Chapter 97 Training for Wilderness Adventure
For online-only figures, please go to www.expertconsult.com
For a couple million years, humans have survived in wilderness environments. It is only in the last few millennia that socialization has led to what we call civilization. Modern life has formed a construct for human existence that has largely overcome the need for survival competence. However, there are still populations that rely on physical capabilities and resourcefulness to survive on planet Earth, most of which is still wilderness (Figure 97-1).
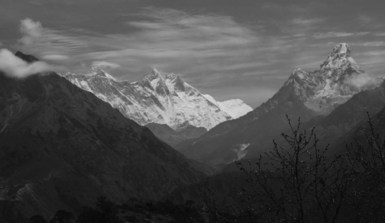
FIGURE 97-1 View in the Khumbu region of Nepal, with Mt Everest, Mt Lhotse, and Mt Ama Dablam appearing most prominent.
(Courtesy Robert B. Schoene.)
Physical Conditioning
Speed training is essential. Strength and flexibility training are covered in Chapter 98. This chapter deals primarily with aerobic fitness and exercise physiology with an emphasis on high-altitude fitness, because adaptation and exercise performance in that environment carry with them concepts universally applicable to all wilderness endeavors.
Aerobic Fitness
The concepts of aerobic fitness are similar for champion and recreational athletes. The parts of the “engine” are the same; it is the quality and fine-tuning that are different. Three essential characteristics are maximum oxygen consumption (max), lactate or anaerobic threshold, and efficiency. These factors are interrelated in a way that results in effective performance, and each is trainable. The interrelationships result in improved endurance, the most important overall factor for enjoyment and survival in the wilderness.34
Maximum Oxygen Consumption
Oxygen consumption () is defined by the Fick equation:
Cardiac output is equal to heart rate multiplied by stroke volume. Oxygen extraction is the difference between the content of oxygen of the arterial and mixed venous blood (i.e., the amount of oxygen that is used as blood traverses tissue beds). The metabolic response of exertion is limited by cardiac output and the limits of oxygen extraction, both of which can be modulated with training. The role of max and its various considerations are discussed by Levine.40
max is the fingerprint of an individual’s physiology. It is a reproducible marker of fitness in an individual that varies depending on training, altitude, and illness. The many genetic factors (i.e., polygenic) that contribute to a person’s
max make it highly unlikely that any one individual could be endowed with all of these genes.60 One’s
max is influenced by both inherited and environmental factors.8 What remains to be explained is the observation that, among sedentary subjects in family groups who were maintained on a supervised aerobic exercise program for 20 weeks, there was great variability in how much
max could be improved7 (Figure 97-2). The improvement in
max ranged from negative values to 30% improvement, and these various levels of improvement were grouped in family clusters. Further data from this series of studies looked at age, race, gender, and initial fitness and found that all subjects experienced gains in
max, but with a great deal of variability and little correlation among the aforementioned factors that contributed to those gains. It is clear that there are limits in training to improve
max. In other words, a “normal” individual with a
max of 42 cc/kg/min may be able to improve his or her
max to the high 40s cc/kg/min but will never be able to approach the 75 to 85 cc/kg/min range of high-performance middle- to long-distance athletes, who chose their parents well.
What parts of one’s aerobic capacity can be trained? Considering the Fick equation, it becomes apparent that an increase in cardiac output, improved extraction of oxygen, or both will improve max. In fact, both things happen, but it is clearly the heart that can be trained more by increasing its stroke volume and improving its muscular strength.20,25 Thus, the heart rate necessary to achieve an appropriate cardiac output for any given metabolic rate is lower in the trained state as compared with the untrained state. Although maximum heart rate does not change with training, resting and submaximal heart rates are lower and can be used as simple markers to monitor training. Although the elements of oxygen extraction somewhat improve, the heart’s stroke volume conveys increased ability to perfuse large volumes of muscle mass such that, with training, there are increased capillary and mitochondrial densities and optimization of the components of oxidative metabolism.3,24,29,30
It is fascinating to put human physiology in perspective with the rest of the animal kingdom. Normal humans in the age range of 20 to 40 years have a max somewhere around 40 cc/kg/min, and accomplished endurance athletes have one in the range of 70 to 85 cc/kg/min; alternatively, some large mammals have extraordinarily high aerobic capacities. For instance, horses have
max values that range from 134 cc/kg/min in standardbred horses2 to 160 cc/kg/min in thoroughbreds.38,43 The North American pronghorn antelope is said to have values as high as 300 cc/kg/min. Although thoroughbred horses were bred several hundred years ago to be great aerobic athletes, the antelope’s evolutionary strategy is to have exercise capabilities that optimize its chance of preserving the small family groups that live on an open plain full of predators (i.e., the pronghorn can run sustainably at 80.5 km/hr [50 mi/hr]).
