:YAG technology has been the production of a nonuniform pulse sequence mode device with contact cooling to 5°C.115 This device has a fluence of 300 to 360 J/cm2 through a 2-mm diameter spot. The rationale for multiple pulsing is to convert HbO2 to met-Hb, which will be absorbed better by 1064 nm. The pulse duration consists of a series of three 3.5-ms pulses each separated by 250 ms. Sixty percent of the energy is delivered in the first pulse, with 20% in each of the next two pulses. In an initial study on 11 patients with blue leg veins 1 to 2 mm in diameter, patients had up to three treatments at 6-week intervals. There was 98% clearance after three treatments, with moderate pain with each treatment.
SmartEpil lS
A comparative study of the long-pulsed 1064-nm Nd:YAG laser with sclerotherapy was performed on 14 patients with leg telangiectasias 0.5 to 2 mm in diameter.116 The Nd:YAG laser was used at 100 to 125 J/cm2 through a 2.5-mm diameter spot and a 10-ms pulse without cooling. Sclerotherapy was performed with POL 0.5% without post-treatment compression. An evaluation of combinations of laser and sclerotherapy was also performed. There was no statistical difference in efficacy between the four different treatment modalities. However, the sclerotherapy-treated veins had better resolution.
Evaluation of Combined Laser–Sclerotherapy Treatment of Leg Telangiectasia
Goldman et al52 hypothesized that the combination of sclerotherapy with laser thermocoagulation may be effective in the treatment of leg veins as early as 1990. Twenty-seven patients, with either bilaterally symmetrical telangiectatic patches or a large ‘sunburst’ telangiectatic flare that could be divided into two separate treatment sites, were evaluated.53 Patients were treated at one site with the 0.45-ms, 585-nm PDL alone, and at the other site with laser fluences 1 to 2 J/cm2 less than those used with PDL alone immediately before injection of the telangiectasia with POL 0.25%, 0.5%, or 0.75%, with a volume of 0.1 to 0.25 mL per injection site (Fig. 13.37). Forty-four percent of combination treated areas completely resolved (Table 13.3 and Fig. 13.38). There appeared to be little difference in efficacy and adverse sequelae with concentrations of POL between 0.25% and 0.75%. There did appear to be an increased efficacy of treatment with laser energies of 7.5 to 7.75 J/cm2. As with the PDL alone, treatment site did not appear to significantly affect outcome except for an increased incidence of complications in the ankle and knee areas.
The most significant difference between the PDL alone and combination treatment was the incidence of complications. With combination treatment, post-treatment ulceration and TM occurred in 11% of treated areas, compared with no adverse sequelae with PDL alone. Six of 23 nonulcerated treatment sites developed persistent pigmentation beyond 1 year. Two of 27 sites developed TM that lasted over 1 year. Four of 27 treatment sites developed superficial ulceration. In these ulcerated patients, laser fluences were equal to or greater than 6.5 J/cm2 and POL concentration was equal to or greater than 0.5% (Table 13.4 and Figs 13.39 and 13.40).
Theoretically, combining administration of a laser with an injected sclerosant to treat leg telangiectasias could decrease the amount of each therapy necessary to yield optimal results, thus mitigating the individual side effect profiles of each. Galeckas et al117 reported the successful treatment of a pyogenic granuloma by combining the 1,064-nm laser with glycerin sclerotherapy. A series of three sessions, spaced 2 to 3 weeks apart, were administered. The spot size was varied over subsequent visits to parallel the decreasing size of the lesion; fluences varied between 200 to 360 J/cm2, and the pulse width was kept constant at 40 ms. Immediately after the laser treatment, the patient underwent an intralesional injection with 0.1 to 0.2 mL of compounded glycerin (14.5 mL of glycerin, 6 mL of bacteriostatic water, and 9.5 mL of lidocaine 1% with epinephrine (adrenaline)). The lesion, which was located on the patient’s lip, resolved completely and an excellent cosmetic outcome remained at a 2-year follow-up visit. This case is pertinent as, although the combined laser and sclerosant treatment was not administered to leg telangiectasias, it was used to treat a thick and deep vascular lesion that necessitated a longer wavelength device. Theoretically, this technique could be used to treat leg telangiectasias of the deeper, larger variety. However, given the potential for scarring associated with the 1064-nm Nd:YAG laser, the superiority of sclerotherapy alone in many cases, and the potential for synergistic adverse effects when lasers are used in combination with sclerotherapy, the authors do not routinely advise combining these two modalities to treat leg telangiectasias.
