CHAPTER 39 The orbit and accessory visual apparatus
BONY ORBIT
Each cavity approximates to a quadrilateral pyramid with its base at the orbital opening, narrowing to its apex along a posteromedially directed axis. Each orbit has a roof, floor, medial and lateral walls. The medial walls lie approximately 25 mm apart in adults and are nearly parallel. The angle between the medial and lateral walls is about 45°. The compromise between protection and ensuring a good field of view dictates that each eyeball is located anteriorly within the orbit. The eyeball thus occupies only one fifth of the volume of the orbit (Fig. 39.1): the remainder of the cavity is filled with vessels and nerves that are contained within and supported by orbital fat and connective tissue. In brief, the orbit also contains the extraocular muscles; the optic, oculomotor, trochlear and abducent nerves, and branches of the ophthalmic and maxillary divisions of the trigeminal nerve; the ciliary parasympathetic ganglion; the ophthalmic vessels; the nasolacrimal apparatus.
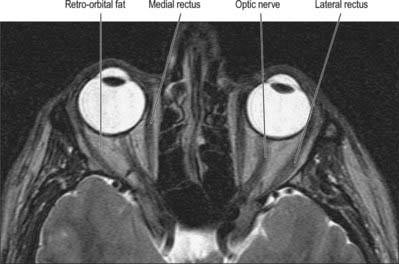
Fig. 39.1 T2-weighted axial MRI scan though the mid-orbit.
(By courtesy of Dr Timothy Beale FRCR, Royal National Throat Nose and Ear Hospital, London.)
Roof
The roof of the orbit is formed principally by the thin orbital plate of the frontal bone (Fig. 39.2). It is gently concave on its orbital aspect, which separates the orbital contents and the brain in the anterior cranial fossa. Anteromedially it contains the frontal sinus and displays a small trochlear fovea, sometimes surmounted by a small spine, where the cartilaginous trochlea (pulley) for superior oblique is attached. Anterolaterally there is a shallow lacrimal fossa which houses the orbital part of the lacrimal gland. The roof slopes significantly towards the apex, joining the lesser wing of the sphenoid, which completes the roof. The optic canal lies between the roots of the lesser wing, and is bounded medially by the body of the sphenoid.
Medial wall
The medial wall of the orbit is formed principally by the orbital plate (lamina papyracea) of the ethmoid bone (Fig. 39.2). This paper thin, rectangular plate covers the middle and posterior ethmoidal air cells, providing a route by which infection can spread into the orbit (see Ch. 32). The ethmoid articulates with the medial edge of the orbital plate of the frontal bone at a suture which is interrupted by anterior and posterior ethmoidal foramina. Posteriorly, it articulates with the body of the sphenoid, which forms the medial wall of the orbit to its apex. The lacrimal bone lies anterior to the ethmoid: it contains a fossa for the nasolacrimal sac that is limited in front by the anterior lacrimal crest on the frontal process of the maxilla and behind by the posterior lacrimal crest of the lacrimal bone (to which the lacrimal part of orbicularis oculi and lacrimal fascia are attached). A descending process of the lacrimal bone at the lower end of the posterior lacrimal crest contributes to the formation of the upper part of the nasolacrimal canal, which is completed by the maxilla (Fig. 39.3).
Floor
The floor of the orbit is mostly formed by the orbital plate of the maxilla which articulates with the zygomatic bone anterolaterally and the small triangular orbital process of the palatine bone posteromedially (Fig. 39.3). The floor is thin and largely roofs the maxillary sinus. Not quite horizontal, it ascends a little laterally. Anteriorly it curves into the lateral wall, and posteriorly it is separated from the lateral wall by the inferior orbital fissure, which connects the orbit posteriorly to the pterygopalatine fossa, and more anteriorly to the infratemporal fossa. The medial lip is notched by the infraorbital groove. The latter passes forwards and sinks into the floor to become the infraorbital canal, which opens on the face at the infraorbital foramen: the infraorbital groove, canal and foramen contain the infraorbital nerve and vessels. Proportionally more orbital fractures involve the floor, particularly in the region of the infraorbital groove. The classic ‘blowout fracture’ leaves the orbital rim intact and typically entraps soft tissue structures, leading to diplopia, impaired ocular motility and enophthalmos; infra-orbital nerve involvement leads to ipsilateral sensory disturbance of the skin of the mid face.
Lateral wall
The lateral wall of the orbit is formed by the orbital surface of the greater wing of the sphenoid posteriorly and the frontal process of the zygomatic bone anteriorly: the bones meet at the sphenozygomatic suture (Fig. 39.2). The zygomatic surface contains the openings of minute canals for the zygomaticofacial and zygomaticotemporal nerves, the former near the junction of the floor and lateral wall, the latter at a slightly higher level, sometimes near the suture. The orbital tubercle, to which the lateral palpebral ligament, the check ligament of lateral rectus and the aponeurosis of levator palpebrae are all attached, lies just inside the midpoint of the lateral orbital margin. The lateral wall is the thickest wall of the orbit, especially posteriorly where it separates the orbit from the middle cranial fossa. Anteriorly the lateral wall separates the orbit and the infratemporal fossa. The lateral wall and roof are continuous anteriorly but are separated posteriorly by the superior orbital fissure, which lies between the greater wing (below) and lesser wing (above) of the sphenoid, and communicates with the middle cranial fossa. The fissure tapers laterally but widens at its medial end, its long axis descending posteromedially. Where the fissure begins to widen, its inferolateral edge shows a projection, often a spine, for the lateral attachment of the common tendinous ring. An infraorbital sulcus which runs from the superolateral end of the superior orbital fissure towards the orbital floor is sometimes associated with an anastomosis between the middle meningeal and infraorbital arteries.
ORBITAL FISSURES AND FORAMINA
Optic canal
The lesser wing of the sphenoid is connected to the body of the sphenoid by a thin, flat anterior root and a thick, triangular posterior root. The optic canal lies between these roots (Fig. 39.2) and connects the orbit to the middle cranial fossa, transmitting the optic nerve and its meningeal sheaths, and the ophthalmic artery. The common tendinous ring, which gives origin to the four recti, is attached to the bone near the superior, medial and lower margins of the orbital opening of the canal.
Superior orbital fissure
The superior orbital fissure is the gap between the greater and lesser wings of the sphenoid, bounded medially by the body of the sphenoid, and closed at its anterior extremity by the frontal bone (Fig. 39.2). It connects the cranial cavity with the orbit and transmits the oculomotor, trochlear and abducens nerves, branches of the ophthalmic nerve and the ophthalmic veins.