Sustainable Threshold
The onset of unsustainable work intensity essentially involves a shift of fuel supply within the cell. At workloads below the LT, free fatty acids are the primary oxidative fuel. Above the LT, when the oxidative turnover of free fatty acids cannot keep up with the demand for adenosine triphosphate, glycolysis occurs. Muscle glycogen is broken down as fuel, with lactic acid being produced at a rate beyond the body’s ability to use it.33,49 Blood lactate levels correlate with intensity of work and thus are inversely correlated with the duration of a competitive event (Figure 97-3).
Improving Human Performance
Malleability of the Lactate Threshold
The ability of a muscle to sustain work is related to its oxidative capacity. This capacity is quite malleable, and depends on the level of the muscle’s activity while it is engaged.19,28 Among high-level athletes, oxidative capacity can be several-fold greater than among untrained individuals. Functionally, then, high-level athletes have inherently high max levels, and can perform sustainable work at a much higher percentage of their maximum capacity. For example, an international cyclist may have a maximum work capacity of 550 watts and be able to sustain 450 watts of work during an hour-long hill climb. This is an extraordinary level of work output. A more usual and quasi-sedentary individual may have a maximum workload of 200 watts and be able to sustain 50% to 60% of that work intensity, which is considered to be “normal.”
In highly specialized athletes such as cyclists, the muscle mass involved in the effort has been shown to be progressively recruited in a way such that the oxidative stress is balanced and shared.13,15 As much as 25% of the cyclist’s muscle mass can be spared on a rotating basis, which reduces the oxidative stress of muscle fibers, thus prolonging the onset of the LT. This phenomenon may perhaps be a way to acquire more endurance, delay fatigue, and promote efficiency. Furthermore, with a finite fuel supply, this strategy would preserve glycogen stores and delay the onset of glycolysis (and thus the production of lactate).
The crux of cellular oxidative metabolism is convection of oxygen to the cell by the circulation, diffusion of oxygen across the cell membrane into the cytosol, and then diffusion of oxygen into the mitochondria. The actual diffusion gradient necessary to get oxygen to the mitochondria is on the order of 2 to 3 mm Hg at each of these steps.52 Thus, perfusion rather than hypoxemia per se is a limiting factor. Therefore, one of the most important adaptive steps is augmenting blood flow through angiogenesis of the microcirculation. In this regard, in two studies, highly trained cyclists and triathletes with comparable values of max were exercised at 88% of their maximum aerobic capacity until fatigue.13,14 There were two patterns that showed a shorter and longer time to fatigue. The athletes with more endurance had a substantially greater capillary density than did the athletes who fatigued earlier, despite comparable maximum aerobic capacities. Because both groups were highly trained, it is not clear whether, in certain athletes, there is some inherent propensity for greater signaling of angiogenesis that comes from training. The authors speculated that this augmented perfusion may be important not only for the convective phase of oxygen but also for providing a greater volume of the effluent portion of metabolic byproducts. Another study looked at subtle factors that contribute to fatigue at very high levels of exercise and found that very small changes in energy expenditure when a person is at exercise intensities of greater than 80% of
max can lead to rapid onset of fatigue.44 Therefore, it is critical for an athlete—whether on the field or in the wilderness—to know the location of his or her “edge” so there is some reserve for optimally finishing an activity.
Training Effect on the Lactate Threshold
Much has been written since the late 1970s about plasticity of the LT. It behooves any athlete to be able to perform at the highest percentage of his or her maximum aerobic capacity. One of the first studies to look at the effect of aerobic training on the LT involved nine sedentary men who performed 9 weeks of supervised endurance training for 45 minutes per day for 4.1 days per week.16 There was a comparable untrained control group. The exercise group increased its LT by 44% expressed as absolute , and 15% expressed as
max.
max also increased 25%. Maximum work rate increased 28%, with decreases in the ventilatory equivalent seen at submaximal levels of work. The volume of work was similar in the test group, so the study did not answer the following questions (Figure 97-4): How much volume is necessary to induce these changes? If some work is good, is more or less better?
Several studies have looked at a number of variations on the IT theme and its effect on performance, LT, serum lactate, time to exhaustion, muscle physiology, and so forth. One study enrolled seven trained male distance runners and added 3 days of intense levels of training (i.e., >95% heart rate maximum) per week for 8 weeks.1