Conclusions
Since sclerotherapy treatment is relatively cost-effective compared to laser or IPL treatment, when is it appropriate to use this advanced therapy? Obviously, needle-phobic patients will tolerate this technology even though the pain from lasers and IPL is more intense than that of sclerotherapy with all but hypertonic solutions. Patients who are prone to TM are also appropriate candidates. Vessels below the ankle are particularly appropriate to treat with lasers and light, since sclerotherapy has a relatively high incidence of ulceration in this area due to the higher distribution of arteriovenous anastomosis (see Chapter 8). Finally, patients who have vessels that are resistant to sclerotherapy are excellent candidates. Efficacy of 75% clearance with two to three IPL treatments has been reported in sclerotherapy-resistant vessels.118
In a similar ‘vein’, enhanced efficacy of treatment may occur by combining sclerotherapy with lasers or IPL. This technique is not new and was even reported approximately 30 years ago by the Italian vascular surgeon Leonardo Corcos, who used the argon laser to spot-weld telangiectasia so the sclerosing solution could have prolonged contact with the vessel wall.25 This combination technique also gives the patient the opportunity to experience ‘laser’ treatment, which is perceived as more advanced than merely injecting a solution into a vein.
However, the answer is also no, as presently available lasers still require skillful use for safe and effective treatment. The laser of the future was detailed in a September 2001 publication.119 This ideal laser will have a built-in thermal sensor to detect both epidermal and vascular heating, thus enabling it to automatically regulate the fluence so that the vessel is completely thermocoagulated, while cooling the epidermis to maintain its temperature at that of one below a damaging threshold. Even better would be an infrared sensor that would determine the location of feeding dermal vessels so that they too can be treated along with the visible telangiectasia. One could imagine in the future, the patient placing the leg into a laser machine that would map the visible veins to be thermocoagulated and automatically treat the entire superficial venous network. At this time, the only barrier preventing the development of such a laser is money and the willingness of a company to produce a machine of this type.
1 Ross V, Anderson RR. Laser-tissue interactions. In: Goldman MP, editor. Cosmetic cutaneous laser surgery. New York: Elsevier, 2006.
2 Goldman MP. Laser treatment of cutaneous vascular lesions. In: Goldman MP, editor. Cosmetic cutaneous laser surgery. New York: Elsevier, 2006.
3 Dover JS, Sadick NS, Goldman MP. The role of lasers and light sources in the treatment of leg veins. Dermatol Surg. 1999;25:328.
4 Anderson AR, Parrish JA. The optics of human skin. J Invest Dermatol. 1981;77:13.
5 Ross EV, Domankevitz Y. Laser treatment of leg veins: physical mechanisms and theoretical considerations. Lasers Surg Med. 2005;36:105.
6 Kunishige JH, Goldberg LH, Friedman PM. Laser therapy for leg veins. Clinics Dermatol. 2007;25:454.
7 Weiss RA, Weiss MA. Resolution of pain associated with varicose and telangiectatic leg veins after compression sclerotherapy. J Dermatol Surg Oncol. 1990;16:333.
8 Bernstein EF. Clinical characteristics of 500 consecutive patients presenting for removal of lower extremity spider veins. Dermatol Surg. 2001;27:31.
9 Goldman MP, Kaplan RP, Duffy DM. Postsclerotherapy hyperpigmentation: a histologic evaluation. J Dermatol Surg Oncol. 1987;13:547.
10 Duffy DM. Small vessel sclerotherapy: an overview. In: Callen JP, Dahl MV, Golitz LE, et al, editors. Advances in dermatology. Chicago: Year Book Medical Publishers, 1988.
11 Faria JLde, Moraes IN. Histopathology of the telangiectasias associated with varicose veins. Dermatologia. 1963;127:321.
12 Goldman MP. Laser and sclerotherapy treatment of leg veins: my perspective on treatment outcomes. Dermatol Surg. 2002;28:969.
