11 The Lens
DEVELOPMENT AND GROWTH OF THE LENS
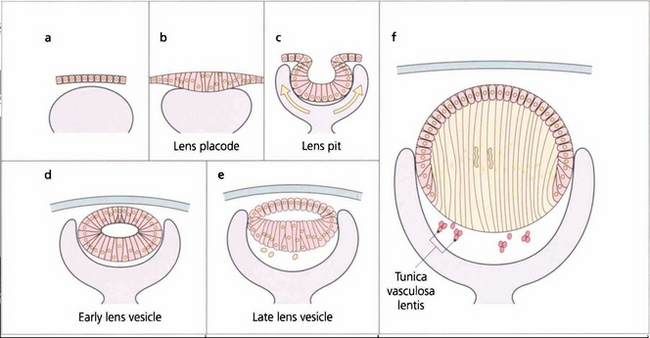
Fig. 11.1 Recently there has been considerable research on the genetic control of lens development and on the growth factors such as fibroblast growth factor and insulin-like growth factor that modulate the development of the lens vesicle and its invagination into the optic vesicle. The lens placode differentiates from the surface ectoderm at the 4-mm stage (about 3 weeks after conception). By 14 mm (6 weeks) it has separated from the surface ectoderm to form the lens vesicle and the embryonic lens nucleus then begins to form. The lens is supported by the tunica vasculosa lentis which is derived from the hyaloid vascular system. This blood supply is fully formed by 3 months’ gestation after which it begins to atrophy and has disappeared by the end of the seventh month of gestation.
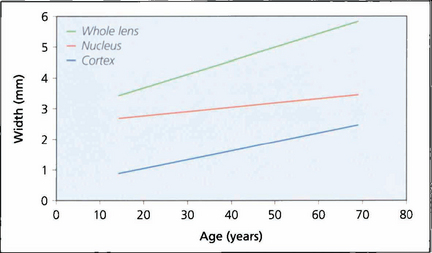
Fig. 11.2 A normal lens increases in thickness with age. The observed increase is the sum of growth by fibre accretion and central compaction. Increasing thickness is largely the result of cortical growth; lenses that develop cataract tend to be smaller than their age-matched norm until the onset of hydration with maturity.
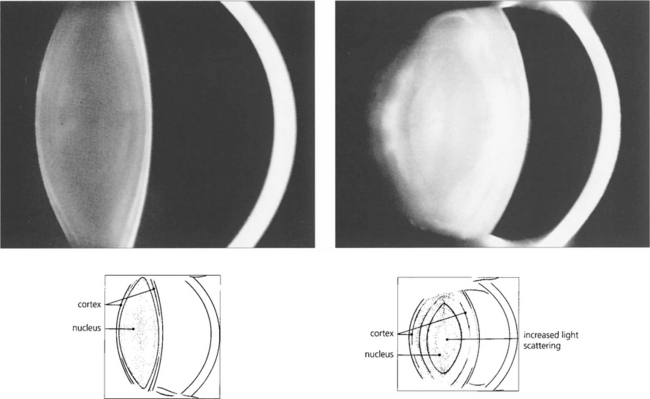
Fig. 11.3 These Scheimphlug images compare the lens of a 10-year-old child with that of an elderly person. In the child the lens is composed largely of nucleus, the cortex being represented only by a thin bright band. The lens in the older person is greatly increased in thickness due almost entirely to growth of the cortex with the nucleus remaining almost unchanged. The scattering properties of the lens are greatly increased in the elderly so that the lens appears to be brighter; in addition the radii of curvature of its surfaces, particularly the anterior surface, are reduced so that the lens becomes more convex.
By courtesy of Mr N Phelps Brown.
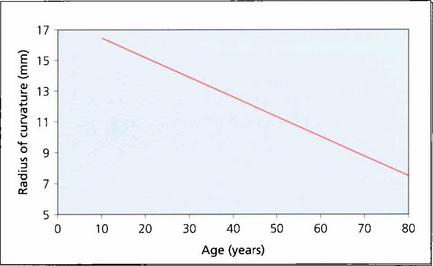
Fig. 11.4 The radius of curvature of the anterior surface of the lens becomes progressively shorter with age (i.e. more convex), resulting in increased postive spherical aberration (see Ch. 1). The cornea has a positive spherical aberration too so that with age there is increasing aberration which leads to image degradation and loss of contrast. This is of some interest as intraocular lenses are now being manufactured with a negative spherical aberration to neutralize the corneal aberration leading to better contrast post- operatively.
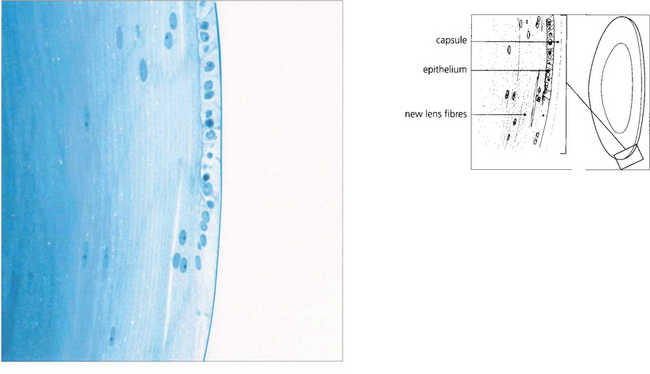
Fig. 11.5 This histological section shows the anterior surface of the lens at the equator. The capsule appears structureless and stains weakly. It is the basement membrane of the lens epithelium and consists of type 4 collagen. It varies in thickness between 10 and 20μm and is thinnest at the anterior and posterior poles and the equator. The lens epithelium, which is present only anteriorly, is composed of a single layer of nucleated cells. It is responsible for the continual production of new lens fibres which are seen to be separating from the epithelial layer in the lower part of the figure. Although the young fibres are nucleated they eventually lose their nuclei as they sink into the cortex.
