202 Thrombolytics
Thrombolytic agents comprise a diverse group of compounds that indirectly initiate lysis of thrombi. In the formation of a thrombus, fibrin forms the molecular scaffolding. After initiation of the coagulation cascade, fibrinolytic mechanisms are concomitantly activated to prevent unchecked thrombosis. The fibrinolytic process begins with cleavage of the proenzyme plasminogen to plasmin, an enzyme that hydrolyzes key bonds within the fibrin clot matrix, resulting in clot lysis (Figure 202-1). Intravenous (IV) or intraarterial thrombolytic agents function by promoting the conversion of plasminogen to plasmin. The different thrombolytic agents vary in their specificity for plasminogen, metabolic half-life, and antigenicity (Table 202-1).
Drugs
Streptokinase
Streptokinase, a protein produced by β-hemolytic streptococci, was first identified as having fibrinolytic properties in the 1930s1 and was the first compound used clinically as a thrombolytic drug.2 Despite its name, streptokinase itself is not an enzyme. Rather, it complexes with plasminogen in a 1 : 1 stoichiometric relationship. The streptokinase-plasminogen complex then converts both circulating and fibrin-bound plasminogen to plasmin. One of the major drawbacks to clinical use of streptokinase is its antigenicity, as antibodies may have formed during prior streptococcal infection. Allergic reactions occur in 2% to 5% of patients receiving the drug and are generally mild, but severe anaphylactic reactions can occur.3 It has a short half-life of approximately 20 minutes. Anistreplase (APSAC [anisoylated plasminogen streptokinase activator complex]) is a modified form of streptokinase that has a substantially longer half-life but still can cause allergic reactions (see Figure 202-1).
Urokinase
Urokinase is a thrombolytic protein that was initially isolated from human urine and has been used clinically for over 30 years. It is isolated from human fetal renal tissue cultures and, unlike streptokinase, enzymatically cleaves plasminogen. During the 1980s and early 1990s, urokinase was the primary thrombolytic agent used clinically for treatment of graft thrombosis and peripheral arterial occlusion. In 1999, urokinase was removed from the U.S. market after questions were raised by the Food and Drug Administration (FDA) regarding the safety of this product.4 It was reintroduced in the United States in late 2002 after rigorous testing showed that the preparations were free of human pathogens, but its only current indication is for pulmonary embolism. Prourokinase, also known as single-chain urokinase-type plasminogen activator (scu-PA), is a single-chain precursor molecule of urokinase that is converted into two-chain urokinase by hydrolysis. It is relatively fibrin specific and has low antigenicity.
Tissue Plasminogen Activator
Tissue plasminogen activator (tPA) was first isolated in 1981.5 It is a naturally occurring protein synthesized by human vascular endothelial cells. Commercially available preparations are manufactured using recombinant technologies, as first described by Pennica and colleagues.6 A number of different recombinant variants are available, including alteplase (rtPA, approved by the FDA in 1987) and duteplase, as well as other forms of the tissue-type plasminogen activators: reteplase (rPA), tenecteplase (TNK-tPA), and lanoteplase (nPA). Recombinant tPAs have the advantage of being nonantigenic and specific for fibrin-bound plasminogen and avoid the infectious risks associated with products isolated from cultured human tissues. Newer recombinant tPAs have improved pharmacokinetics, allowing for more convenient administration such as bolus dosing.
Clinical Indications
Myocardial Infarction
Acute myocardial infarction (AMI) represents a significant healthcare burden in industrialized countries, with trends estimating the growing impact of this disease on the world. Modern management of AMI focuses on rapidly restoring perfusion to optimize salvage of myocardium. To this end, primary percutaneous coronary interventions (PCIs) have been shown to be superior to thrombolytic therapy when employed as an early reperfusion strategy after AMI and are thus recommended as first-line therapy when available.7 However, logistical barriers exist hindering access to early PCI for all patients with AMI, whereas fibrinolysis can be used in nearly all hospitals.