13 Braverman IM. Ultrastructure and organization of the cutaneous microvasculature in normal and pathologic states. J Invest Dermatol. 1989;93(Suppl):2S.
14 Wokalek H, Vanscheidt W, Martay K, Leder O. Morphology and localization of sunburst varicosities: an electron microscopic and morphometric study. J Dermatol Surg Oncol. 1989;15:149.
15 Bodian EL. Sclerotherapy. Semin Dermatol. 1987;6:238.
16 Sommer A, Van Mierlo PLH, Neumann HAM, et al. Red and blue telangiectasia: differences in oxygenation? Dermatol Surg. 1997;23:55.
17 Bean WB. Vascular spiders and related lesions of the skin. Springfield, Ill.: Charles C Thomas; 1958.
18 Apfelberg DB, Smith T, Maser MR, et al. Study of three laser systems for treatment of superficial varicosities of the lower extremity. Lasers Surg Med. 1987;7:219.
19 Apfelberg DB, Maser MR, Lash H, et al. Use of the argon and carbon dioxide lasers for treatment of superficial venous varicosities of the lower extremity. Lasers Surg Med. 1984;4:221.
20 Frazzetta M, Palumbo FP, Bellisi M, et al. Considerations regarding the use of the CO2 laser: personal case study. Laser. 1989;2:4.
21 Landthaler M, Haina D, Waidelich W, Braun-Falco O. Laser therapy of venous lakes (Bean-Walsh) and telangiectasias. Plast Reconstr Surg. 1984;73:78.
22 Glaich AS, Goldberg LH, Dai T, et al. Fractional photothermolysis for the treatment of telangiectatic matting: A case report. J Cosmet Laser Ther. 2007;9:101.
23 Apfelburg DB, Maser MR, Lash H. Argon laser management of cutaneous vascular deformities. West J Med. 1976;124:99.
24 Dixon JA, Rotering RH, Huethner SE. Patient’s evaluation of argon laser therapy of port-wine stain, decorative tattoos, and essential telangiectasia. Lasers Surg Med. 1984;4:181.
25 Corcos L, Longo L. Classification and treatment of telangiectases of the lower limbs. Laser. 1988;1:22.
26 Chess C, Chess Q. Cool laser optics treatment of large telangiectasia of the lower extremities. J Dermatol Surg Oncol. 1993;19:74.
27 Tan OT, Kerschmann R, Parrish JA. Effect of skin temperature on selective vascular injury caused by pulsed laser irradiation. J Invest Dermatol. 1985;85:441.
28 Bruck LB. Micro-contact argon laser probe may enhance therapy, Dermatology Times, p. 15, October, 1988.
29 Weiss RA, Weiss MA. Combination intense pulsed light and sclerotherapy: a synergistic effect. Presented at the 11th Annual Meeting of the North American Society of Phlebology, Palm Desert, Calif., November, 1997.
30 West TB, Alster TS. Comparison of the long-pulse dye (590–595 nm) and KTP (532 nm) lasers in the treatment of facial and leg telangiectasias. Dermatol Surg. 1998;24:221.
31 Quintana AT, Grossman MG, Kauvar ANB, et al. Removal of leg veins with laserscope potassium titanyl phosphate-532 nm and Dermaststat 2 mm handpiece. Presented at the 18th Annual Meeting of the American Society of Laser Medicine and Surgery, San Diego, Calif., April 5–7, 1998.
32 Adrian RM. Treatment of leg telangiectasias using a long-pulse frequency-doubled neodymium:YAG laser at 532 nm. Dermatol Surg. 1998;24:19.
33 McMeekin TO. Treatment of spider veins of the leg using a long-pulsed Nd:YAG laser (VersaPulse) at 532 nm. J Cutan Laser Ther. 1999;1:179.
34 Bernstein EF, Kornbluth S, Brown DB, Black J. Treatment of spider veins using a 10 millisecond pulse-duration frequency-doubled neodymium YAG laser. Dermatol Surg. 1999;25:316.
35 Narurkar VA. The efficacy of the Coherent VersaPulse 532 nm variable pulse laser for the treatment of superficial leg telangiectasia. Presented at the 18th Annual Meeting of the American Society of Laser Medicine and Surgery, San Diego, Calif., April 5–7, 1998.
