Chapter 5 The lumbar lordosis and the vertebral canal
The lumbar lordosis
The intact lumbar spine is formed when the five lumbar vertebrae are articulated to one another (Fig. 5.1). Anteriorly the vertebral bodies are separated by the intervertebral discs and are held together by the anterior and posterior longitudinal ligaments. Posteriorly the articular processes form the zygapophysial joints, and consecutive vertebrae are held together by the supraspinous, interspinous and intertransverse ligaments and the ligamenta flava.
Although the lumbar vertebrae can be articulated to form a straight column of vertebrae, this is not the shape assumed by the intact lumbar spine in the upright posture. The reason for this is that the sacrum, on which the lumbar spine rests, is tilted forwards, so that its upper surface is inclined downwards and forwards. From radiographs taken in the supine position, the size of this angle with respect to the horizontal plane of the body has a mean value of about 42–45°,1–3 and is said to increase by about 8° upon standing.1
If a straight lumbar spine articulated with the sacrum, it would consequently be inclined forwards. To restore an upward orientation and to compensate for the inclination of the sacrum, the intact lumbar spine must assume a curve (see Fig. 5.1). This curve is known as the lumbar lordosis.
The junction between the lumbar spine and the sacrum is achieved through joints like those between the lumbar vertebrae. Anteriorly, the body of the L5 vertebra forms an interbody joint with the first sacral vertebra, and the intervertebral disc of this joint is known as the lumbosacral disc. Posteriorly, the inferior articular processes of L5 and the superior articular processes of the sacrum form synovial joints, known either as the L5–S1 zygapophysial joints or as the lumbosacral zygapophysial joints. A ligamentum flavum is present between the laminae of L5 and the sacrum, and an interspinous ligament connects the L5 and S1 spinous processes. However, there is no supraspinous ligament at the L5–S1 level,4 nor are there intertransverse ligaments, the latter having been replaced by the iliolumbar ligament.
The shape of the lumbar lordosis is achieved as a result of several factors. The first of these is the shape of the lumbosacral intervertebral disc. This disc is unlike any of the other lumbar intervertebral discs in that it is wedge shaped. Its posterior height is about 6–7 mm less than its anterior height.5 Consequently, when the L5 vertebra is articulated to the sacrum, its lower surface does not lie parallel to the upper surface of the sacrum. It is still inclined forwards and downwards but less steeply than the top of the sacrum. The angle formed between the bottom of the L5 vertebra and the top of the sacrum varies from individual to individual over the range 6–29° and has an average size of about 16° (Fig. 5.2).5
The second factor that generates the lumbar lordosis is the shape of the L5 vertebra. Like the lumbosacral disc, the L5 vertebral body is also wedge shaped. The height of its posterior surface is some 3 mm less than the height of its anterior surface.6 As a consequence of the wedge shape of both the L5 body and the lumbosacral disc, the upper surface of L5 lies much closer to a horizontal plane than does the upper surface of the sacrum.
The remainder of the lumbar lordosis is completed simply by inclination of the vertebrae above L5. Each vertebra is inclined slightly backwards in relation to the vertebra below. As a result of this inclination, the anterior parts of the anuli fibrosi and the anterior longitudinal ligament are stretched. Posteriorly, the intervertebral discs are compressed slightly, and the inferior articular processes slide downwards in relation to the superior articular processes of the vertebra below, and may impact either the superior articular process or the pedicle below. The latter phenomenon has particular bearing on the weight-bearing capacity of the zygapophysial joints and is described further in Chapter 8.