The use of lytic therapy for the treatment of AMI was first attempted in the 1950s.8 The rationale for this therapy was that reestablishing coronary blood flow in an acutely thrombosed vessel would reduce infarct size and mortality. A meta-analysis of 33 randomized trials in 1985 demonstrated a 22% reduction in mortality with the use of thrombolytics in AMI, and these findings prompted further investigation into lytic therapy for MI.9 The Fibrinolytic Therapy Trialists’ Collaborative Group (FTT) performed a meta-analysis in 1994 with aggregated results from over 58,000 patients treated with thrombolytics.10 This analysis revealed a nearly 25% reduction in mortality in those patients with ST-segment elevation or bundle branch block. Numerous studies since have been performed to evaluate the efficacy of different lytic agents, dosing strategies, routes of administration, and adjunctive therapies for rapid restoration of antegrade flow in thrombosed coronary arteries.
Early studies focused on the use of streptokinase, demonstrating 18% and 25% reductions in mortality at 3 and 5 weeks, respectively, in the ISIS-23 and GISSI studies.11 These short-term findings in the fibrinolytic group were maintained out to 1- to 10-year follow-ups.12 The efficacy of tPA was studied in the GUSTO-1 trial, which examined 4 dosing regimens for the treatment of MI in 41,021 patients.13 This study utilized “accelerated” tPA dosing, wherein two-thirds of the total dose was administered in the first 30 minutes rather than over 3 hours. This dosing regimen resulted in a modest but significant reduction in 30-day mortality (6.3%) compared to streptokinase (7.4%) or a combination of tPA and streptokinase (7.0%). The GUSTO angiographic substudy demonstrated that differing patency rates between patients treated with either agent accounted for this difference in clinical efficacy. A subsequent meta-analysis of this approach, however, failed to validate the survival advantage.14 The recombinant deletion mutant of tPA, reteplase, was compared to accelerated tPA in 15,059 patients in the GUSTO III trial. No survival advantage was observed with reteplase, and rates of intracranial hemorrhage were similar (0.91% and 0.87% with reteplase and tPA, respectively).15 The ASSENT-2 trial showed that mortality at 30 days and ICH rates were identical between use of tenecteplase and accelerated tPA.16 While reteplase and tenecteplase have not surpassed tPA in terms of efficacy, their pharmacokinetics translate to simplified administration versus accelerated tPA.
Adjunctive therapies such as aspirin or clopidogrel and antithrombin agents improve the results of lytic therapy. As fibrinolysis strips fibrin from the occluding thrombus, the exposed thrombin initiates platelet aggregation and subsequent rethrombosis.17 Therefore some form of antithrombin strategy is warranted. Heparin can be infused to keep the activated partial thromboplastin time (APTT) between 50 and 70 seconds. If heparin-induced thrombocytopenia is suspected, direct thrombin inhibitors (hirudin or bivalirudin) can be used.18 Another development has been the introduction of glycoprotein IIb/IIIa receptor blockers such as abciximab (ReoPro), eptifibatide (Integrilin), and tirofiban (Aggrastat).19–21 Despite some promising early results,22 no randomized trial has yet to show an impact on mortality with combination therapy.23–25
The timing of diagnosis and institution of thrombolytic therapy is critical.26,27 Patients with AMI treated with thrombolytic agents more than 4 hours after the onset of symptoms have 30-day and 6-month mortality rates that are 2 to 3 times higher than patients who were treated within 2 hours after the onset of symptoms.28 Eighty-two percent of patients treated within 2 hours had return of normal cardiac wall motion, whereas only 46% of those treated within 2 to 5 hours after the onset of symptoms have return of normal wall motion.29 The LATE (Late Assessment of Thrombolytic Efficacy) study reported 1-year mortality rates of 17.6% versus 15.8% in those patients treated with rtPA at greater than 3 hours versus less than 3 hours, respectively, after the onset of symptoms.30 So critical is the timing of the initiation of treatment that prehospital administration of thrombolytics has been advocated in selected patients with ST-segment elevations on an electrocardiogram.31,32
Currently accepted guidelines for lytic therapy in AMI are outlined by the American College of Chest Physicians in the 2008 8th edition of the Evidence-Based Clinical Practice Guidelines and by the American College of Cardiology/American Heart Association in their 2004 guidelines.33,34 The treatment algorithm is summarized in Figure 202-2. Unfortunately, the value of thrombolytic agents for the management of unstable angina remains unproven. Currently there is no role for lytic therapy in acute coronary syndrome in the absence of ST-segment elevation in two or more contiguous leads or without new-onset bundle branch block. Contraindications to lytic therapy in the setting of AMI are also summarized in Table 202-2.