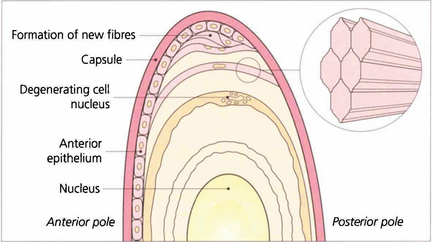
Fig. 11.6 Lens fibres are hexagonal in shape and lie in layers rather like the skin of an onion. Individual fibres are connected to one another by ‘ball and socket’ interdigitations. Initially they are attached to the capsule anteriorly and posteriorly (their basement membrane). With time and the formation of new fibres they lose their capsular attachments, their nuclei and interdigitations and cell membranes to become compacted as insoluble protein in the nucleus.
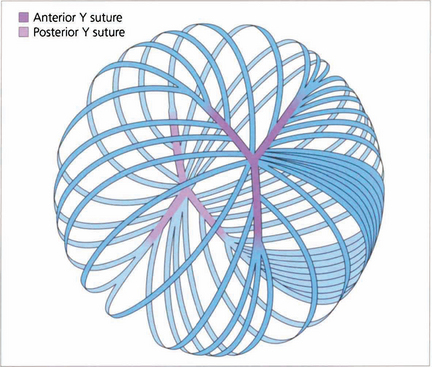
Fig. 11.7 Each fibre is a long ribbon-like structure with ends that stretch to each anterior and posterior pole where they meet a fellow fibre from the opposite side. If the system was completely symmetrical they would meet at a single point but this is not the case and they meet one another in a complicated branching system known as suture lines. The suture lines of the fetal nucleus are easily seen in the normal adult lens as an erect Y anteriorly and an inverted Y posteriorly. With increasing age the sutures become a more complex branching system extending out into the cortex, each lens fibre running anteroposteriorly across the equator of the lens and interdigitating with its neighbours.
ACCOMMODATION
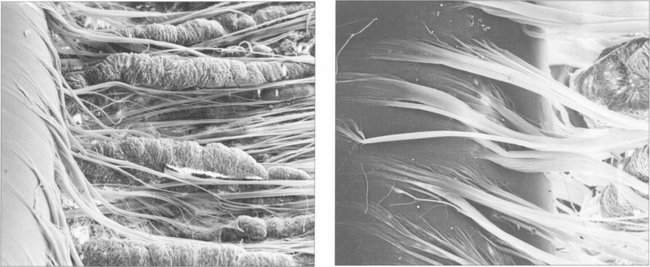
Fig. 11.9 (Left) Zonular fibres can be seen inserting into the lens capule from between the ciliary processes (right).
By courtesy of Professor John Marshall.
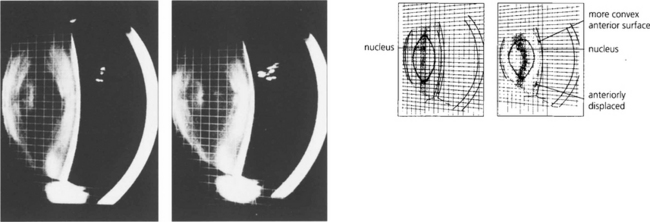
Fig. 11.10 These slit-image Scheimphlug photographs show the changes in a 30-year-old lens focused at infinity (left) and exerting 10D of accommodation (right). The increased curvature of the anterior lens surface and its slight anterior positioning can be seen clearly. The posterior lens surface remains relatively unchanged.
By courtesy of Mr N Phelps Brown.
ANOMALIES OF SHAPE AND POSITION
Anomalies of lens shape and position are rare disorders either resulting from primary lens pathology or due to secondary zonular changes. They are either genetic (in which case there may be other systemic abnormalities) or a result of trauma or pseudo lens exfoliation, which is associated with zonular weakness. Pupil block glaucoma is a common feature of subluxating or dislocating lenses (see Ch. 8). Intact lenses that dislocate posteriorly can lie in the vitreous or on the retinal surface for many years without causing ocular damage.
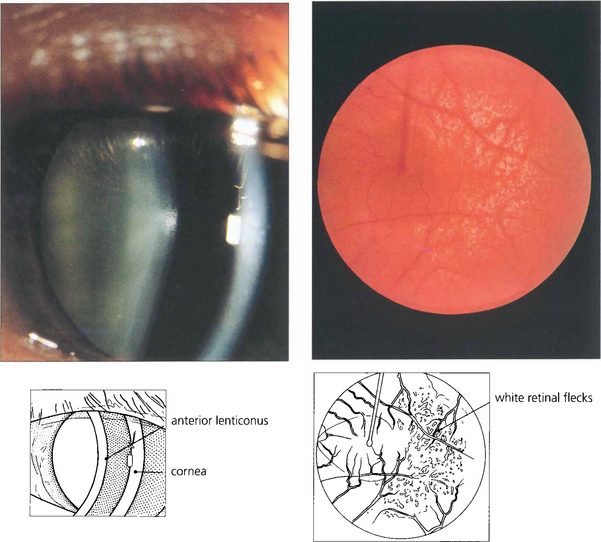
Fig. 11.11 Anterior lenticonus is a feature of Alport’s syndrome. This is a dominant disorder of variable penetrance that is due to a basement membrane defect. As well as the lens defect, which causes irregular astigmatism, the condition is associated with renal failure and neuronal deafness. Patients may have an associated retinopathy of white inner retinal flecks.
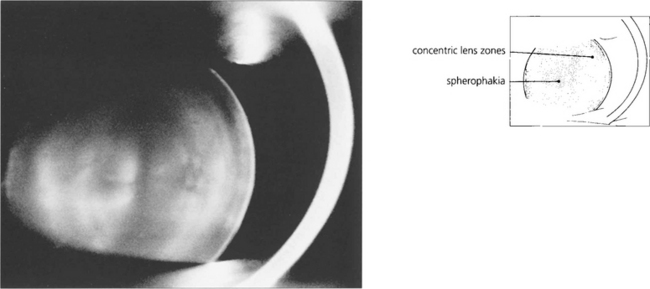
Fig. 11.12 Spherophakia is seen in a number of conditions in which the lens has an abnormally short radius of curvature and may also be exceptionally small (microphakia). Extreme myopia results. Although this lens is normally situated, there is usually a tendency for lenses to subluxate and dislocate anteriorly.