36 Massey RA, Katz BE. Successful treatment of spider leg veins with a high-energy, long-pulse, frequency-doubled neodymium:YAG laser (Help-G). Dermatol Surg. 1999;25:677.
37 Krause L. Regarding successful treatment of spider leg veins. Dermatol Surg. 2000;26:169.
38 Fournier N, Brisot D, Mordon S. Treatment of telangiectases with a 532 nm KTP laser in multipulse mode. Dermatol Surg. 2002;28:564.
39 Woo WK, Jasim ZF, Handley JM. 532-nm Nd:YAG and 595-nm pulsed dye laser treatment of leg telangiectasia using ultralong pulse duration. Dermatol Surg. 2003;29:1176.
40 Raskin B, Fany RR. Laser treatment for neovascular formation. Lasers Surg Med. 2004;34:189.
41 Sadick NS, Weiss R. The utilization of a new yellow light laser (578 nm) for the treatment of class I red telangiectasia of the lower extremities. Dermatol Surg. 2002;28:21.
42 Goldman MP, Fitzpatrick RE. Cutaneous laser surgery: the art and science of selective photothermolysis, 2nd ed. St Louis: Mosby; 1998.
43 Garden JM, Tan OT, Kerschmann R, et al. Effect of dye laser pulse duration on selective cutaneous vascular injury. J Invest Dermatol. 1986;87:653.
44 Polla LL, Tan OT, Garden JM, Parrish JA. Tunable pulsed dye laser for the treatment of benign cutaneous vascular ectasia. Dermatologica. 1987;174:11.
45 Ashton N. Corneal vascularization. In: Duke-Elder S, Perkins ES, editors. The transparency of the cornea. Oxford: Blackwell, 1960.
46 Folkman J, Klagsbrun M. Angiogenic factors. Science. 1987;235:442.
47 Ryan TJ. Factors influencing the growth of vascular endothelium in the skin. Br J Dermatol. 1970;82(Suppl 5):99.
48 Dvorak HF. Tumors: wounds that do not heal: similarities between tumor stroma generation and wound healing. N Engl J Med. 1986;315:1650.
49 Majewski S, Kaminski M, Jablonska S, et al. Angiogenic capability of peripheral blood mononuclear cells in psoriasis. Arch Dermatol. 1985;121:1018.
50 Nakagawa H, Tan OT, Parrish JA. Ultrastructural changes in human skin after exposure to a pulsed laser. J Invest Dermatol. 1985;84:396.
51 Tan TT, Carney JM, Margolis R, et al. Histologic responses of port-wine stains treated by argon, carbon dioxide, and tunable dye lasers: a preliminary report. Arch Dermatol. 1986;122:1016.
52 Goldman MP, Martin DE, Fitzpatrick RE, Ruiz-Esparza J. Pulsed dye laser treatment of telangiectases with and without subtherapeutic sclerotherapy: clinical and histologic examination in the rabbit ear vein model. J Am Acad Dermatol. 1990;23:23.
53 Goldman MP, Fitzpatrick RE. Pulsed-dye laser treatment of leg telangiectasia: with and without simultaneous sclerotherapy. J Dermatol Surg Oncol. 1990;16:338.
54 Perez B, Nunez M, Boixeda P, et al. Progressive ascending telangiectasia treated with the 585 nm flashlamp-pumped pulsed dye laser. Lasers Surg Med. 1997;21:413.
55 Fajardo LF, Prionas SD, Kowalski J, Kwan HH. Hyperthermia inhibits angiogenesis. Radiat Res. 1988;114:297.
56 Sadick NS, Sorhaindo L. An evaluation of post-sclerotherapy laser compression and its efficacy in the treatment of leg telangiectasias. Phlebol. 2006;21:191.
57 Reichert D. Evaluation of the long-pulse dye laser for the treatment of leg telangiectasias. Dermatol Surg. 1998;24:737.
58 Hsia J, Lowery JA, Zelickson B. Treatment of leg telangiectasia using a long-pulse dye laser at 595 nm. Lasers Surg Med. 1997;20:1.
59 Bernstein EF. Then new-generation, high-energy, 595 nm, long pulse-duration, pulsed-dye laser effectively removes spider veins of the lower extremity. Lasers Surg Med. 2007;39:218.