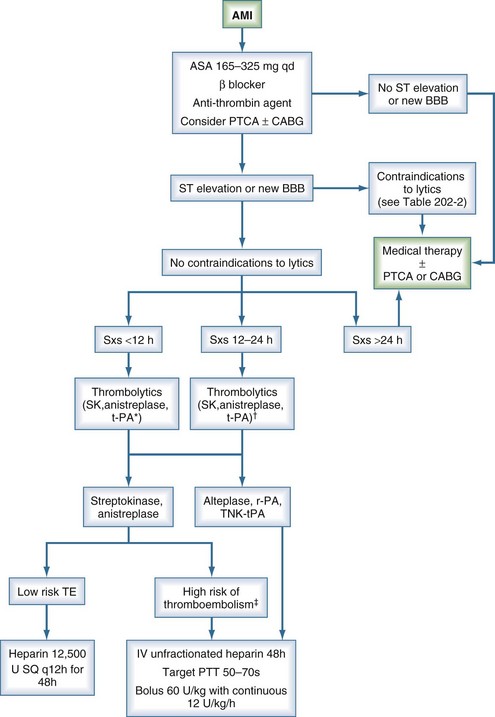
Figure 202-2 Algorithm for treatment of acute myocardial infarction. *, preferred for symptoms <6 h; †, grade 2b data11; ‡, anterior myocardial infarction, existing heart failure, previous embolus, atrial fibrillation, left ventricular thrombus; AMI, acute myocardial infarction; BBB, bundle branch block; CABG, coronary artery bypass grafting; PTCA, percutaneous transluminal coronary angioplasty; PTT, partial thromboplastin time; SK, streptokinase; SQ, subcutaneous; Sxs, signs and symptoms; TE, thromboembolism.
(Adapted from 1999/2002 ACC/AHA Guideline Update and 2001 ACCP Consensus Conference.11–12)
TABLE 202-2 Contraindications to Thrombolytic Therapy in the Setting of Acute Myocardial Infarction*
Absolute Contraindications | Relative Contraindications |
---|---|
>24 hours since onset of symptoms | 12 to 24 hours since onset of symptoms |
Prior intracranial hemorrhage | Age > 75 years |
Stroke within past year | Systolic blood pressure > 180 mm Hg or diastolic blood pressure > 110 mm Hg |
Intracranial neoplasm | Bleeding disorder |
Active bleeding/bleeding diathesis | Prior allergic reaction to thrombolytics |
Suspected aortic dissection | Pregnant or lactating |
Significant closed-head or facial trauma within 3 months | Prolonged cardiopulmonary resuscitation (>10 min) Recent internal bleeding (<2-4 wk) Active peptic ulcer |
* With ST-segment elevation and/or new bundle branch block.
Recently, a large meta-analysis was performed that examined 7739 patients with ST-segment elevation randomized to either thrombolytic agents (76% receiving fibrin-specific lytics) or primary percutaneous transluminal coronary angioplasty (PTCA).7 Short-term (4-6 weeks) mortality in the PTCA group was 7%, compared to 9% in the group that received lytic therapy (P = .0003). The group treated with primary PTCA had lower rates for nonfatal reinfarction (3% versus 7%) and stroke (1% versus 2%) as part of a follow-up to a smaller study.35 The short-term results of this meta-analysis are summarized in Figure 202-3.
Potential advantages of angioplasty over thrombolysis36–39 as primary therapy for AMI must be tempered by the recognition that angioplasty results are highly dependent on the volume of cases at a given treatment center. Moreover, comparisons to lytic therapy often use historical data before the era of accelerated dosing regimens and adjunctive treatment with antiplatelet and antithrombin agents. Lytic therapy also may be advantageous in critically ill patients who are unable to be transported to cardiac catheterization facilities or in those who have other contraindications to PTCA. Ultimately, the ideal treatment for some patients may involve combinations of angioplasty, reduced-dose thrombolytic therapy, antithrombotic agents, and antiplatelet agents.