By courtesy of Mr N Phelps Brown.
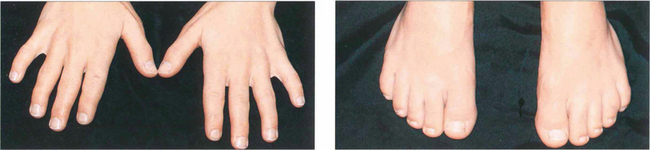
Fig. 11.13 The Weil–Marchesani syndrome is inherited recessively and characterized by short stature and stubby fingers (left) and toes (right), which have stiff joints. Spherophakia, lenticular myopia of 10–20D and lens dislocation are common. Heterozygotes may show a milder form of the disease.
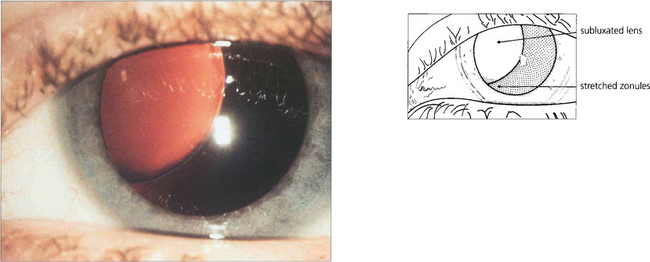
Fig. 11.14 Marfan’s syndrome is a dominantly inherited disorder that affects the eye, skeleton and cardiovascular system. It is due to a deficiency of fibrillin, a component of the microfibrillar system associated with elastin and encoded on chromosome 15q. The lens tends to dislocate upwards (in contrast to homocystinuria where it dislocates inferiorly). The lens is more spherical, causing myopia with marked astigmatism. Management of these patients has been transformed by a complex procedure of lens removal, placement of a sclerally sutured endocapsular ring to recentre the bag and IOL implantation.
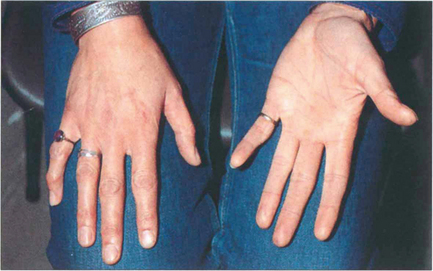
Fig. 11.15 Patients with Marfan’s syndrome have arachnodactyly and an arm span greater than their height. All patients require cardiac monitoring to detect the potentially fatal complications of aortic valve incompetence and a dissecting ascending aortic aneurysm.
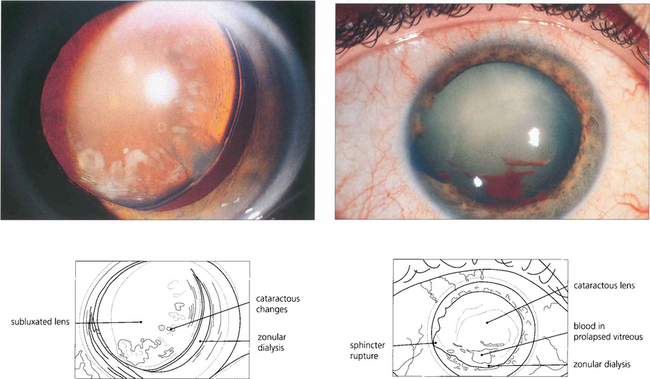
Fig. 11.17 Traumatic zonular dehiscence (left) should be looked for by eliciting phacodonesis on preoperative mydriasis in any patient who has a history of ocular injury. This patient (right) has a traumatic mydriasis, cataract and vitreous in the anterior chamber indicating zonular damage following a blow to the eye; ultrasonography is required to exclude posterior rupture of the lens capsule if the cataractous change is too dense to visualize the capsule.
CATARACT
A cataract is any opacity within the lens. Cataracts are classified according to their morphology and position within the lens and graded by the degree of opacity or ‘maturity’ produced. If lens damage is insufficient to progress to maturity a localized opacity is produced in the injured region that becomes surrounded by new lens fibres as they are laid down beneath the capsule (see glaucomflecken Ch. 7). The three major types of age-related cataract are nuclear, cortical and posterior subcapsular opacity; many patients have combinations of these. It has been suggested that these represent different disease processes: nuclear changes being caused by protein denaturation, cortical by damage to lens fibres and posterior subcapsular cataract by migration of lens epithelial cells posteriorly. This remains to be proven. Occasionally the morphology of a cataract may give an indication of its aetiology (e.g. posterior subcapsular cataract with trauma or steroids) and this may have important medicolegal implications. The morphology does, however, influence the patient’s symptomatology.
Genetic factors have shown to be important risk factors for age related nuclear and cortical opacity; other recognized cataractogenic environmental risks are sunlight, smoking, dehydration and chronic diarrhoea (Table 11.1).
Congenital | Acquired |
---|---|
Maternal infection (e.g. rubella) | Age related |
Genetic | Metabolic (diabetes, hypothyroidism, atopy) |
Metabolic (e.g. galactosaemia) | Drugs (steroids) |
Chromosomal (e.g. Down’s syndrome) | Intraocular disease (uveitis, retinitis pigmentosa) |
Ocular developmental (e.g. Peters’ anomaly) | Trauma (blunt injury, radiotherapy, intraocular surgery) |
Trauma | Genetic (age related nuclear and cortical, Dystrophia myotonica) |
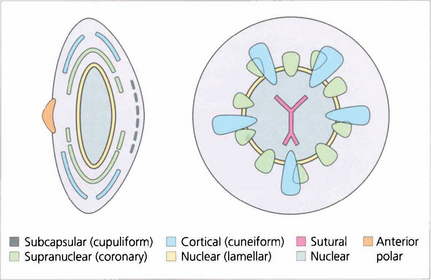
Fig. 11.18 This diagram illustrates the different morphological patterns of cataract together with their depth and location within the lens.