Inferior orbital fissure
The inferior orbital fissure is bounded above by the greater wing of the sphenoid, below by the maxilla and the orbital process of the palatine bone, and laterally by the zygomatic bone (Fig. 39.2). The maxilla and sphenoid often meet at the anterior end of the fissure, excluding the zygomatic bone. The inferior orbital fissure connects the orbit with the pterygopalatine and infratemporal fossae and transmits the infraorbital and zygomatic branches of the maxillary nerve and accompanying vessels, orbital rami from the pterygopalatine ganglion and a connection between the inferior ophthalmic vein and pterygoid venous plexus. A small maxillary depression may mark the attachment of inferior oblique anteromedially, lateral to the lacrimal hamulus.
Ethmoidal foramina
The anterior and posterior ethmoidal foramina usually lie in the frontoethmoidal suture (Fig. 39.2). The posterior foramen may be absent, and occasionally there is a middle ethmoidal foramen. The foramina open into canals which transmit their vessels and nerves into the ethmoidal sinuses, anterior cranial fossa and nasal cavity.
COMMON TENDINOUS RING
The common tendinous ring is a fibrous ring which surrounds the optic canal and part of the superior orbital fissure at the apex of the orbit, and gives origin to the four recti (Fig. 39.4). The optic nerve and ophthalmic artery enter the orbit via the optic canal, and so lie within the common tendinous ring. The superior and inferior divisions of the oculomotor nerve, the nasociliary branch of the ophthalmic nerve, and the abducens nerve, also enter the orbit within the common tendinous ring, but they do so via the superior orbital fissure (see Fig. 39.15). The trochlear nerve and the frontal and lacrimal branches of the ophthalmic nerve all enter the orbit through the superior orbital fissure but lie outside the common tendinous ring. Structures which enter the orbit through the inferior orbital fissure lie outside the common tendinous ring. The close anatomical relationship of the optic nerve and other cranial nerves at the orbital apex means that lesions in this region may lead to a combination of visual loss from optic neuropathy and ophthalmoplegia from multiple cranial nerve involvement.
ORBITAL CONNECTIVE TISSUE AND FAT
The orbit contains a complex arrangement of connective tissue which forms a supporting framework for the eyeball and also acts to limit ocular rotations and compartmentalize orbital fat (Fig. 39.5). Certain regions have anatomical and clinical significance, including the orbital septum, fascial sheath of the eye, ‘check’ ligaments, suspensory ligament and periosteum.
ORBITAL SEPTUM
The orbital septum is a weak membranous sheet, attached to the orbital rim where it becomes continuous with the periosteum (Fig. 39.5). It passes inwards into each eyelid and blends with the tarsal plates, and, in the upper eyelid, with the superficial lamella of levator palpebrae superioris. The orbital septum is thickest laterally, where it lies in front of the lateral palpebral ligament. It passes behind the medial palpebral ligament and nasolacrimal sac, but in front of the pulley of superior oblique. The septum is pierced above by levator palpebrae superioris and below by a fibrous extension from the sheaths of inferior rectus and inferior oblique. The lacrimal, supratrochlear, infratrochlear and supraorbital nerves and vessels pass through the septum from the orbit en route to the face and scalp.
FASCIAL SHEATH OF THE EYEBALL
A thin fascial sheath, the fascia bulbi (Tenon’s capsule), envelops the eyeball from the optic nerve to the corneoscleral junction, separating it from the orbital fat, and forming a socket for the eyeball (Figs 39.5, 39.6). The ocular aspect of the sheath is loosely attached to the sclera by delicate bands of episcleral connective tissue. Posteriorly, it is traversed by ciliary vessels and nerves. It fuses with the sclera and with the sheath of the optic nerve where the latter enters the eyeball: attachment to the sclera is strongest in this position and again anteriorly, just behind the corneoscleral junction at the limbus. The fascia bulbi is perforated by the tendons of the extraocular muscles and is reflected on to each as a tubular sheath called the muscular fascia. The sheath of superior oblique reaches the fibrous pulley (trochlea) associated with the muscle. The sheaths of the four recti are very thick anteriorly but are reduced posteriorly to a delicate perimysium. Just before they blend with the fascia bulbi, the thick sheaths of adjacent recti become confluent and form a fascial ring.
Expansions from the muscular fascia are important for the attachments they make. Those from the medial and lateral recti are triangular and strong, and are attached to the lacrimal and zygomatic bones respectively: since they may limit the actions of the two recti, they are termed the medial and lateral check ligaments (Fig. 39.6). Other extraocular muscles have less substantial check ligaments, and the capacity of any of them to actually limit movement has been questioned.
The sheath of inferior rectus is thickened on its underside and blends with the sheath of inferior oblique. These two, in turn, are continuous with the fascial ring noted earlier and therefore with the sheaths of the medial and lateral recti. Since the latter are attached to the orbital walls by check ligaments, a continuous fascial band, the suspensory ligament of the eye, is slung like a hammock below the eye, providing sufficient support such that, even when the maxilla (forming the floor of the orbit) is removed, the eye will retain its position.
ORBITAL FAT
The spaces between the main structures of the orbit are occupied by fat, particularly in the region between the optic nerve and the surrounding cone of muscles (Figs 39.5, 39.7). Fat also lies between the muscles and periosteum and is limited anteriorly by the orbital septum. Collectively, the fat helps to stabilize the position of the eyeball and also acts as a socket within which the eye can rotate. Conditions resulting in an increased overall volume of orbital fat, e.g. hyperthyroidism (Graves’ disease), may lead to forward protrusion of the eyeball (exophthalmos).
EXTRAOCULAR MUSCLES
LEVATOR PALPEBRAE SUPERIORIS
Levator palpebrae superioris is a thin, triangular muscle which arises from the inferior aspect of the lesser wing of the sphenoid, above and in front of the optic canal, and separated from it by the attachment of superior rectus (Fig. 39.5). It has a short narrow tendon at its posterior attachment, and broadens gradually, then more sharply as it passes anteriorly above the eyeball. The muscle ends in front in a wide aponeurosis. Some of its tendinous fibres pass straight into the upper eyelid to attach to the anterior surface of the tarsus, while the rest radiate and pierce orbicularis oculi to pass to the skin of the upper eyelid. A thin lamina of smooth muscle, the superior tarsal muscle, passes from the underside of levator palpebrae superioris to the upper margin of the superior tarsus.