Stroke
Stroke is the third leading cause of death in the United States, affecting over 700,000 people per year. Strokes are a major source of morbidity and mortality among hospitalized patients.40 The majority of strokes are ischemic in nature, resulting from sudden occlusion of arteries delivering blood supply to the brain. These occlusions often are caused by thromboemboli from a variety of sources.41 Traditional therapy for ischemic stroke has focused on the use of anticoagulation and antiplatelet agents for medical support and then rehabilitation after the acute event. More recently, thrombolytic therapy has emerged as a mode of intervention in ischemic stroke patients. Similar to the treatment of AMI, the efficacy of thrombolytic agents is highly time dependent owing to the characteristics of the ischemic penumbra.42 Indeed, efficacy is greater when lytic treatment is administered within 90 minutes of the event.43
The concept of utilizing “clot-busting” therapies for ischemic stroke blossomed in 1995 when the National Institute of Neurological Disorders and Stroke (NINDS) published a study on rtPA in acute ischemic stroke.44 This trial consisted of two parts. Part I enrolled 291 patients and examined the clinical efficacy of IV tPA given within 3 hours after symptom onset. This treatment failed to improve neurologic function after 24 hours versus placebo. By 3 months, however, patients treated with tPA showed significant improvement in 4 different functional outcome measurements as assessed by the National Institutes of Health Stroke Scale (NIHSS). Part II of this study assessed the long-term outcomes of tPA treatment. Patients who received tPA were 30% more likely to have minimal residual disability or to have returned to baseline functional status at 3, 6, and 12 months.45 Unfortunately, patients treated with tPA suffered a greater incidence of intracerebral hemorrhage (6.4% versus 0.6% in the placebo group; P < .001) at 36 hours. Subsequent studies have shown similar rates of intracranial hemorrhage after lytic therapy. Nevertheless, mortality at 3 months was not significantly different (17% versus 21%; P = .30). Intravenous tPA was approved by the FDA for treatment of acute ischemic stroke within a 3-hour window as a result of this study. Other early randomized trials of IV tPA in acute stroke treatment included the European Cooperative Acute Stroke Study (ECASS-I),46 ECASS-II,47 and the ATLANTIS trials.42,48 These trials, while each failing to reach significance for their primary outcome measure, did show significant benefit in favor of tPA usage between 0 and 6 hours for alternative outcome measures, lending some additional support for its use. A subsequent analysis of these trials showed that the odds ratio of benefit of IV tPA decreased as time from stroke onset increased.49
More recently, the ECASS-III trial did demonstrate a lesser, albeit significant, benefit of tPA compared to placebo when administered in the 3- to 4.5-hour window, with no difference in mortality.50 Rates of symptomatic intracranial hemorrhage (based on the NINDS definition) were slightly higher than in the NINDS trial (7.9% versus 3.5% in the placebo group; P = 0.006). It is important to note that this trial exercised more stringent inclusion criteria than the NINDS trial. Based on the results of this trial, Lansberg et al. calculated that the number of patients deriving benefit per 100 treated are 28, 23, and 17 for 0- to 1.5-hour, 1.5- to 3-hour, and 3- to 4.5-hour windows, respectively.51 It is estimated that in the 3- to 4.5-hour window, 1 in 6 patients will have a better outcome and 1 in 35 a worse outcome.52 As a result of the ECASS III trial, IV tPA usage for treatment of stroke has been supported by the Scientific Advisory from the American Heart Association Stroke Council, although the FDA has not expanded its approval to date.53
Currently, the only drug and route of administration currently approved by the FDA for treatment of ischemic stroke is tPA through an IV route. However, prourokinase as well as intraarterial fibrinolytic administration are also used in the setting of established clinical protocols. Intravenous delivery has both practical and theoretical disadvantages. One disadvantage is the inability to effectively lyse the internal carotid artery or the middle cerebral artery.54 After confirmation by head computed tomography (CT) of ischemic stroke (i.e., not associated with hemorrhage), patients are typically treated by IV tPA at a dose of 0.9 mg/kg, with 10% of the total dose given as an initial bolus, and the remainder infused over 60 minutes.55