Magnitude
Various parameters have been used by different investigators to quantify the curvature of the lumbar lordosis, although they all involve measuring one or other of the angles formed by the lumbar vertebral bodies (see Fig. 5.2). Some have used the angle formed by the planes through the top surface of L1 and the top surface of the sacrum,7,8 and this could be called the ‘L1–S1 lordosis angle’. Fernand and Fox (1985)9 measured the angles between the top of L2 and the top of the sacrum, and between the top of L2 and the bottom of L5, which they called, respectively, the ‘lumbosacral lordotic angle’ and the ‘lumbolumbar lordotic angle’. Others have measured the angle between the top of L3 and the sacrum,10 or the angle formed between planes that bisect the L1–L2 disc and the L5–S1 disc.11,12 Consequently, the measures obtained in these various studies differ somewhat from one another. Nevertheless they all show substantial ranges of variation.
In radiographs taken in the supine position, the angle between the top of L1 and the top of the sacrum varies from 20° to more than 60° but has an average value of about 50°.7 In the standing position, this same angle has been measured as 67° (±3° standard deviation, SD) in children, and 74° (±7° SD) in young males.13 The angle between the top of L2 and the sacrum has a range of 16–80° and a mean value of 45°.9 A value greater than 68° is considered to indicate a hyperlordotic curve.9 However, despite a common belief that excessive lordosis is a risk factor for low back pain, comparison studies reveal that there is no correlation between the shape of the lumbar lordosis and the presence or absence of back pain symptoms.7,10,12
Stability
As described in Chapter 3, the lumbar zygapophysial joints provide a bony locking mechanism that resists forward displacement, and the degree to which a joint affords such resistance is determined by its orientation. The more a superior articular process faces backwards, the greater the resistance it offers to forward displacement.
To resist the tendency for the L5 vertebra to slip forwards, the superior articular processes of the sacrum face considerably backwards. The average orientation of the L5–S1 zygapophysial joints with respect to the sagittal plane is about 45° with most lumbosacral zygapophysial joints assuming this orientation (see Fig. 3.4). Only a minority of joints assume a greater or lesser angle. Joints with a greater angle, i.e. facing backwards to an even greater extent, provide greater resistance to forward displacement of L5, but they provide less resistance to axial rotation (twisting movements) of L5. Joints with an angle less than 45° provide greater protection against rotation but less against forward displacement. An angle of 45° is therefore a satisfactory compromise, allowing the lumbosacral zygapophysial joints to resist both rotation and forward displacement.
The L4–5 zygapophysial joints are also orientated at about 45° (see Fig. 3.4) and thereby resist forward displacement of the L4 vertebra. Above L4, the slopes of the upper surfaces of the vertebral bodies are horizontal or inclined backwards, and there is no tendency, at rest, for the upper lumbar vertebrae to slide forwards. Consequently, there is less need for the upper lumbar zygapophysial joints to face backwards, and their angle of orientation is progressively less than 45° (see Fig. 3.4). Such resistance as may be required to resist forward displacement of these joints during flexion of the lumbar spine is nevertheless afforded by the curved shape of their articular surfaces. Although their general orientation is closer to the sagittal plane, the anteromedial ends of the articular surfaces of the upper lumbar joints face backwards and can resist forward displacement, if required (see Ch. 3).
One of the advantages of a curved lumbar spine lies in this resilience. By being curved, the lumbar spine is protected to an appreciable extent from compressive forces and shocks. In a straight lumbar spine, an axial compressive force would be transmitted through the vertebral bodies and intervertebral discs, and the only mechanism to protect the lumbar vertebrae would be the shock-absorbing capacity of the intervertebral discs (see Ch. 2). In contrast, in a curved lumbar spine, compressive forces are transmitted through the posterior ends of the intervertebral discs while the anterior ends of the vertebral bodies tend to separate. In other words, compression tends to accentuate the lumbar lordosis. This tendency will cause the anterior ligaments to become tense, which, in turn, will resist the accentuation. In this way, some of the energy of the compressive force is diverted into stretching the anterior ligaments instead of being transmitted directly into the next vertebral body.