The connective tissue sheaths of the adjoining surfaces of levator palpebrae superioris and superior rectus are fused (Fig. 39.5). Where the two muscles separate to reach their anterior attachments, the fascia between them forms a thick mass to which the superior conjunctival fornix is attached: this is usually described as an additional attachment of levator palpebrae superioris. Traced laterally, the aponeurosis of the levator passes between the orbital and palpebral parts of the lacrimal gland to attach to the orbital tubercle of the zygomatic bone. Traced medially, it loses its tendinous nature as it passes closely over the reflected tendon of superior oblique, and continues on to the medial palpebral ligament as loose strands of connective tissue.
THE FOUR RECTI
The four recti are approximately strap-shaped; each has a thickened middle part which thins gradually to a tendon (Figs 39.8, 39.9). They are attached posteriorly to a common tendinous ring that encircles the superior, medial and inferior margins of the optic canal, continues laterally across the inferior and medial parts of the superior orbital fissure, and is attached to a tubercle or spine on the margin of the greater wing of the sphenoid (Fig. 39.4). The tendinous ring is closely adherent to the dural sheath of the optic nerve medially and to the surrounding periosteum. Inferior rectus, part of medial rectus and the lower fibres of lateral rectus, are all attached to the lower part of the ring, whereas superior rectus, part of medial rectus and the upper fibres of lateral rectus are all attached to the upper part. A second small tendinous slip of lateral rectus is attached to the orbital surface of the greater wing of the sphenoid, lateral to the common tendinous ring.
Each rectus muscle passes forwards, in the position implied by its name, to be attached anteriorly by a tendinous expansion into the sclera, posterior to the margin of the cornea.
Superior rectus
Superior rectus is slightly larger than the other rectus muscles. It arises from the upper part of the common tendinous ring, above and lateral to the optic canal. Some fibres also arise from the dural sheath of the optic nerve. The fibres pass forwards and laterally (at an angle of approximately 25° to the median plane of the eye in the primary position) to insert into the upper part of the sclera, approximately 8 mm from the limbus (Fig. 39.8). The insertion is slightly oblique, the medial margin more anterior than the lateral margin.
Superior rectus moves the eye so that the cornea is directed upwards (elevation) and medially (adduction). To obtain upward movement alone, the muscle must function with inferior oblique. Superior rectus also causes intorsion of the eye (i.e. medial rotation). Because a check ligament extends from the muscle to levator palpebrae superioris, elevation of the eye also results in elevation of the upper eyelid. For more detailed discussion of its actions, see p. 661.
Inferior rectus
Inferior rectus arises from the common tendinous ring, below the optic canal. It runs along the orbital floor in a similar direction to superior rectus (i.e. forwards and laterally) and inserts obliquely into the sclera below the cornea, approximately 6.5 mm from the limbus (Fig. 39.9).
The principal activity of inferior rectus is to move the eye so that it is directed downwards (depression). It also causes the eye to deviate medially, and extorts the eye (i.e. produces lateral rotation). To obtain downward movement alone, inferior rectus must function with superior oblique. A fibrous extension from inferior rectus to the inferior tarsal plate of the eyelid causes the lower eyelid to be depressed when the muscle contracts. For more detailed discussion of its actions, see p. 661.
Medial rectus
Medial rectus is slightly shorter than the other recti, but is the strongest of the group. It arises from the medial part of the common tendinous ring, and also from the dural sheath of the optic nerve, passing horizontally forwards along the medial wall of the orbit, below superior oblique (Fig. 39.8). It inserts into the medial surface of the sclera, approximately 5.5 mm from the limbus and slightly anterior to the other recti.
Lateral rectus
Lateral rectus arises principally from the lateral part of the common tendinous ring and bridges the superior orbital fissure; some fibres also arise from a spine on the greater wing of the sphenoid. The muscle passes horizontally forward along the lateral wall of the orbit to insert into the lateral surface of the sclera, approximately 7 mm from the limbus (Fig. 39.8).
THE OBLIQUES
Superior oblique
Superior oblique is a fusiform muscle which arises from the body of the sphenoid superomedial to the optic canal and the tendinous attachment of the superior rectus. It passes forwards to end in a round tendon which plays through a fibrocartilaginous loop, the trochlea, attached to the trochlear fossa of the frontal bone (Fig. 39.8). Tendon and trochlea are separated by a delicate synovial sheath. The tendon subsequently descends posterolaterally and inferior to superior rectus, and is attached to the sclera in the superolateral part of the posterior quadrant behind the equator, between the superior and lateral recti.
Superior oblique is inserted into the posterior part of the eyeball; when it contracts, the back of the eyeball is elevated, and the front of the eyeball is depressed (particularly in the adducted position). Superior oblique also moves the eye laterally (abducted) and intorts the eyeball. For more detailed discussion of its actions, see p. 695.
Inferior oblique
Inferior oblique is a thin, narrow muscle that lies near the anterior margin of the floor of the orbit. It arises from the orbital surface of the maxilla lateral to the nasolacrimal dossa and ascends posterolaterally, at first between inferior rectus and the orbital floor, and then between the eyeball and lateral rectus. It is inserted into the lateral part of the sclera behind the equator of the eyeball, in the inferolateral part of the posterior quadrant between the inferior and lateral recti, near, but slightly posterior to, the attachment of superior oblique (Fig. 39.9). The muscle broadens and thins, and, in contrast to the other extraocular muscles, its tendon is barely discernible at its scleral attachment.
Inferior oblique is inserted into the posterior part of the eyeball; when it contracts, the back of the eyeball is depressed, and the front of the eyeball is elevated (particularly in the adducted position). The muscle moves the eye laterally (abduction) and also causes extorsion. For more detailed discussion of its actions, see p. 661.
MINOR MUSCLES OF THE EYELIDS
Several smooth muscles are associated with the orbit, although they are not directly attached to the eyeball. Orbitalis, the orbital muscle of Müller, lies at the back of the orbit and spans the infraorbital fissure. Its functions are uncertain, but its contraction may possibly produce a slight forward protrusion of the eyeball. The superior and inferior tarsal muscles are small muscle laminae inserted into the upper and lower eyelids and are described in more detail with the tarsal plates (p. 670). All three minor muscles receive a sympathetic innervation from the superior cervical ganglion via the internal carotid plexus.
