Chapter 22 Traumatic Brain Injury
Major Head Trauma
How Does Head Trauma Cause TBI?
Direct Force
A blow – by its direct mechanical force (a coup injury) – disrupts the underlying, delicate brain tissue (the parenchyma). As with strokes, trauma causes cell death by necrosis and its accompanying inflammatory changes, particularly monocular cell infiltration. (In contrast, neurodegenerative diseases, such as Huntington disease and amyotrophic lateral sclerosis, cause cell death by apoptosis [see Chapter 18].)
In addition to causing the coup injury, head trauma throws the brain against the opposite inner surface (table) of the skull, which causes a contrecoup injury of that surface of the brain. Damage from contrecoup injuries frequently surpasses that from the coup injury. Contrecoup injuries frequently damage the temporal and frontal lobes because their anterior surfaces abut against the sharp edges of the skull’s anterior and middle cranial fossae (Fig. 22-1). Depending on its severity, damage of the frontal and temporal lobes characteristically leads to memory impairment and personality changes.
Diffuse Axonal Shearing
Diffuse axonal shearing, which also leads to TBI, consists not only of trauma to long subcortical white-matter axons, but also damage to cytoskeletal elements and a fatal intracellular influx of calcium. Although neither computed tomography (CT) nor magnetic resonance imaging (MRI) can illustrate diffuse axonal shearing, diffusion tensor imaging readily detects it by showing traumatic disruption of white-matter tracts (see Chapter 20).
Bleeding Within the Skull, but Outside the Brain
• Pia mater, the innermost layer, is a thin, translucent, vascular membrane adherent to the cerebral gyri. It follows gyri into sulci and thus allows for a generous region, the subarachnoid space, between it and the immediately overlying layer – the arachnoid mater.
• Arachnoid mater, also thin, spans the tops of gyri, capping the sulci. The subarachnoid space, which contains the cerebrospinal fluid (CSF), envelops the brain and, continuing downward within the spinal canal to the sacrum, the spinal cord and cauda equina. Neurologists performing a spinal tap or lumbar puncture insert a special needle into the subarachnoid space below the conus medullaris (lower end of the spinal cord), which is situated between the T12 and L1 vertebrae, to sample CSF.
• Dura mater, the outermost layer of the meninges, is a thick fibrous tissue adherent to the interior surface of the skull. Two of its infoldings, the falx and tentorium, support the brain and house most of its venous drainage. Neurologists designate the space between the skull and the underlying dura as the epidural space, and between the dura and the underlying arachnoid as the subdural space. Major head trauma may cause hematomas in either or both of these areas.
Epidural hematomas, which typically result from temporal bone fractures with concomitant middle meningeal artery lacerations, are essentially rapidly expanding, high-pressure, fresh blood clots (Fig. 22-2). They compress the underlying brain and force it through the tentorial notch, i.e., they produce transtentorial herniation (see Fig. 19-3). Unless surgery can immediately arrest the bleeding, epidural hematomas are usually fatal.
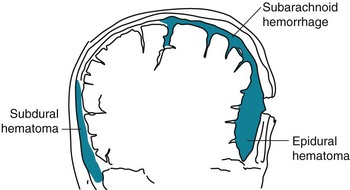
FIGURE 22-2 Meningeal arterial bleeding, which usually results from a blow forceful enough to fracture the skull, causes epidural hematomas. In contrast, venous bleeding, usually slower and under less pressure, causes subdural hematomas. Ruptured aneurysms and head trauma often cause subarachnoid hemorrhage (SAH). In SAH, blood spreads within the subarachnoid space over the convexities, between the gyri, into the interhemispheric fissure, and down into the spinal canal.
As an example, a victim of an assault with a baseball bat lost consciousness when struck. After regaining consciousness for 1 hour, the victim lapsed into coma and developed fatal decerebrate posture (see Fig. 19-3). A CT showed a temporal skull fracture and an underlying epidural hematoma (see Fig. 20-9D). Neurologists label the period when the victim transiently regained consciousness the lucid interval.