CAPSULAR CATARACT
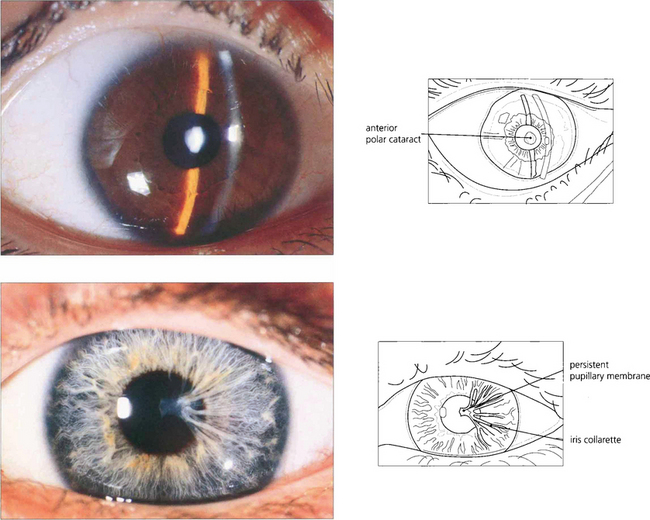
Fig. 11.19 Anterior polar capsular opacity is usually congenital. It can lie flat on the surface or protrude from the lens forwards as a small pyramid (top); sometimes remnants of a persistent pupillary membrane are present (bottom). Underlying lens fibres are affected and may produce an appearance similar to a stack of plates in the anterior cortex. The defect appears to be due to failure of lens fibres to meet and interdigitate at the anterior pole. Anterior polar opacities do not affect visual acuity as severely as those in the posterior pole which lie closer to the nodal point of the eye.
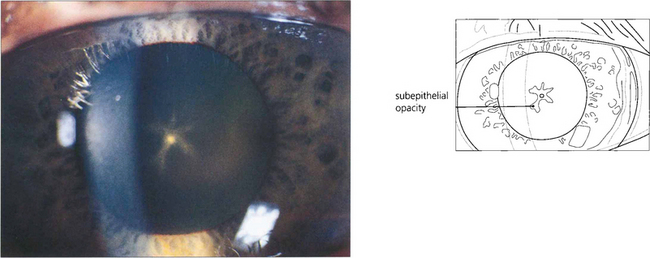
Fig. 11.20 Following prolonged use of high doses of chlorpromazine in psychiatric treatment a characteristic pigmented deposit appears on the capsule with a star-shaped cataract in the subepithelial fibres outlining the lens sutures. This patient also had increased cutaneous and corneal pigmentation, a well recognized feature of long-term phenothiazine treatment.
SUBCAPSULAR CATARACT
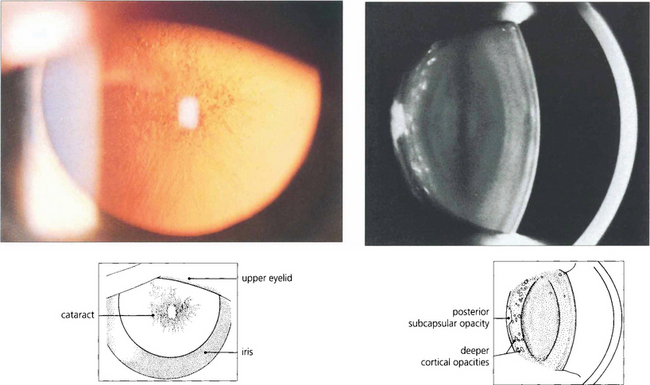
Fig. 11.21 Posterior subcapsular changes start as a polychromic lustre of the cortex adjacent to the posterior capsule progressing to a greyish granular opacity. The subjacent cortex often contains opacities. These deeper cortical opacities are a sign of long-standing changes and were themselves subcapsular at the time of their formation.
By courtesy of Mr N Phelps Brown.
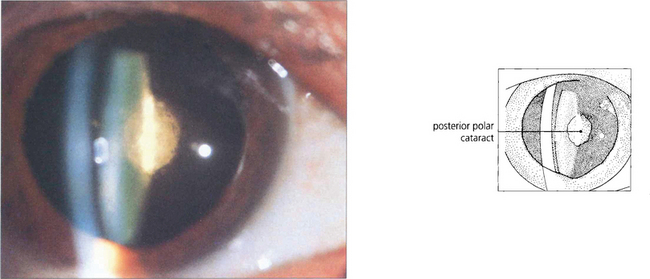
Fig. 11.22 This is a discrete dense posterior polar cattaract. These are often associated with a weakened or deficient posterior capsule which is of considerable surgical importance.
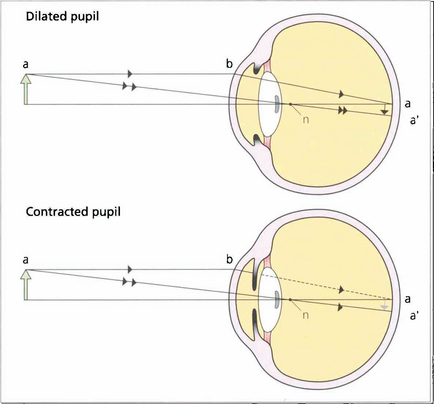
Fig. 11.23 The position of a posterior subcapsular cataract on the visual axis has a very destructive effect on vision as it lies near the nodal point of the eye so that light must pass through this point. Pupillary constriction in bright light enhances this effect because the reduced aperture prevents the entrance of the more peripheral and less affected rays.