MOVEMENTS OF THE EYES
Movements of the eyes involve rotations around a centre of rotation within the globe. For practical purposes this can be considered to lie 13.5 mm behind the corneal apex. Normal eye movements are binocular. Movements of the eyes in the same direction are termed versions, whilst those in opposite directions are termed vergences. Eye movements are often accompanied by corresponding movements of the eyelids, particularly in upgaze where the activity of levator palpebrae superioris is closely coupled to that of superior rectus. The following section describes the ocular motor system in terms of the actions of individual extraocular muscles, the diversity of eye movements and their neural control.
Actions of the extraocular muscles
The six extraocular muscles all rotate the eyeball in directions dependent on the geometrical relation between their bony and global attachments (Fig. 39.10), which are altered by the ocular movements themselves. For convenience, each muscle will be considered in isolation, but it must be appreciated that any movement of the eyeball alters the tension and/or length in all six muscles. It is useful to consider the four recti and two obliques as separate groups, (remembering always that they act in concert), because they form more obvious groupings as antagonists or synergists. The extrinsic ocular muscles collectively position the eyeball in the orbital cavity and prevent its anteroposterior movements, other than a slight retraction during blinks, because the recti exert a posterior traction while the obliques pull the eyeball to some degree anteriorly. They may be assisted by various ‘check ligaments’ (see above). A simplified description of the actions of the extraocular muscles is summarized in Figure 39.11.
The medial and lateral recti do not rotate the eye around its horizontal axis and so cannot elevate or depress the visual axes as gaze is transferred from nearer to more distant objects or the reverse. This movement requires the superior and inferior recti (aided by the two oblique muscles). It must be remembered that the orbital axis does not correspond with the visual axis in its primary position but diverges from it at an angle of approximately 23° (the value varies between individuals, and depends on the angle between the orbital axes and the median plane). Thus, the simple rotation caused by an isolated superior rectus, analysed with reference to the three hypothetical ocular axes, appears more complex, being primarily elevation (horizontal axis), and secondarily a less powerful medial rotation (vertical axis) and slight intorsion (anteroposterior axis) in which the midpoint of the upper rim of the cornea (often referred to as ‘12 o’clock’) is rotated medially towards the nose. These actions, compounded as a single, simple rotation, are easily appreciated when it is seen that the direction of traction of superior rectus runs in a posteromedial direction from its attachment in front, which is anterior to the equator and superior to the cornea, to its bony attachment near the orbital apex (Fig. 39.10). Inferior rectus pulls in a similar direction to superior rectus, but rotates the visual axis downwards about the horizontal axis. It rotates the eye medially on a vertical axis but its action around the anteroposterior axis extorts the eye, i.e. rotates it so that the corneal ‘12 o’clock’ point turns laterally. The combined, equal contractions of the superior and inferior recti therefore rotate the eyeball medially, since their effects around the horizontal and anteroposterior axes are opposed. In binocular movements they assist the medial recti in converging the visual axes, and by reciprocal adjustment can elevate or depress the visual axes. As the eyeball is rotated laterally, the lines of traction of the superior and inferior recti approach the plane of the anteroposterior ocular axis (Fig. 39.10), and so their rotational effects about this and the vertical ocular axis diminish. In abduction to approximately 23°, they become almost purely an elevator and depressor respectively of the visual axis.
Superior oblique acts on the eye from the trochlea, and, since the attachment of inferior oblique is for practical purposes vertically below this, both muscles approach the eyeball at the same angle, being attached in approximately similar positions in the superior and inferior posterolateral ocular quadrants (Fig. 39.10). Superior oblique elevates the posterior aspect of the eyeball, and inferior oblique depresses it, which means that the former rotates the visual axis downwards and the latter rotates it upwards, and both movements occur around the horizontal axis. When the eye is in the primary position, the obliquity of both muscles means that they pull in a direction posterior to the vertical axis and both therefore rotate the eye laterally around this axis. With regard to the anteroposterior axis, in isolation, superior oblique intorts the eye and inferior oblique extorts it. Like the superior and inferior recti, therefore, the two obliques have a common turning movement around the vertical axis but are opposed forces in respect of the other two. Acting in concert they could therefore assist the lateral rectus in abducting the visual axis, as in divergence of the eyes in transferring attention from near to far. Again, like the superior and inferior recti, the directions of traction of the oblique muscles also vary with ocular position, such that they become more nearly a pure elevator and a depressor as the eye is adducted.
Movements that shift or stabilize gaze
The vestibular apparatus induces a variety of reflex eye movements to compensate for the potentially disruptive effects on vision caused by head and body movement (see Ch. 37). Receptors in the semicircular canals respond to active or passive rotational (angular) accelerations of the head. When the body makes substantial rotational movements a vestibulo-ocular reflex generates a cycle of responses involving both the shifting and stabilizing of gaze. Body rotation is matched by counter-rotation of the eyes so that gaze direction is unaltered and clear vision is maintained. Physical constraint limits the rotation to 30° or less and is followed by a rapid saccadic movement of the eyes to another object in the visual scene and the cycle is repeated. Vision is therefore clear throughout most of the cycle while the image is stationary, but at the cost of no useful vision during the brief periods of the saccades. The reflex is efficient and rapid: this speed could not be generated by the visual system, which is slow relative to the short latency of vestibular receptors.
Neural control of gaze
Although the detailed anatomical substrates for the different types of eye movement differ, they share common neural circuitry which lies mainly in the pons and midbrain, for horizontal and vertical gaze movements respectively (Fig. 39.12). The common element for all types of horizontal gaze movements is the abducens nucleus. It contains motor neurones which innervate the ipsilateral lateral rectus and interneurones which project via the medial longitudinal fasciculus (MLF) to the contralateral oculomotor nucleus which controls medial rectus. A lesion of the abducens nucleus leads to a total loss of ipsilateral horizontal conjugate gaze. A lesion of the MLF produces slowed or absent adduction of the ipsilateral eye, usually associated with jerky movements (nystagmus) of the abducting eye, a syndrome called internuclear ophthalmoplegia. The gaze motor command involves specialized areas of the reticular formation of the brain stem which receive a variety of supranuclear inputs. The main region for horizontal gaze is the paramedian pontine reticular formation (PPRF), located on each side of the midline in the central paramedian part of the tegmentum, and extending from the pontomedullary junction to the pontopeduncular junction. Each PPRF contains excitatory neurones (referred to as ‘burst’ cells) which discharge at high frequencies just prior to and during ipsilateral saccades. Pause neurones, located in a midline caudal pontine nucleus called the nucleus raphe interpositus, discharge tonically except just before and during saccades. They appear to exert an inhibitory influence on the burst neurones and so prevent extraneous saccades occurring during fixation.