In contrast, subdural hematomas usually result from slowly bleeding bridging veins, under relatively low pressure, into the subdural space (see Fig. 20-9). Dark, venous blood generally oozes into the extensive subdural space until the expanding hematoma encounters underlying brain. The brain dampens bleeding and suppresses further expansion of the subdural hematoma. However, if the hematoma continues to expand, it may lead to cerebral transtentorial herniation or cerebellar herniation through the foramen magnum. Survivors often have permanent brain damage from the initial trauma and the pressure from the subdural hematoma.
Acute subdural hematomas, which are most apt to occur in alcoholics, individuals medicated with warfarin, or the elderly (see later), produce headache, confusion, and a deteriorating level of consciousness over several hours to 1 or 2 days. Depending on the extent of the bleeding and time until the diagnosis, patients may develop focal signs and herniation. A history of head trauma does not necessarily precede the symptoms. CTs show acute, dense blood in the subdural space (see Fig. 20-9).
Chronic subdural hematomas, ones that have developed and persisted for weeks, usually have spread extensively in the subdural space (see Fig. 20-9). They typically give rise to insidiously developing headache, change in personality, and cognitive impairment, but only subtle focal physical deficits (see Chapters 19 and 20). Although subdural hematomas may spontaneously resolve, they often require surgical evacuation. Because subdural hematoma patients’ cognitive decline is rapid, but treatment usually reverses their symptoms, neurologists often include subdural hematomas as an explanation for “rapidly developing dementia” and a “reversible cause of dementia” (see Chapter 7).
Posttraumatic Coma and Delirium
Following head trauma, as well as at other times, neurologists often classify patients’ level of consciousness as alert, lethargic, stuporous, or comatose. They may also use the Glasgow Coma Scale (GCS), which measures three readily obvious neurologic functions: eye opening, speaking, and moving (Table 22-1). In major head trauma, the GCS correlates closely with survival and neurologic sequelae; however, in minor head trauma, it correlates poorly. Moreover, physicians cannot appropriately include the GCS as part of a standard mental status examination for patients suspected of having dementia or circumscribed neuropsychologic deficits.
Category | Score | |
---|---|---|
Eyes opening | Never | 1 |
To pain | 2 | |
To verbal stimuli | 3 | |
Spontaneously | 4 | |
Best verbal response | None | 1 |
Incomprehensible sounds | 2 | |
Inappropriate words | 3 | |
Disoriented and converses | 4 | |
Oriented and converses | 5 | |
Best motor response | None | 1 |
Extension* | 2 | |
Flexion† | 3 | |
Flexion withdrawal | 4 | |
Patient localizes pain | 5 | |
Patient obeys | 6 | |
Total | 3–15 |
This standard scale quantitates the level of consciousness, with the lower scores indicating less neurologic function. Neurologists interpret scores of 3–8 as signifying severe traumatic brain injury (TBI) or coma; 9–12, moderate TBI; and 13–15, mild TBI. However, the GCS is not readily applicable to patients who have sustained cerebral hypoxia and, because they cannot make a verbal response, those who are intubated. Adapted, with kind permission, from Teasdale G, Jennett B. Assessment of coma and impaired consciousness: A practical scale. Lancet 1974;2:81–84.
*Decerebrate rigidity (Fig. 19-3).
†Decorticate rigidity (Fig. 11-5).
By the first day after TBI, of patients who score 3 on the GCS, which is the lowest possible score, 90% have a fatal outcome and most of the remaining never regain consciousness. By 4 weeks, almost all TBI comatose patients die, partially recover and regain consciousness, or evolve into the vegetative state (see Chapter 11). When in coma or the vegetative state, individuals cannot perceive pain and do not suffer.
Posttraumatic Epilepsy
Seizures that occur either within the first 24 hours (immediate posttraumatic seizures) or the first 7 days (early posttraumatic seizures) do not fall within the definition of PTE because neurologists label them “provoked seizures.” Only seizures that occur (and recur) more than 7 days after the traumatic injury fall into the definition of PTE. Of note, 1 week of phenytoin prophylaxis following severe TBI reduces early posttraumatic seizures, but no AED clearly reduces the prevalence of PTE. PTE remits in only 25–50% of cases. PTE usually takes the form of complex partial seizures that undergo secondary generalization (see Chapter 10). Not only does PTE cause disability and carry the risk of further head injury, AEDs may exacerbate TBI-induced cognitive and personality changes.