TRAUMATIC SUBCAPSULAR CHANGES
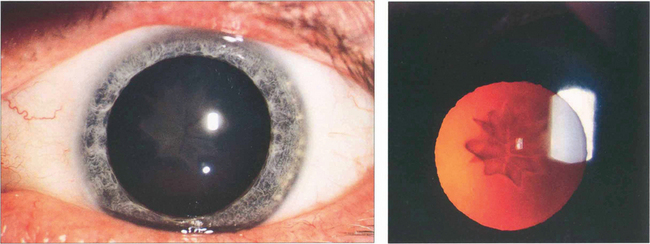
Fig. 11.24 Star-shaped cataracts at the posterior pole are a typical but uncommon sign of blunt injury which more commonly produces just posterior subcapsular lens opacity. A possible explanation of the appearance is that the superficial lens cortex ruptures at the suture line allowing fluid into the fibre tips which then spreads up the fibre before the defect is sealed.
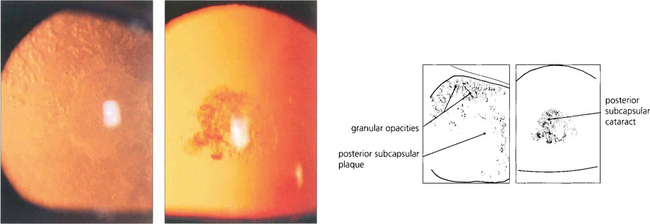
Fig. 11.25 Radiation cataract results from injury to the lens by ionizing radiation (X-rays, β-rays, γ-rays and neutrons); about 4Gy is sufficient to produce a cataract. It begins as a centripetal streaming of granular particles in the posterior subcapsular zone (left), which form a plaque of opacities at the posterior pole. After severe irradiation the cataract deteriorates to maturity but with more minor irradiation the cataract eventually becomes a static discrete opacity which comes to lie deeper in the lens with time (right).
CORTICAL CATARACTS
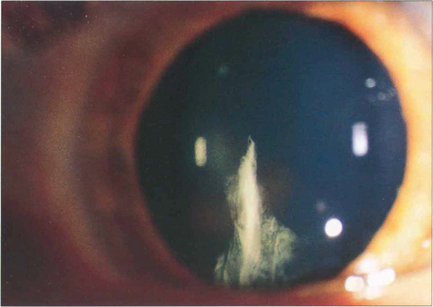
Fig. 11.26 Spoke, or cuneiform, cataracts are a relatively common form of senile opacity that lie in radial wedge shapes at about mid-depth in the cortex. They form peripherally and produce symptoms when they extend into the pupillary area.
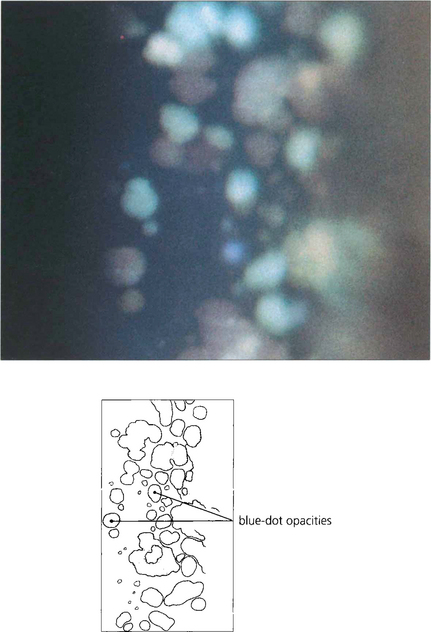
Fig. 11.27 Congenital blue dot or punctate opacities are seen as discrete punctate opacities with a bluish colour throughout the cortex. They have little effect on vision and are often found coincidentally on routine examination, although patients tend to develop presenile cataract.
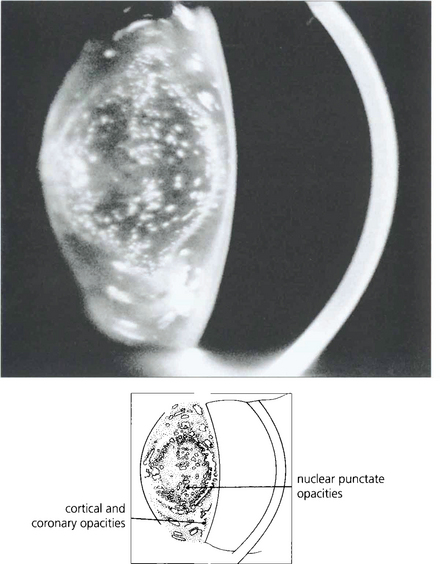
Fig. 11.28 While punctate cataracts are usually situated in the cortex, they may also be seen within the nucleus if opacity formation began either in utero or in infancy.
By courtsey of Mr N Phelps Brown.
NUCLEAR CATARACTS
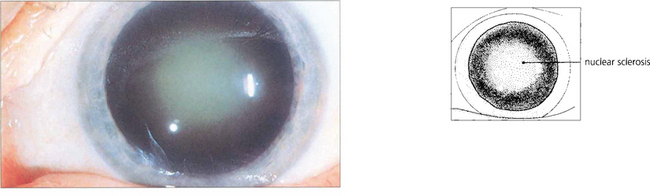
Fig. 11.31 The common age-related nuclear cataract is seen as a central opalescence in the lens that scatters light.