The vertebral canal
In the intact lumbar spine, the vertebral foramina of the five lumbar vertebrae are aligned to form a continuous channel called the vertebral canal (Fig. 5.3). The anterior wall of this canal is formed by the posterior surfaces of the lumbar vertebrae, the intervening discs and the posterior longitudinal ligament. The posterior wall is formed by the laminae of the vertebrae and the intervening ligamenta flava. Because operations on the lumbar spine are most frequently performed with the patient in the prone position, the anterior and posterior walls of the vertebral canal are, by convention, alternatively referred to as the floor and roof of the vertebral canal, respectively.
The floor of the vertebral canal is not absolutely flat because the posterior surfaces of the lumbar vertebral bodies exhibit slight curves, transversely and longitudinally. The posterior surfaces of the L1 to L3 vertebrae regularly exhibit a slight transverse concavity. In contrast L5 is slightly convex while L4 exhibits an intermediate curvature.14 Along the sagittal plane, the lumbar vertebrae present a slightly concave posterior surface so that in profile the floor of the vertebral canal presents a scalloped appearance.15 This scalloping is believed to be produced by the pulsatile, hydrostatic pressure of the cerebrospinal fluid in the dural sac, which occupies the vertebral canal.15
The lateral walls of the vertebral canal are formed by the pedicles of the lumbar vertebrae. Between the pedicles, the lateral wall is deficient where the superior and inferior vertebral notches appose one another to form the intervertebral foramina. Each intervertebral foramen is bounded anteriorly by an intervertebral disc, the adjacent lower third of the vertebral body above, and the uppermost portion of the vertebral body below (Fig. 5.4). Above and below, each intervertebral foramen is bounded by a pedicle, while posteriorly it is bounded by a vertebral lamina and a zygapophysial joint. More accurately, the posterior boundary of each intervertebral foramen is the lateral portion of the ligamentum flavum that covers the anterior aspect of the lamina and zygapophysial joint (see Ch. 4).
Subdivisions of the vertebral canal, recognised by surgeons because of their relationship to the spinal nerve roots,16–19 are the so-called radicular canals. These are not true canals because they do not have boundaries around all their aspects. More accurately, they are only subdivisions of the space of the vertebral canal and intervertebral foramina, through which the spinal nerve roots run (see Ch. 10), but in so far as they form a series of bony relations to the course of the nerve roots, they may be regarded as canals.
Each radicular canal is a curved channel running around the medial aspect of each pedicle in the lumbar spine, and each can be divided into three segments.19 The uppermost, or retrodiscal segment, lies above the level of the pedicle. Its anterior wall is formed by the intervertebral disc in this region, while its posterior wall is formed by the uppermost end of a superior articular process (Fig. 5.5). This segment lacks a lateral wall because it lies opposite the level of an intervertebral foramen. Similarly, it has no medial wall for in this direction it is simply continuous with the rest of the vertebral canal.
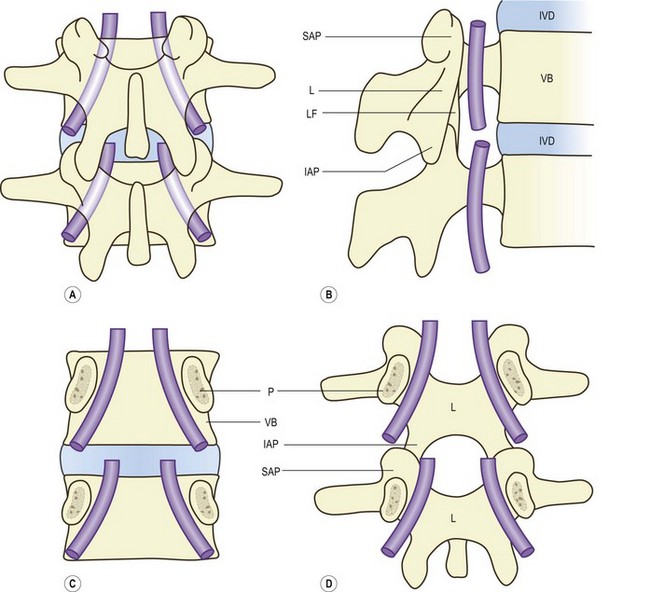
Figure 5.5 The radicular canals. (A) The location of the radicular canals (shaded) in a dorsal view of the lumbar spine. (B) A view of the radicular canals from within the vertebral canal, showing their lateral, anterior and posterior boundaries. (C) The anterior and lateral boundaries of the radicular canals, viewed from behind. (D) The posterior and lateral boundaries of the radicular canals, as seen from within the vertebral canal, looking at its roof. The ligamentum flavum has not been included (see also Fig. 4.5B). IAP, inferior articular process; IVD, intervertebral disc; L, lamina; LF, ligamentum flavum; P, pedicle; SAP, superior articular process; VB, vertebral body.