Cognitive Impairment
TBI-induced coma usually lasts, at most, 4 weeks. By then, most patients have either succumbed to their injuries or recovered at least some cognition. However, many patients remain in a twilight state with their eyes open, but unconscious. These patients are incapable of thinking, communicating, or deliberately moving. They cannot perceive pain and do not suffer. Most of them linger in the persistent vegetative state (see Chapter 11).
Surprisingly, the trauma’s location, with one important exception, correlates inconsistently with cognitive impairment. Left temporal lobe injuries, the exception, routinely produce vocabulary deficits similar to anomic aphasia (see Chapter 8).
In addition to TBI causing debilitating cognitive impairments, some epidemiologic studies suggest that it also constitutes a risk factor for Alzheimer disease. Studies have shown that severe head trauma causes increased levels of soluble amyloid and deposition of amyloid plaques, one of the hallmarks of Alzheimer disease. Several, but not all, studies also suggest that moderate and severe head trauma in individuals with two apolipoprotein E4 (Apo-E4) alleles correlates with a marked increased risk of developing Alzheimer disease (see Chapter 7). Surviving moderate TBI, individuals with two Apo-E4 alleles may have twice the risk of developing Alzheimer disease, and surviving severe TBI, those individuals may have four times the risk. (A confounding issue for some of these studies is that individuals with Alzheimer disease are prone to cause an accident in which they sustain TBI and come to medical attention.)
Treatment Strategies for Posttraumatic Cognitive Impairment
Neurologists and other physicians who work with TBI patients with cognitive impairment administer medications that hopefully increase their attentiveness, if not reverse their learning and memory impairments. Of the numerous medicines that purportedly help, few have undergone rigorous trials that have documented their value. For example, many trials have indicated that methylphenidate and other dopamine-enhancing medications increase patients’ attention and directly or indirectly memory. Anticholinesterases may also improve TBI patients’ memory, at least those who have suffered severe memory deficits (see Chapter 7). Treatment of comorbid PTSD, if present, may improve cognitive impairments and other posttraumatic neuropsychologic changes. At the same time, physicians should, if possible, reduce or eliminate medicines that may interfere with attention and memory, such as AEDs, antipsychotics, and minor tranquilizers. They should always bear in mind that multiple medicines are likely to lead to adverse interactions and unwanted, occasionally fatal, outcomes.
Other Mental Disturbances
Personality Changes
In addition to altering patients’ personality and possibly cognitive capacity, frontal lobe injury may disable their executive abilities. Just as with patients who sustain anterior cerebral artery infarctions, those who sustain frontal lobe trauma have impaired ability to solve abstract problems, plan sequenced actions, or execute responses. Moreover, patients who develop pseudobulbar palsy, another complication of frontal lobe trauma, may display emotional incontinence with easily precipitated fits of pathologic laughing or crying, and unexpected sudden switches between them (see Chapter 4).
Trauma in Childhood
When TBI occurs before growth spurts, affected limbs may fail to achieve their normal, expectable size. The limbs’ growth arrest resembles the spastic hemiparesis with foreshortened limbs that characterizes congenital cerebral injuries (see Fig. 13-4). If dominant hemisphere injury were to occur before age 5 years, the opposite hemisphere would usually assume control of language. For example, a left-sided cerebral injury in a 4-year-old child will probably not result in aphasia because the plasticity of the brain allows the right cerebral hemisphere to develop language centers. If TBI affects the dominant hemisphere of someone age 5 years or older, it is likely to cause language impairments, if not clear-cut aphasia.
Nonaccidental Head Injury
The shaken baby syndrome, which is another form of abusive head trauma, consists of the injuries inflicted by violent back-and-forth throws, without direct impact. In this violence, rotational (angular) deceleration produces diffuse axonal shearing, hemorrhages in the brain’s delicate parenchyma and subdural space, and retinal hemorrhages (Fig. 22-3).
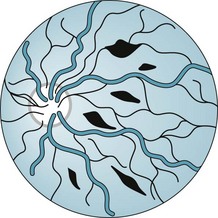
FIGURE 22-3 Head trauma causes small hemorrhages throughout the retina – not just around the disk as in papilledema.