60 Lee PK, Lask GP. Treatment of leg veins by long pulse dye laser (Sclerolaser). Lasers Surg Med. 1997;9(Suppl):40.
61 Bernstein EF, Lee J, Lowery J, et al. Treatment of spider veins with the 595 nm pulsed-dye laser. J Am Acad Dermatol. 1999;39:746.
62 Hohenleutner U, Walther T, Wenig M, et al. Leg telangiectasia treatment with a 1.5 ms pulsed dye laser and ice cube cooling of the skin: 595 vs. 600 nm. Lasers Surg Med. 1998;23:72.
63 Alora MB, Stern RS, Arndt KA, Dover JS. Comparison of the 595 nm long pulse (1.5 ms) and ultra-long pulse (4 ms) lasers in the treatment of leg veins. Dermatol Surg. 1999;25:445.
64 Polla LL. Treatment of leg telangiectasia with a 595 nm LPDL. Lasers Surg Med Suppl. 2002;14:78.
65 Weiss RA, Weiss MA. Long pulsed dye laser (LPDL) treatment of resistant telangiectatic matting of the legs. Lasers Surg Med Suppl. 2002;14:86.
66 Woo WK, Jasim ZF, Handley JM. 532-nm Nd:YAG and 595-nm pulsed dye laser treatment of leg telangiectasia using ultralong pulse duration. Dermatol Surg. 2003;29:1176.
67 Kono T, Yamaki T, Ercocen AR, et al. Treatment of leg veins with the long pulse dye laser using variable pulse durations and energy fluences. Lasers Surg Med. 2004;35:62.
68 Adrian RM. Long pulse normal mode alexandrite laser treatment of leg veins. Presented at the 18th Annual Meeting of the American Society of Laser Medicine and Surgery, San Diego, Calif., April 5–7, 1998.
69 McDaniel DH, Ash K, Lord J, et al. Laser therapy of spider leg veins: clinical evaluation of a new long pulsed alexandrite laser. Dermatol Surg. 1999;25:52.
70 Izikson L, Nelson JS, Anderson RR. Treatment of hypertrophic and resistant port wine stains with a 755-nm laser: A case series of 20 patients. Lasers Surg Med. 2009;41:427.
71 Kauvar ANB, Lou WW. Pulsed alexandrite laser for the treatment of leg telangiectasia and reticular veins. Arch Dermatol. 2000;136:1371.
72 Brunnberg S, Lorenz S, Landthaler M, Hohenleutner U. Evaluation of the long pulsed high fluence alexandrite laser therapy of leg telangiectasia. Lasers Surg Med. 2002;31:359.
73 Ross EV, Meehan KJ, Gilbert S, et al. Optimal pulse durations for the treatment of leg telangiectasias with an alexandrite laser. Lasers Surg Med. 2009;41:104.
74 Eremia S, Li C, Umar SH. A side-by-side comparative study of 1064 nm Nd:YAG, 810 nm diode and 755 nm alexandrite lasers for treatment of 0.3–3 mm leg veins. Dermatol Surg. 2002;28:224.
75 Dierickx CC, Dugue V, Anderson RR. Treatment of leg telangiectasia by a pulsed 800 nm diode laser. Presented at the 18th Annual Meeting of the American Society of Laser Medicine and Surgery, San Diego, Calif., April 5–7, 1998.
76 Trelles MA, Allones I, Álvarez J, et al. The 800-nm diode laser in the treatment of leg veins: assessment at 6 months. J Am Acad Dermatol. 2006;54:282.
77 Garden JM, Bakus AD, Miller ID. Diode laser treatment of leg veins. Presented at the 18th Annual Meeting of the American Society of Laser Medicine and Surgery, San Diego, Calif., April 5–7, 1998.
78 Wollina U, Konrad H, Schmidt W-D, et al. Response of spider leg veins to pulsed diode laser (810 nm): a clinical, histological and remission spectroscopy study. J Cosmet Laser Ther. 2003;5:154.
79 Kaudewitz P, Kloverkorn W, Rother W. Effective treatment of leg vein telangiectasia with a new 940 nm diode laser. Dermatol Surg. 2001;27:101.