Intraarterial administration of thrombolytics is gaining popularity for treating ischemic stroke. This method requires that a neurointerventionalist obtain arterial images and place an intraarterial catheter into the thrombosed vessel. The thrombolytic agent is then infused through the catheter directly into the target vessel, achieving high local concentration at the site of occlusion while decreasing systemic drug levels. Direct intraarterial delivery has been shown to be effective in limited studies. The Prolyse in Acute Cerebral Thromboembolism (PROACT II) study56 randomized 180 patients with middle cerebral artery occlusion to either intraarterial prourokinase plus heparin or heparin alone. Intraarterial prourokinase improved the modified Rankin score to 2 or less in over 40% of patients, whereas heparin infusion alone improved the score in only 25%. Also, prourokinase was associated with significantly higher vessel recanalization rates (66% versus 18%; P < .0001). The MELT trial was a Japanese trial of intraarterial urokinase which was halted early due to external reasons and was thus underpowered to reach significance of the primary endpoint.57 Nevertheless, secondary analysis from that study and combined analyses with the PROACT trials suggested benefit to arterial urokinase.58 Prourokinase is not available for general use, but tPA and its variants are frequently administered in intraarterial fashion.59,60
Additionally, the Interventional Management of Stroke (IMS) trialists recently began a phase III trial comparing standard-dose IV tPA with a reduced-dose IV tPA bridge to an endovascular treatment consisting of either mechanical thrombectomy, arterial tPA infusion, or a combination of low-intensity ultrasound with tPA infusion.61 Although growing evidence supports use of intraarterial revascularization methods, they are obviously restricted to those centers with skilled neurointerventionalists. Standard contraindications to IV thrombolytic therapy in acute ischemic stroke are similar to the exclusion criteria used in the NINDS study (Table 202-3). Blood pressure should be tightly controlled, ideally maintained below 180/105 mm Hg. Antithrombotic agents should also be withheld for 24 hours owing to the risk of intracranial hemorrhage. A number of adjunctive therapies including mechanical thrombectomy,62 glycoprotein IIb/IIIa inhibitors,63 and ultrasound64,65 have shown promise in improving recanalization rates.
TABLE 202-3 Contraindications for Thrombolytic Therapy in Ischemic Stroke
Contraindications | Relative Contraindications |
---|---|
Symptom duration >6 hours | Symptom duration of 3 to 6 hours |
History of intracranial hemorrhage | Witnessed seizure |
Evidence of active bleeding | Gastrointestinal or urinary hemorrhage within 3 weeks |
Platelet count <100,000/mm3 | Recent lumbar puncture, noncompressible arterial puncture site |
Prior stroke, head trauma, or intracranial surgery within 3 months | Systolic blood pressure >185 mm Hg or diastolic blood pressure >110 mm Hg |
Rapidly improving or only minor symptoms | Mass effect or hypodensity of greater than one-third middle cerebral artery distribution on head CT |
Major surgery within 14 days | |
Known arteriovenous malformation or intracranial aneurysm | Glucose <50 mg/dL or >400 mg/dL |
Elevated partial thromboplastin time or international normalized ratio (>1.7) |
Pulmonary Embolism
Pulmonary embolism (PE) is not only a major source of morbidity and mortality in hospitalized patients, accounting for up to 15% of in-hospital deaths, but is also a surprisingly underrecognized source of cardiovascular collapse.66–68 Anticoagulation has been a critical component in the treatment of PE since first shown to be beneficial in 1960.69 This may be achieved using IV unfractionated heparin (UFH), with an initial bolus of 80 units/kg followed by 18 units/kg/h, with monitored dose adjustment to maintain the APTT prolongation at a level that corresponds to 0.3 to 0.7 IU/mL anti-Xa activity. Alternatively, subcutaneous (SQ) low-molecular-weight heparin (LMWH), SQ fondaparinux, or monitored or fixed-dose SQ UFH may be used.70 Unfortunately, despite the proven efficacy of anticoagulation in the setting of acute PE, a significant proportion of patients will have incomplete resolution of their occlusion, with subsequent organization of the thrombus and obliteration of the pulmonary artery.71–73 Accordingly, thrombolytic therapy for pulmonary embolism may offer more rapid and complete resolution of thrombus burden.
One of the initial studies that evaluated thrombolytic therapy for PE was the Urokinase Pulmonary Embolism Trial (UPET).71 This prospective trial randomized 160 patients to either urokinase followed by heparin or heparin alone. Although transient hemodynamic improvement was achieved, no differences were evident with regard to mortality or perfusion scan past 5 days. Nonetheless, the UPET and the subsequent Urokinase-Streptokinase Embolism Trial (USET) demonstrated improvements in small vessel patency at 2 weeks and 1 year compared with anticoagulation alone.74–75 Seven-year follow-up of this cohort of patients suggested the risk of pulmonary hypertension was decreased by thrombolysis, presumably because lytic therapy achieved superior clot dissolution and decreased the risk of subsequent pulmonary embolism.76