The vestibular nuclei and the perihypoglossal complex (especially the nucleus prepositus hypoglossi) project directly to the abducens nuclei. These projections probably carry both smooth pursuit signals, via the cerebellum, and vestibular signals. In addition, these nuclei, via reciprocal innervation with the PPRF, contain integrator neurones which control the step change in innervation required to maintain the eccentric position of the eye against the viscoelastic forces in the orbit. These forces tend to move the eyeball back to the position of looking straight ahead, i.e. the primary position, after a saccade.
The cerebellum plays an important role in the control of eye movements (see Ch. 20). The vestibulo-cerebellum (floculus and nodule) is involved in gaze holding, smooth pursuit and the vestibulo-ocular reflex. The dorsal vermis and fastigial nucleus play a major role in programming accurate saccades and smooth pursuit.
The cerebral hemispheres are extremely important for the programming and coordination of both saccadic and pursuit conjugate eye movements (see Ch. 23). There appear to be four main cortical areas in the cerebral hemispheres involved in the generation of saccades. These are the frontal eye field (FEF), which lies laterally at the caudal end of the second frontal gyrus in the premotor cortex (Brodmann area 8); the supplementary eye field (SEF), which lies at the anterior region of the supplementary motor area in the first frontal gyrus (Brodmann area 6); the dorsolateral prefrontal cortex (DLPFC), which lies anterior to the FEF in the second frontal gyrus (Brodmann area 46); and a posterior eye field (PEF), which lies in the parietal lobe, possibly in the superior part of the angular gyrus (Brodmann area 39), and the adjacent lateral intraparietal sulcus. These areas all appear to be interconnected with each other and to send projections to the superior colliculus and the brain stem areas controlling saccades.
Both area MST and FEF send direct projections to a group of nuclei which lie in the basal part of the pons. In the monkey, the dorsolateral and lateral groups of pontine nuclei receive direct cortical inputs related to smooth pursuit. Lesions of similarly located nuclei in man result in abnormal pursuit. These nuclei transfer the pursuit signal bilaterally to the posterior vermis, contralateral flocculus and fastigial nuclei of the cerebellum (see Ch. 20). The pursuit signal ultimately passes from the cerebellum to the brain stem, specifically to the medial vestibular nucleus and nucleus propositus hypoglossi, thence to the PPRF and possibly directly to the ocular motor nuclei. This circuitry therefore involves a double decussation, firstly at the level of the midpons (ponto-cerebellar neurones), and secondly in the lower pons (vestibuloabducens neurones).
VASCULAR SUPPLY AND LYMPHATIC DRAINAGE
ARTERIES
The main vessel supplying orbital structures is the ophthalmic artery (Fig. 39.13). Its terminal branches anastomose on the face and scalp with those of the facial, maxillary and superficial temporal arteries, thereby establishing connections between the external and internal carotid arteries. The infraorbital branch of the maxillary artery, and possibly the recurrent meningeal artery, also supply orbital structures.
Ophthalmic artery
Central retinal artery
The small central artery of the retina is the first branch of the ophthalmic artery. It begins below the optic nerve and for a short distance lies in the dural sheath of the nerve. It enters the inferomedial surface of the nerve approximately 1.2 cm behind the eye, and runs to the retina (accompanying the central retinal vein) along its axis. The further distribution of the central retinal artery is described on p. 693.
Ciliary arteries
The ciliary arteries are distributed in long and short posterior, and anterior groups. Long posterior ciliary arteries, usually two, pierce the sclera near the optic nerve, pass anteriorly along the horizontal meridian and join the major arterial circle of the iris (see Fig. 40.10). About seven short posterior ciliary arteries pass close to the optic nerve to reach the eyeball where they divide into 15–20 branches. They pierce the sclera around the optic nerve to supply the choroid, and anastomose with twigs of the central retinal artery at the optic disc (see Fig. 40.31). Anterior ciliary arteries arise from muscular branches of the ophthalmic artery. They reach the eyeball on the tendons of the recti, form a circumcorneal subconjunctival vascular zone, and pierce the sclera near the sclerocorneal junction to end in the major arterial circle of the iris (see p. 685).
Anterior ethmoidal artery
The anterior ethmoidal artery passes with its accompanying nerve through the anterior ethmoidal canal to supply the ethmoidal and frontal air sinuses. Entering the cranium, it gives off a meningeal branch to the dura mater and nasal branches which descend into the nasal cavity with the anterior ethmoidal nerve. It runs in a groove on the deep surface of the nasal bone to supply the lateral nasal wall and septum. A terminal branch appears on the nose between the nasal bone and the upper nasal cartilage (see Ch. 32).
Supratrochlear artery
The supratrochlear artery is a terminal branch of the ophthalmic artery. It leaves the orbit superomedially with the supratrochlear nerve, ascends on the forehead to supply the skin, muscles and pericranium, and anastomoses with the supraorbital artery and with its contralateral fellow.
INNERVATION
Somatic and autonomic motor and somatic sensory nerves are found in the orbit (Figs 39.15, 39.16, 39.17). The oculomotor, trochlear and abducens nerves supply the extraocular muscles. Parasympathetic fibres from the oculomotor nerve supply sphincter pupillae and the ciliary muscle (ciliaris) via the ciliary ganglion, and from the facial nerve innervate the lacrimal gland and choroid via the pterygopalatine ganglion (see Ch. 31). Sympathetic fibres supply dilator pupillae. Both sympathetic and parasympathetic nerves supply the arteries. The sensory nerves within the orbit are the optic, ophthalmic and maxillary nerves (the maxillary nerve and most of the ophthalmic branches only pass through the orbit en route to supply the face and jaws).
Oculomotor nerve
The oculomotor nerve is the third cranial nerve (Fig. 39.15). It innervates four of the extraocular muscles (superior, inferior and medial rectus and inferior oblique), and also conveys parasympathetic fibres which relay in the ciliary ganglion. The nerve emerges at the midbrain, on the medial side of the crus of the cerebral peduncle and passes along the lateral dural wall of the cavernous sinus, dividing into superior and inferior divisions which run beneath the trochlear and ophthalmic nerves. The two divisions enter the orbit through the superior orbital fissure, within the common tendinous ring of the recti, separated by the nasociliary branch of the ophthalmic nerve.
Trochlear nerve
The trochlear nerve is the fourth cranial nerve and innervates superior oblique exclusively. It is the only cranial nerve to emerge from the dorsal surface of the brain stem, passing from the midbrain onto the lateral surface of the crus of the cerebral peduncle. It runs through the lateral dural wall of the cavernous sinus and then crosses the oculomotor nerve to enter the orbit through the superior orbital fissure, above the common tendinous ring and levator palpebrae superioris, and medial to the frontal and lacrimal nerves (Fig. 39.15). The trochlear nerve travels but a short distance to enter the superior (orbital) surface of superior oblique.