The parapedicular segment lies immediately medial to the pedicle, which therefore forms its lateral wall. Anteriorly, this segment is related to the back of the vertebral body, while posteriorly it is covered by the vertebral lamina and the anteromedial edge of the superior articular process that projects from this lamina (see Fig. 5.5). Technically, this segment of the radicular canal is simply the lateral portion of the vertebral canal opposite the level of a pedicle, and for this reason this segment is also known as the lateral recess (of the vertebral canal). A lateral recess is therefore present on both sides of the vertebral canal opposite each of the lumbar pedicles.
The third segment of the radicular canal is formed by the upper part of the intervertebral foramen: that part behind the vertebral body and below the upper pedicle (see Fig. 5.5).
The anatomical relevance of the radicular canals is that the lumbar nerve roots run along them; the anatomy of these nerves is described in Chapter 10. The clinical relevance lies in the propensity for the nerve roots to be compressed by structural alterations in one or other of the structures that form boundaries to the canals.
Another concept of relevance to nerve root compression concerns narrowing of the vertebral canal. The shape and size of the lumbar vertebral canal govern the amount of space available for the nerves that the canal transmits, and if this space is in any way lessened by encroachment of the boundaries of the canal, the condition is referred to as canal stenosis or spinal stenosis.20–26
In transverse section, the lumbar vertebral canal varies in shape. It is oval at upper lumbar levels, becoming triangular more caudally, sometimes assuming a trefoil shape at lower lumbar levels (Fig. 5.6).27
The term ‘trefoil’ refers to a triangular shape in which the angles are stretched or accentuated.28 The basal angles of the triangular or trefoil outline are formed by the lateral recesses of the vertebral canal.
The shape and size of the vertebral canal can be abnormally small as a result of aberrations in the development of the neural arch. In relation to the size of the vertebral canal, the pedicles may be too thick or the articular process may be too large. In effect, the space left in the vertebral canal becomes relatively too small for the volume of nerves that it has to transmit. This condition is called congenital or developmental spinal stenosis,20 but by itself developmental stenosis does not cause compression of nerves. It only renders the patient more likely to compression in the face of the slightest aberration of the boundaries of the vertebral canal.20,23
Acquired spinal stenosis occurs whenever any of the structures surrounding the vertebral canal is affected by disease or degeneration that results in enlargement of the structure into the space of the vertebral canal. Examples of such processes include buckling of the ligamentum flavum, osteophytes from the zygapophysial joints or intervertebral discs, and intervertebral disc herniations or bulges.17,20,23,25 Such changes may occur at single levels in the vertebral canal or at multiple levels, and symptoms may arise either from the disease process that caused the changes or as a result of compression of one or more nerves by the encroaching structure. The pathogenesis of symptoms in spinal stenosis is described further in Chapter 15.
1 Arnoldi CC, Brodsky AE, Cauchoix J, et al. Lumbar spinal stenosis. Clin Orthop. 1976;115:4-5.
2 Bose K, Balasubramaniam P. Nerve root canals of the lumbar spine. Spine. 1984;9:16-18.
3 Crock HV. Normal and pathological anatomy of the lumbar spinal nerve root canals. J Bone Joint Surg. 1981;63B:487-490.
4 Dommisse GF. Morphological aspects of the lumbar spine and lumbosacral regions. Orthop Clin North Am. 1975;6:163-175.
5 Ehni G. Significance of the small lumbar spinal canal:cauda equina compression syndromes due to spondylosis. J Neurosurg. 1962;31:490-494.