80 Kaudewitz P, Kloverkorn W, Rother W. Treatment of leg vein telangiectasias: 1-year results with a new 940 nm diode laser. Dermatol Surg. 2002;28:1031.
81 Passeron T, Olivier V, Duteil L, et al. The new 940-nanometer diode laser: an effective treatment for leg venulectasia. J Am Acad Dermatol. 2003;48:768.
82 Chess C. Prospective study on combination diode laser and radiofrequency energies (ELOS™) for the treatment of leg veins. J Cosmet Laser Ther. 2004;6:86.
83 Sadick NS, Trelles MA. A clinical and histological, and computer-based assessment of the polaris LV, combination diode, and radiofrequency system, for leg vein treatment. Lasers Surg Med. 2005;36:98.
84 Trelles MA, Martín-Vázquez M, Trelles OR, et al. Treatment effects of combined radio-frequency current and a 900 nm diode laser on leg blood vessels. Lasers Surg Med. 2006;38:185.
85 Prieto V, Zhang P, Sadick NS. Comparison of a combination diode laser and radiofrequency device (Polaris®) and a long-pulsed 1064-nm Nd:YAG laser (Lyra®) on leg telangiectases. Histologic and immunohistochemical analysis. J Cosmet Laser Ther. 2006;8:191.
86 Bone Salat C. Preliminary study on varix treatment with laser. Presented at the Pan-American Congress on Phlebology and Lymphology, Cordoba, Argentina, June 1–3, 2000.
87 Schroeter CA, Wilder D, Reineke T, et al. Clinical significance of an intense, pulsed light source on leg telangiectasias of up to 1 mm diameter. Eur J Dermatol. 1997;7:38.
88 Goldman MP, Eckhouse S. Photothermal sclerosis of leg veins. Dermatol Surg. 1996;22:323.
89 Hellwig S, Schroter C, Raulin C. Behandlung essentieller telangiektasien durch das Photoderm VL. Z Hautk. 1996;71:44.
90 Weiss RA, Weiss MA. Intense pulsed light revisited: progressive increase in pulse durations for better results on leg veins. Presented at the 11th Annual Meeting of the North American Society of Phlebology, Palm Desert, Calif., November 1997.
91 Schroeter CA, Neumann HAM. An intense light source: the PhotoDerm-VL flashlamp as a new treatment possibility for vascular skin lesions. Dermatol Surg. 1998;24:743.
92 Green D. Pitfalls in the evaluation of ablative therapy for telangiectases. Dermatol Surg. 1998;24:1143.
93 Green D. Photothermal removal of telangiectasias of the lower extremities with the PhotoDerm VL. J Am Acad Dermatol. 1998;38:61.
94 Raulin C, Weiss RA, Schonermark MP. Treatment of essential telangiectasias with an intense pulsed light source (PhotoDerm VL). Dermatol Surg. 1997;23:941.
95 Glassberg E, Lask GP, Tan EM, Uitto J. The flashlamp-pumped 577-nm pulsed tunable dye laser: clinical efficacy and in vitro studies. J Dermatol Surg Oncol. 1988;14:1200.
96 Black JF, Wade N, Barton JK. Mechanistic comparison of blood undergoing laser photocoagulation at 532 and 1,064 nm. Lasers Surg Med. 2005;36:155.
97 Cisneros JL, Del Rio R, Palou J. Sclerosis and the Nd:YAG, Q-switched laser with multiple frequency for treatment of telangiectases, reticular veins, and residual pigmentation. Dermatol Surg. 1998;24:1119.
98 Sadick NS, Weiss RA, Goldman MP. Advances in laser surgery for leg veins: bimodal wavelength approach to lower extremity vessels, new cooling techniques, and longer pulse durations. Dermatol Surg. 2002;28:16.
99 Sadick NS. A dual wavelength approach for laser/intense pulsed light source treatment of lower extremity veins. J Am Acad Dermatol. 2002;46:66.
100 Weiss RA, Weiss MA. Initial results with a new synchronized pulsed 1064 nm laser for larger telangiectasia. Lasers Med Surg. 1999;11(Suppl):21.