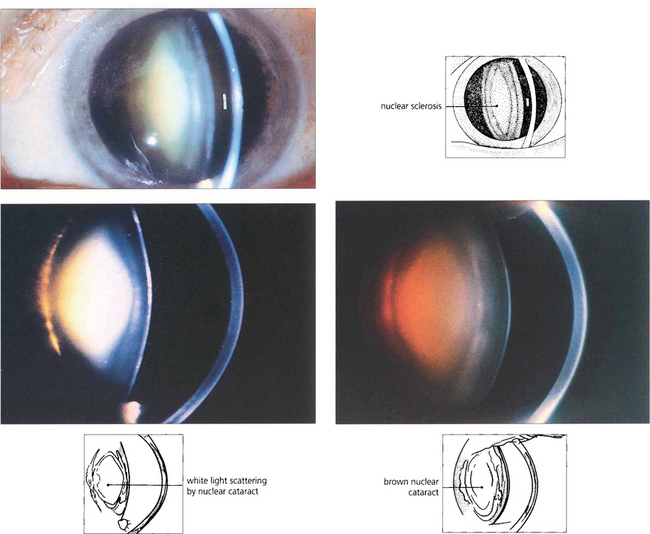
Fig. 11.32 Nuclear cataracts also show increasing brunescent pigmentation from yellow through to dark brown. This is an important physical sign as brunescence correlates well with increasing lens hardness and hence more phaco power is required for removal. Brunescence absorbs as well as scatters light and alters the patient’s colour perception; this may have a beneficial effect as it protects the macula from short wavelength blue light which is thought to be a risk factor in age-related macular degeneration. Increasing brunescence lowers visual performance at low light levels and alters colour perception. This is thought to explain why artists such as Turner and Rembrandt used a predominance of orange, red and brown colours in their later paintings.
By courtesy of Mr N Phelps Brown.
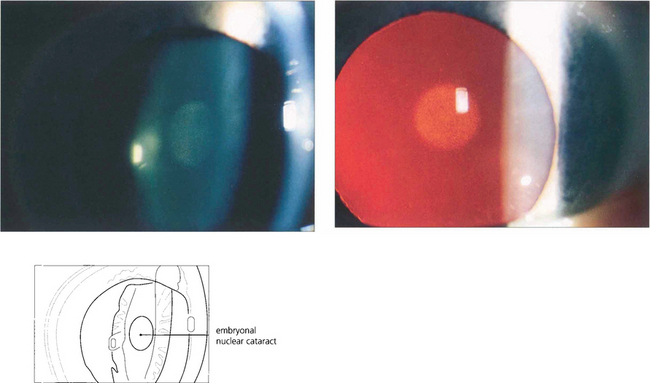
Fig. 11.33 In congenital cataract the extent to which the nucleus is affected depends on the duration of cataract formation in utero. The embryonal nuclear cataract, cataracta centralis pulverulenta – or Coppock cataract after the family in which it was first described – consists of a small grey opacity in the embryonal nucleus. It is bilateral and dominantly inherited and does not interfere with vision because of its small size. The genetics are well understood and the condition is due to expression of an abnormal γ-crystallin in the prenatal period.
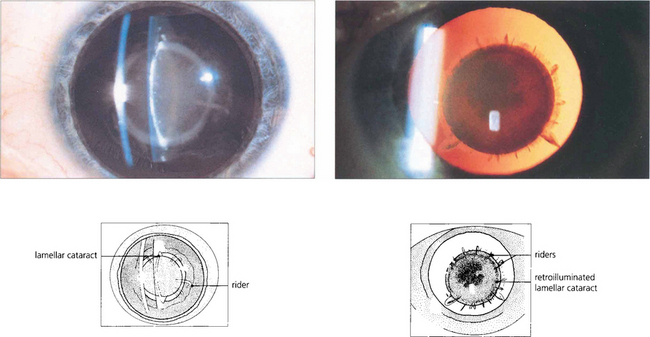
Fig. 11.34 Lamellar cataract affects a layer of lens fibres damaged by an insult for a limited period of time. It is usually seen as a nuclear opacity that is congenital in origin although when first formed the cataract is actually subcapsular and moves deeper into the lens as normal new fibres are laid down over it. If the precipitating cause continues to a lesser extent individual groups of fibres are subsequently affected and are seen as ‘riders’ over the main opacity.
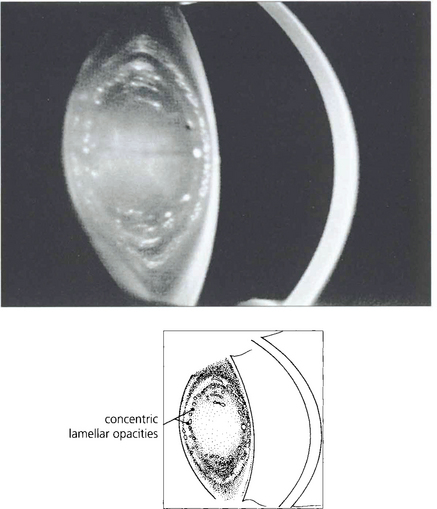
Fig. 11.35 Scheimphlug photography in this lens shows two affected concentric lamellae.
By courtesy of Mr N Phelps Brown.
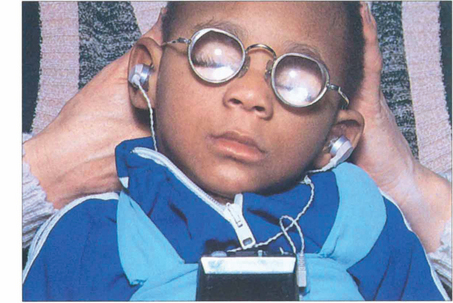
Fig. 11.36 The commonest cause of congenital cataracts used to be maternal rubella infection occurring during the first trimester of pregnancy. Fortunately the incidence of this infection has declined dramatically as a result of immunization of the female population. Affected infants have active virus infection in the eye and raised titres of rubella antibody in the blood. Other signs of the disease are frequently present as in this 2-year-old child who had bilateral nerve deafness, microcephaly, severe intellectual impairment and a congenital heart defect. Some patients also have retinopathy (see Ch. 16).
PROGRESS AND PROGNOSIS OF CATARACT
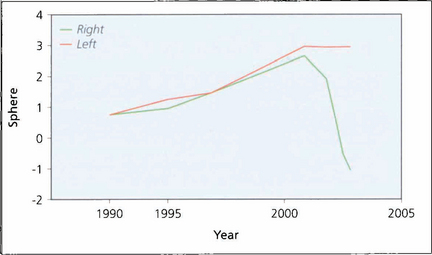
Fig. 11.37 In the normal ageing eye there is gradual shift towards hyperopia with age but lenses developing nuclear cataract shift towards myopia which frequently preceeds diagnosis of the cataract. This is caused by increased lens thickness and swelling. This graph shows the spherical equivalent in a patient followed for many years. Increasing hyperopia is seen until the development of a cataract in the right eye when there is a rapid myopic shift.