6 Eisenstein S. The trefoil configuration of the lumbar vertebral canal. J Bone Joint Surg. 1980;62B:73-77.
7 Epstein JA, Epstein BS, Levine L. Nerve root compression associated with narrowing of the lumbar spinal canal. J Neurol Neurosurg Psychiatry. 1962;25:165-176.
8 Farfan HF, Huberdeau RM, Dubow HI. Lumbar intervertebral disc degeneration. The influence of geometrical features on the pattern of disc degeneration – a post-mortem study. J Bone Joint Surg. 1972;54A:492-510.
9 Fernand R, Fox DE. Evaluation of lumbar lordosis. A prospective and retrospective study. Spine. 1985;10:799-803.
10 Gilad I, Nissan M. Sagittal evaluation of elemental geometrical dimensions of human vertebrae. J Anat. 1985;143:115-120.
11 Hansson T, Bigos S, Beecher P, et al. The lumbar lordosis in acute and chronic low-back pain. Spine. 1985;10:154-155.
12 Hellems HK, Keates TE. Measurement of the normal lumbosacral angle. Am J Roentgenol. 1971;113:642-645.
13 Heylings DJA. Supraspinous and interspinous ligaments of the human spine. J Anat. 1978;125:127-131.
14 Kirkaldy-Willis WH, Wedge JH, Yong-Hing K, et al. Pathology and pathogenesis of lumbar spondylosis and stenosis. Spine. 1978;3:319-328.
15 Larsen JL. The posterior surface of the lumbar vertebral bodies. Part 1. Spine. 1985;10:50-58.
16 Larsen JL. The posterior surface of the lumbar vertebral bodies. Part 2. An anatomic investigation concerning the curvatures in the horizontal plane. Spine. 1985;10:901-906.
17 Parkin IG, Harrison GR. The topographical anatomy of the lumbar epidural space. J Anat. 1985;141:211-217.
18 Pearcy M, Portek I, Shepherd J. Three-dimensional X-ray analysis of normal movement in the lumbar spine. Spine. 1984;9:294-297.
19 Pelker RR, Gage JR. The correlation of idiopathic lumbar scoliosis and lumbar lordosis. Clin Orthop. 1982;163:199-201.
20 Pope MH, Bevins T, Wilder DG, et al. The relationship between anthropometric, postural, muscular, and mobility characteristics of males ages 18–55. Spine. 1985;10:644-648.
21 Schmorl G, Junghanns H. The Human Spine in Health and Disease, 2nd American ed. New York: Grune & Stratton; 1971.
22 Splithoff CA. Lumbosacral junction: roentgenographic comparisons of patients with and without backache. JAMA. 1953;152:199-201.
23 Torgerson WR, Dotter WE. Comparative roentgenographic study of the asymptomatic and symptomatic lumbar spine. J Bone Joint Surg. 1976;58A:850-853.
24 Verbiest H. A radicular syndrome from developmental narrowing of the lumbar vertebral canal. J Bone Joint Surg. 1954;36B:230-237.
25 Verbiest H. Pathomorphological aspects of developmental lumbar stenosis. Orthop Clin North Am. 1975;6:177-196.
26 Verbiest H. Fallacies of the present definition, nomenclature and classification of the stenoses of the lumbar vertebral canal. Spine. 1976;1:217-225.
27 Vital JM, Lavignolle B, Grenier N, et al. Anatomy of the lumbar radicular canal. Anat Clin. 1983;5:141-151.
28 Von Lackum HL. The lumbosacral region. An anatomic study and some clinical observations. JAMA. 1924;82:1109-1114.