Abducens nerve
The abducens nerve is the sixth cranial nerve and innervates lateral rectus exclusively. It emerges from the brain stem between the pons and the medulla oblongata and usually runs through the inferior venous compartment of the petroclival venous confluence in a bow-shaped canal, Dorello’s canal (see Ch. 27). It then bends sharply across the upper border of the petrous part of the temporal bone to enter the cavernous sinus, where it lies lateral to the internal carotid artery (unlike the oculomotor, trochlear, ophthalmic and maxillary nerves, which merely invaginate the lateral dural wall of the sinus). The abducens nerve enters the orbit through the superior orbital fissure, within the common tendinous ring, at first below, and then between, the two divisions of the oculomotor nerve and lateral to the nasociliary nerve (Fig. 39.15). It passes forwards to enter the medial (ocular) surface of lateral rectus.
Optic nerve
The optic nerve is the second cranial nerve. It arises from the optic chiasma on the floor of the diencephalon and enters the orbit through the optic canal, accompanied by the ophthalmic artery. It changes its shape from being flattened at the chiasma to rounded as it passes through the optic canal. In the orbit the optic nerve passes forwards, laterally and downwards, and pierces the sclera at the lamina cribrosa (see p. 695), slightly medial to the posterior pole. It has a somewhat tortuous course within the orbit to allow for movements of the eyeball, and is surrounded by extensions of the three layers of the meninges.
Ophthalmic nerve
The ophthalmic division of the trigeminal nerve arises from the trigeminal ganglion in the middle cranial fossa. It passes forwards along the lateral dural wall of the cavernous sinus, giving off three main branches, the lacrimal, frontal and nasociliary nerves, just before it reaches the superior orbital fissure (Figs 39.16, 39.17). These branches subsequently travel through the orbit to supply targets that are primarily in the upper part of the face (see Ch. 29 for further details of this distribution).
Frontal nerve
Supraorbital nerve
The supraorbital nerve, the larger of the two terminal branches of the frontal nerve, continues forwards along levator palpebrae superioris until it leaves the orbit through the supraorbital notch or foramen. It emerges onto the forehead and supplies the mucous membrane which lines the frontal sinus, the skin and conjunctiva covering the upper eyelid, and the skin over the forehead and scalp (see Ch. 29). The postganglionic sympathetic fibres which innervate the sweat glands of the supraorbital area probably travel in the supraorbital nerve, having entered the ophthalmic nerve via its communication with the abducens nerve within the cavernous sinus.
Nasociliary nerve
Anterior ethmoidal nerve
The anterior ethmoidal nerve passes through the anterior ethmoidal foramen and canal and enters the cranial cavity. It runs forwards in a groove on the upper surface of the cribriform plate beneath the dura mater, and descends through a slit lateral to the crista galli into the nasal cavity, where it occupies a groove on the internal surface of the nasal bone and gives off the medial and lateral internal nasal branches. For a description of the subsequent distribution of the anterior ethmoidal nerve, see Chapter 32.
Maxillary nerve
Most of the branches of the maxillary division of the trigeminal nerve arise in the pterygopalatine fossa (see Ch. 31). They include the zygomatic and infraorbital nerves, which pass into the orbit through the inferior orbital fissure (Fig. 39.17).
Zygomatic nerve
The zygomatic nerve lies close to the base of the lateral wall of the orbit. It divides soon after entering the orbit into two branches, the zygomaticotemporal and the zygomaticofacial nerves, which run within the orbit for only a short distance before passing onto the face through the lateral wall of the orbit. They may either enter separate canals within the zygomatic bone or the zygomatic nerve itself may enter the bone before dividing. For a description of the distribution of the zygomaticotemporal and zygomaticofacial nerves on the face, see Chapter 29.
Infraorbital nerve
The infraorbital nerve initially lies in the infraorbital groove on the floor of the orbit. As it approaches the rim of the orbit it runs into the infraorbital canal through which it passes to emerge onto the face at the infraorbital foramen. It supplies the skin of the lower eyelid and possibly the conjunctiva. For a description of the subsequent distribution of the infraorbital nerve on the face, see Chapter 29.
Ciliary ganglion
The ciliary ganglion is a parasympathetic ganglion concerned with the innervation of certain intraocular muscles. It is a small, flat, reddish-grey swelling, 1–2 mm in diameter, connected to the nasociliary nerve, and located near the apex of the orbit in loose fat approximately 1 cm in front of the medial end of the superior orbital fissure. It lies between the optic nerve and lateral rectus, usually lateral to the ophthalmic artery. Its neurones, which are multipolar, are larger than those found in typical autonomic ganglia; a very small number of more typical neurones are also present.
Its connections or roots (motor, sensory and sympathetic) enter or leave the ganglion posteriorly (Fig. 39.18). Eight to ten delicate filaments, termed the short ciliary nerves, emerge anteriorly from the ganglion, arranged in two or three bundles, the lower being larger. They run forwards sinuously with the ciliary arteries, above and below the optic nerve, and divide into 15–20 branches that pierce the sclera around the optic nerve and run in small grooves on the internal scleral surface. They convey parasympathetic, sympathetic and sensory fibres between the eyeball and the ciliary ganglion: only the parasympathetic fibres synapse in the ganglion.
EYELIDS, CONJUNCTIVA AND LACRIMAL SYSTEM
The exposed ocular surface is protected by retractable eyelids and by a tear film produced mainly by the lacrimal gland, with contributions from glands within the eyelid and conjunctiva (a transparent mucous membrane that covers the inner surface of the eyelid and the exposed surface of the sclera) (see Fig. 40.1).
EYELIDS
The eyelids (palpebrae) are two folds of modified skin which cover the anterior surface of the eye (Fig. 39.19). By their reflex closure, achieved by contraction of orbicularis oculi, they protect the eye from injury and shield the eyes from excessive light. Periodic blinking maintains a thin film of tears over the cornea which prevents desiccation: movement of the eyelid during blinking helps ensure the even distribution of the tear film.