101 Weiss RA, Weiss MA. Early clinical results with a multiple synchronized pulse 1064 nm laser for leg telangiectasias and reticular veins. Dermatol Surg. 1999;25:399.
102 Sadick NS. The utilization of a new Nd:YAG pulsed laser (1064 nm) for the treatment of varicose veins. Lasers Med Surg. 1999;11(Suppl):21.
103 Goldman MP. Treatment of leg telangiectasia with three different 1064 nm lasers. Presented at the 24th Annual Hawaii Dermatology Seminar, The Big Island, Hawaii, Feb 7, 2000. Published as: Laser treatment of leg veins with 1064 nm long-pulsed lasers]. Cosmet Dermatol. 2000;13:27.
104 Weiss MA, Weiss RA. Three year results with the long pulsed Nd:YAG 1064 laser for leg telangiectasia. Presented at the Annual Meeting of the American Society for Dermatologic Surgery, Dallas, Tex., October 2001.
105 Sadick NS. Long-term results with a multiple synchronized-pulse 1064 nm Nd:YAG laser for the treatment of leg venuelectasias and reticular veins. Dermatol Surg. 2001;27:365.
106 Trelles MA, Allones I, Martin-Vazquez MJ, et al. Long pulse Nd:YAG laser for treatment of leg veins in 40 patients with assessments at 6 and 12 months. Lasers Surg Med. 2004;35:68.
107 Bowes LE, Goldman MP. Treatment of leg telangiectasias with a 1064 nm long pulse Nd:YAG laser using dynamic vs contact cooling: a comparative study. Laser Surg Med Suppl. 2002;14:40.
108 Eremia S, Li CY. Treatment of leg and face veins with a cryogen spray variable pulse width 1064-nm Nd:YAG laser – a prospective study of 47 patients. J Cosmet Laser Ther. 2001;3:147.
109 Li CY, Eremia S. Treatment of leg and face veins with a cryogen spray, variable pulse width 1064 nm Nd:YAG laser – a prospective study of 47 patients. Am J Cosmet Surg. 2002;19:3.
110 Lupton JR, Alster TS, Romero P. Clinical comparison of sclerotherapy versus long-pulsed Nd:YAG laser treatment for lower extremity telangiectases. Dermatol, Surg. 2002;28:694.
111 Rogachefsky AS, Silapunt S, Goldberg DJ. Nd:YAG laser (1064 nm) irradiation for lower extremity telangiectasias and small reticular veins: efficacy as measured by vessel color and size. Dermatol Surg. 2002;28:220.
112 Omura NF, Dover JS, Arndt KA, Kauvar ANB. Treatment of reticular leg veins with a 1064 nm long-pulsed Nd:YAG laser. J Am Acad Dermatol. 2003;48:76.
113 Coles MC, Werner RS, Zelickson BD. Comparative pilot study evaluating the treatment of leg veins with a long pulse Nd:YAG laser and sclerotherapy. Lasers Surg Med. 2002;30:154.
114 Sadick NS. Laser treatment with a 1064-nm laser for lower extremity class I-III veins employing variable spots and pulse width parameters. Dermatol Surg. 2003;29:916.
115 Mordon S, Brisot D, Fournier N. Using a ‘non uniform pulse sequence’ can improve selective coagulation with a Nd:YAG laser (1.06 µm) thanks to met-hemoglobin absorption: a clinical study on blue veins. Lasers Surg Med. 2003;32:160.
116 Levy JL, Elbahr C, Jouve E, Mordon S. Comparison and sequential study of long pulsed Nd:YAG 1,064 nm laser and sclerotherapy in leg telangiectasias treatment. Lasers Surg Med. 2004;34:273.
117 Galeckas KJ, Uebelhoer NS. Successful treatment of pyogenic granuloma using a 1,064-nm laser followed by glycerin sclerotherapy. Dermatol Surg. 2009;35:530.
118 Weiss RA, Weiss MA. Photothermal sclerosis of resistant telangiectatic leg and facial veins using the PhotoDerm VL. Presented at the Annual Meeting of the Mexican Academy of Dermatology, Monterey, Mexico, April 24, 1996.
119 Goldman MP. Are lasers or non-coherent light sources the treatment of choice for leg veins? A look into the future. Cosmet Dermatol. 2001;14:58.