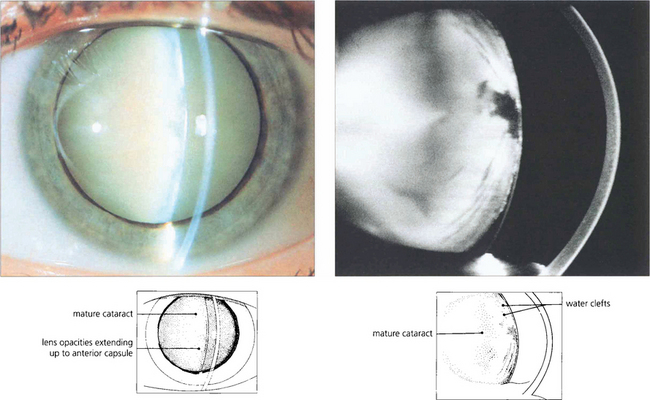
Fig. 11.38 A cataract is said to be mature when all fibres are opacified up to the capsule (a); this is associated with water entry into the lens by osmosis and swelling. The Scheimphlug image of another patient (b) shows water clefts, seen as dark areas between fibre lamellae.
By courtesy of Mr N Phelps Brown.
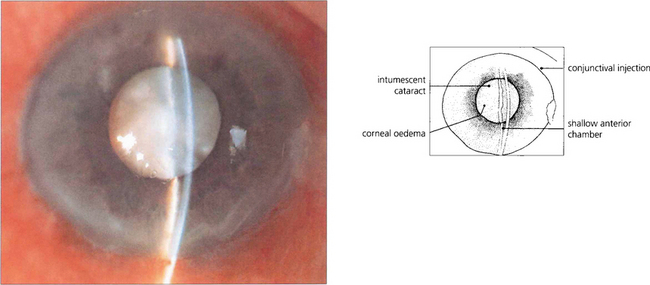
Fig. 11.39 The maturing lens, even in young subjects, can become intumescent to such a degree that it causes shallowing of the anterior chamber, pupil block and closed-angle glaucoma. Phacolytic glaucoma can also result from leakage of lens protein into the anterior chamber (see Ch. 8).
CATARACTS AND SYSTEMIC DISEASE
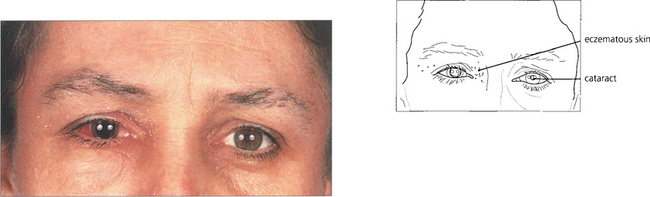
Fig. 11.42 This young woman has cataracts associated with severe chronic eczema, possibly exacerbated by steroids used to control the disease. She has had recent surgery to the right eye; the left has a mature cataract. Such patients have an increased risk of postoperative bacterial endophthalmitis from the skin disease.
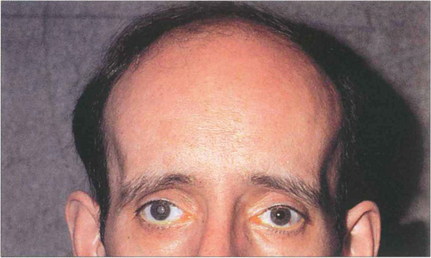
Fig. 11.43 Dystrophia myotonica is a dominantly inherited condition commonly associated with cataract. This disease increases in severity with successive generations. This patient has the typical facial appearance. Notice the frontal balding, wasting of the temporalis muscles and evidence of external ocular myopathy with bilateral ptosis. Characteristically polychromatic glistening granules start to appear during the teenage years in the subcapsular and cortical areas and progress to posterior subcapsular changes and maturity. These opacities can be a useful genetic marker although the lens is sometimes unaffected in people who have overt disease. Myopathy of the respiratory muscles can make general anaesthesia hazardous.
PAEDIATRIC CATARACT
Congenital and infantile cataract is associated with a myriad of rare genetic and metabolic causes, as well as more common causes (see Table 11.1). Most congenital cataracts are picked up by routine screening of healthy neonates when there is no red reflex or as a dense spot against the red reflex on ophthal-moscopy, as a non-seeing baby, as a squint or on routine surveillance of children with a predisposing risk. Most paediatric cataracts are suitable for IOL implantation the major contraindication being paediatric uveitis. Ophthalmic management brings together the problems of challenging surgery, refractive correction in a developing eye, amblyopia and posterior capsule opacification often in the context of a multiply handicapped child.
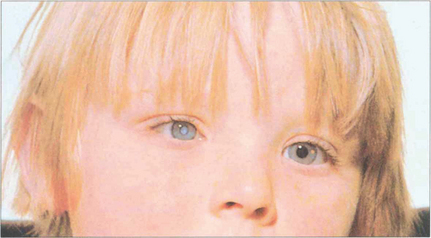
Fig. 11.44 As well as having a mature cataract the right eye of this child is convergent, and microphthalmic. He had rubella retinopathy in the left eye. Ultrasonography is required to eliminate posterior segment pathology in the right eye. In this situation successful visual results from surgery are extremely rare because of the insuperable problems of coexisting eye disease, optical correction and treatment of amblyopia. Treatment is therefore cosmetic.
COMPLICATIONS OF CATARACT SURGERY
Cataract surgery accounts for about 70 per cent of all ophthalmic surgery. In Western countries about 4 to 6 operations are performed per 1000 population; this equates to about 1 million operations a year in the USA and the number is forecast to rise as a result of increasing surgical success justifying earlier intervention and increasing longevity of the population. Serious complications are rare but because of the vast amount of surgery being performed even rare complications affect a lot of people. Complications of phaco-emulsification surgery can be divided into operative and early or late postoperative (more than 3 months after operation) (Table 11.2). The most serious complications arise from infection, posterior segment complications and the consequences of posterior capsular rupture.