The eyelids pass to adjacent facial skin without obvious demarcation, although their limits are clearly defined in pathological conditions such as oedema. Various skin folds or furrows are of topographical interest. A prominent superior palpebral furrow or fold lies approximately opposite the upper margin of the tarsal plate and is deeply recessed when the lids are open. Oriental Asians have a skin flap, the epicanthus, which begins laterally in the superior palpebral fold and progresses medially to cover the medial canthus. Infant Caucasians frequently have a transient epicanthus which sometimes persists in the adult. A less prominent inferior palpebral furrow occupies a similar position in the skin of the lower lid and deepens on downward gaze. A naso-jugal furrow extends obliquely from the medial lower margin of the bony orbit to the cheek, and a malar furrow may be seen laterally along the inferior orbital rim in middle age, but only infrequently and faintly in the young.
Structure
From its anterior surface inwards each eyelid consists of skin, subcutaneous connective tissue, fibres of the palpebral part of orbicularis oculi (see Fig. 29.11A), submuscular connective tissue, the tarsal plate (tarsus) with its tarsal glands and orbital septum, and palpebral conjunctiva (Fig. 39.20). The upper lid also contains the aponeurosis of levator palpebrae superioris.
Tarsal plates
The two tarsal plates (Fig. 39.21) are thin, elongated, crescent-shaped plates of firm, dense fibrous tissue approximately 2.5 cm long. There is one in each eyelid to provide support and determine eyelid form. Each is convex and conforms to the configuration of the anterior surface of the eye. The free ciliary border is straight and adjacent to the eyelash follicles. The orbital border is convex and attached to the orbital septum. The superior tarsus, the larger of the two, is semi-oval, approximately 10 mm in height centrally. Its inferior edge is parallel to, and approximately 2 mm from, the lid margin. The smaller inferior tarsus is narrower and approximately 4 mm in vertical height.
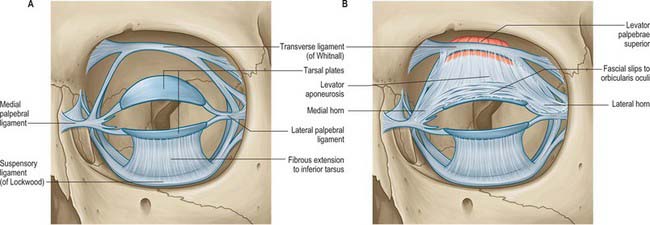
Fig. 39.21 A, Tarsal plates and their attachments. B, Anterior insertion of levator palpebrae superioris.
The tarsal plates are connected to the margins of the orbit by the orbital septum and by the medial and lateral palpebral (canthal) ligaments (Fig. 39.21). The medial palpebral ligament passes from the medial ends of the two tarsal plates to the anterior lacrimal crest and the frontal process of the maxilla. It splits at its insertion into the tarsal plates to surround the lacrimal canaliculi, and lies in front of the nasolacrimal sac and the orbital septum. The lateral palpebral ligament is relatively poorly developed. It passes from the lateral ends of the tarsal plates to a small tubercle on the zygomatic bone within the orbital margin and is more deeply situated than the medial palpebral ligament. It lies beneath the orbital septum and the lateral palpebral raphe of orbicularis oculi.
The deepest fibres of the aponeurosis of levator palpebrae superioris are attached to the anterior surface of the superior tarsus. The superior and inferior tarsal plates are also associated with a thin lamina of smooth muscle forming the superior and inferior tarsal muscles respectively. Opposite the equator of the eye the superior tarsal muscle passes from the inferior face of levator palpebrae superioris to a fibrous extension which projects to the upper margin of the superior tarsus (Fig. 39.20). The muscle is innervated by the sympathetic nervous system and on contraction, elevates the eyelid. Although it may be regarded as supplementing the action of the levator muscle, its full role is not clear. (The mild ptosis that is a characteristic feature of Horner’s syndrome is the result of an interruption to the sympathetic supply to the superior tarsal muscle.) A corresponding but less prominent inferior tarsal muscle in the lower eyelid unites the inferior border, and possibly also the anterior surface, of the inferior tarsus to the capsulopalpebral fascia, which is the anterior expansion of the fused fascial sheath of inferior rectus and inferior oblique. Contraction of inferior rectus during downward gaze therefore also pulls the lower lid downwards. The lower lid is capable of depressing by 4–5 mm, although it is not equipped with a striated muscle counterpart to the levator of the upper lid.
CONJUNCTIVA
The conjunctiva is a thin, transparent mucous membrane that extends from the eyelid margins anteriorly, providing a lining to the lids, before turning sharply upon itself to form the fornices whence it is reflected onto the globe to cover the sclera up to its junction with the cornea. The conjunctiva thus forms a sac that opens anteriorly through the palpebral fissure (Fig. 39.22). At the free palpebral margins the conjunctiva is continuous with the skin, the lining epithelium of the ducts of the tarsal glands, and with the lacrimal canaliculi and lacrimal sac (see below). The continuity between the conjunctiva and the nasolacrimal duct and nasal mucosa is important in the spread of infection (see Ch. 32).
The conjunctiva contributes the mucin component of the pre-ocular tear film and plays a central role in the defence of the ocular surface against microbial infection. It is conventionally divided into five regions: marginal, tarsal, orbital, bulbar and limbal (Fig. 39.22). The marginal, tarsal and orbital regions are collectively referred to as the palpebral conjunctiva.
The marginal zone extends from a line immediately posterior to the openings of the tarsal glands and passes around the eyelid margin to continue on the inner surface of the lid as far as the sub-tarsal groove (a shallow sulcus which marks the inferior edge of the tarsus). The tarsal conjunctiva is highly vascular and is firmly attached to the underlying tarsal plate. The orbital zone extends as far as the fornices, which mark the line of reflection of the conjunctiva from the lids onto the eyeball. The conjunctiva is more loosely attached to underlying tissues over the orbital zone and so folds readily. Elevations of the conjunctival surface in the form of papillae and lymphoid follicles (part of the mucosa associated lymphoid tissue, MALT) are commonly observed in this region (Knop & Knop 2002).
Ducts of the lacrimal gland open into the lateral part of the superior fornix. The bulbar conjunctiva is loosely connected to the eyeball over the exposed sclera, is thin and transparent, and readily permits the visualization of conjunctival and episcleral blood vessels. The loose attachment of the conjunctiva to the fascial sheath of the globe (Tenon’s capsule) in this region means that the conjunctiva is freely movable here: as the bulbar conjunctiva approaches the cornea its surface becomes smoother and its attachment to the sclera increases. The limbal conjunctiva extends approximately 1–1.5 mm around the cornea and contains a dense network of capillaries.
Structure
The conjunctiva is composed of an epithelial layer and an underlying fibrous layer or substantia propria. The form of the epithelium and thickness of the substantia propria varies with location. At the margin of the lids the epithelium is non-keratinized stratified squamous and 10–12 cells thick. The epithelium of the tarsal conjunctiva thins to two or three layers and consists of columnar and flat surface cells. Near the fornices the cells are taller, and a trilaminar conjunctival epithelium covers much of the bulbar conjunctiva. It thickens closer to the corneoscleral junction and then changes to stratified squamous epithelium typical of the cornea (see p. 678). A proportion of limbal conjunctival epithelial cells serve as stem cells for the corneal epithelium: this region of the conjunctiva is therefore essential for maintaining corneal integrity.
LACRIMAL SYSTEM
The lacrimal system consists of structures responsible for the production of tears (principally the main lacrimal gland with a contribution from accessory lacrimal glands) and the lacrimal drainage pathway which collects the tear fluid and conveys it into the nasal cavity (paired lacrimal canaliculi, lacrimal sac and nasolacrimal duct) (Fig. 39.23).
Lacrimal gland
Many small accessory lacrimal glands (glands of Krause and Wolfring) occur in or near the fornix. They are more numerous in the upper eyelid and their presence may explain why the conjunctiva does not dry up after extirpation of the main lacrimal gland.
Microstructure
The lacrimal gland is lobulated and tubuloacinar in form. Its secretory units are acini similar to those found in the salivary glands (Fig. 39.24; see also Ch. 30) (Ruskell 1975). Acini consist of secretory cells which discharge their product into a central lumen continuous with an intercalated duct formed from a single layer of epithelial cells that lack secretory granules. Myoepithelial cells extend processes around the perimeter of acini and ducts; their contraction imparts a mechanical force on the acini and ducts which promotes the expulsion of tears from the gland. The interstices of the gland are composed of loose connective tissue which contains numerous immune cells, mainly B-lymphocytes and plasma cells (particularly IgA-secreting cells).
Innervation
Lacrimation reflex
The lacrimation reflex (Fig. 39.25) is stimulated by irritation of the conjunctiva and cornea, which elicits a large increase in tear volume. The afferent limb of the reflex involves branches of the ophthalmic nerve, with an additional contribution from the infraorbital nerve if the conjunctiva of the lower eyelid is involved. Impulses enter the brain and spread by interneurones to activate parasympathetic neurones in the superior salivatory nucleus (associated with the facial nerve) and sympathetic neurones in the upper thoracic spinal cord. The efferent pathway to the lacrimal gland involves the greater petrosal nerve, which carries preganglionic parasympathetic secretomotor fibres, and the deep petrosal nerve, which conveys postganglionic sympathetic fibres: the parasympathetic fibres relay in the pterygopalatine ganglion, and the sympathetic fibres pass through the ganglion without synapsing.
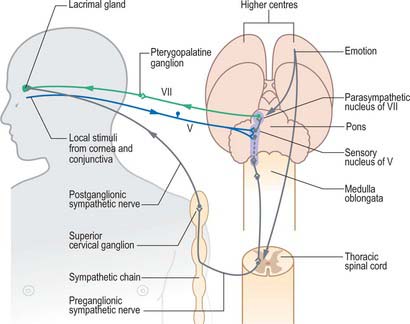
Fig. 39.25 Lacrimation reflex.
(Redrawn from MacKinnon P, Morris J 2005 Oxford Textbook of Functional Anatomy, Vol 3, 2nd edn. Head and Neck. Oxford: Oxford University Press. By permission of Oxford University Press.)
Lacrimation may also occur in response to emotional triggers.
Pre-ocular tear film
The tear film is a complex fluid that covers the exposed parts of the ocular surface framed by the eyelid margins. Classically the tear film has been regarded as a trilaminar structure, with a superficial lipid layer (secreted by the meibomian glands) that overlies an aqueous phase (derived from the main and accessory lacrimal glands) and an inner mucinous layer (produced mainly by conjunctival goblet cells) (Fig. 39.26). The tear film performs a number of important functions. By smoothing out irregularities of the corneal epithelium, it creates an even surface of good optical quality that is reformed with each blink. The air-tear interface forms the principal refractive surface of the optical system of the eye. Since the cornea is avascular, it is dependent on the tear film for its oxygen provision. When the eye is open, the tear film is in a state of equilibrium with the oxygen in the atmosphere, and gaseous exchange takes place across the tear-epithelial interface. The constant turnover of the tear film also provides a mechanism for the removal of metabolic waste products. Tears play a major role in the defence of the eye against microbial colonization: the washing action of the tear fluid reduces the likelihood of microbial adhesion to the ocular surface, and the tears contain a host of protective antimicrobial proteins.
Lacrimal drainage pathway
There is a constant turnover of tears: production is matched by elimination. Some tears are lost by evaporation or absorption across the conjunctiva, but the majority are eliminated via the nasolacrimal drainage system (Fig. 39.23). Tears collect at the medial canthal angle where they drain into the puncta of the upper and lower lids, which are directed towards the surface of the eye to receive tear fluid. From each punctum tears drain into lacrimal canaliculi. There is one canaliculus, approximately 10 mm long, in each lid. Each canaliculus first passes vertically from its punctum for about 2 mm and widens to form an ampulla before passing medially towards the lacrimal sac. The superior canaliculus is smaller and shorter than the inferior. In approximately 80% of adults the canaliculi unite to form a common canaliculus before reaching the lacrimal sac.
The lacrimal sac is the closed upper end of the nasolacrimal duct. It is approximately 12 mm long and lies in a fossa in the lacrimal bone in the anterior part of the medial wall of the orbit (Fig. 39.2). The sac is bounded in front by the anterior lacrimal crest of the maxilla and behind by the posterior lacrimal crest of the lacrimal bone. Its closed upper end is laterally flattened, its lower part is rounded and merges into the duct, and the lacrimal canaliculi open into its lateral wall near its upper end.
Knop E, Knop N. A functional unit for ocular surface immune defence formed by the lacrimal gland, conjunctiva and lacrimal drainage system. Adv Exp Med Biol. 2002;506B:635-644.
Koornneef L. The architecture of the musculo-fibrous apparatus in the human orbit. Acta Morphol Neerl Scand. 1977;15:35-64.
Leigh RJ, Zee DS. The Neurology of Eye Movement, 4th edn. Oxford: Oxford University Press, 2006.
Ruskell GL. Nerve terminals and epithelial cell variety in the human lacrimal gland. Cell Tiss Res. 1975;138:121-136.