Operative | Early postoperative | Late postoperative |
---|---|---|
Phaco wound burn* | Infection† | IOL decentration* |
Iris touch* | Wound leak† | Posterior capsule opacification* |
Corneal touch* | Raised intraocular pressure | Inadequate refractive correction* |
Rhexis tear* | Iritis | Retinal detachment† |
Zonular dialysis* | Medication allergy | Corneal endothelial failure† |
Posterior capsule rupture† | Retained lens material* | Failure of previous trabeculectomy* |
Dropped nucleus† | Pseudophaktc cystoid macular oedema* | |
Vitreous loss† | ||
Choroidal haemorrhage | ||
Induced astigmatism |
†potentially sight-threatening complication.
OPERATIVE COMPLICATIONS
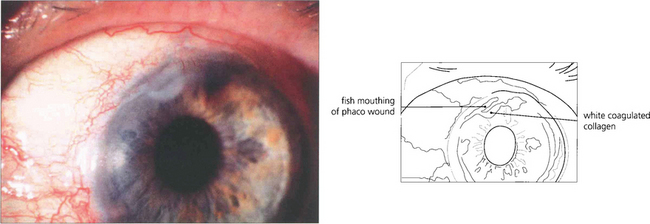
Fig. 11.45 Corneal wound burn has come into prominence with the advent of small-incision clear corneal phaco-emulsification surgery. It is caused by a failure of aspiration, too tight a wound or too much phaco power. Heat is transmitted to the wound causing collagen shrinkage, fish mouthing and defective closure and leading to increased postoperative astigmatism and a risk of postoperative endophthalmitis.
Courtesy of Mr D Smerdon
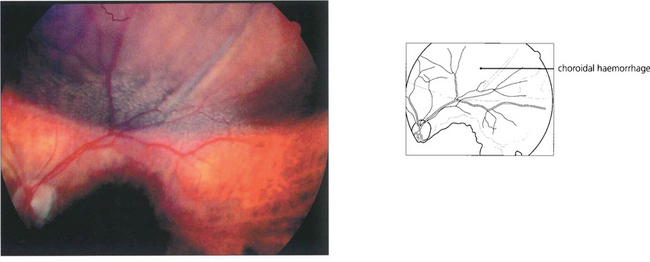
Fig. 11.46 Choroidal haemorrhage is a rare but very serious intra operative complication occuring more commonly in hypertensive patients and exacerbated if the patient is anticoagulated. The eye suddenly develops a shallow anterior chamber and high intraocular pressure.
By courtesy of Mr B Damato
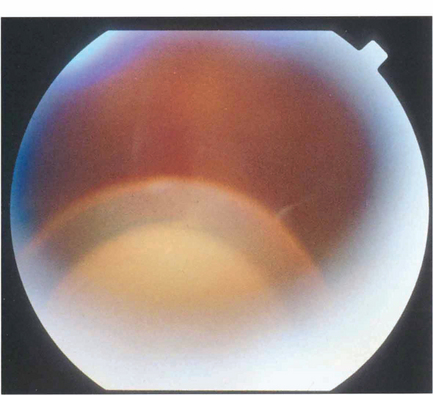
Fig. 11.47 Rupture of the posterior capsule during phaco-emulsification can lead to loss of nuclear fragments into the vitreous cavity followed by uveitis, glaucoma and corneal decompensation. Fragments are best removed by a formal vitreo retinal procedure.
By courtesy of Mr T Williamson
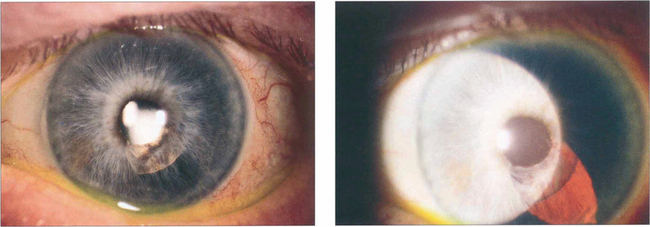
Fig. 11.48 The iris can be damaged either by touch with the phaco tip or from prolapse into the wound. This patient had an iris prolapse through too large a side port incision during the procedure resulting in loss of the pigment epithelium with the potential risk of pupillary distortion, iris translucency and synechiae.
EARLY POSTOPERATIVE COMPLICATIONS
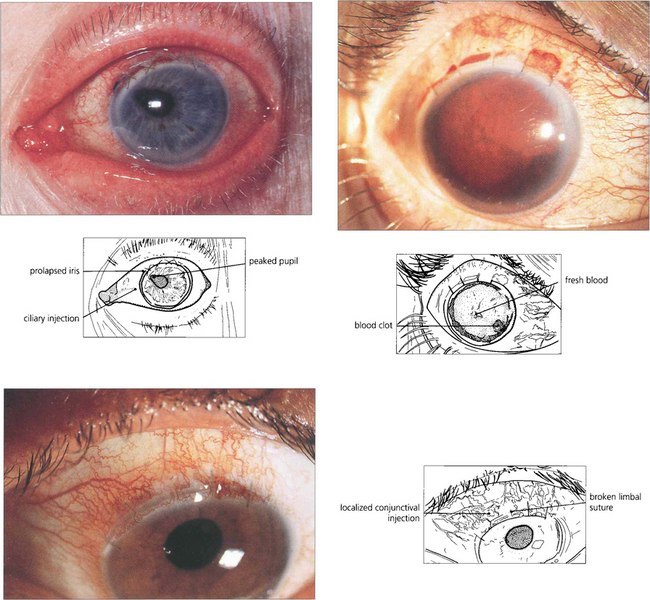
Fig. 11.49 Wound-related problems have largely disappeared with the change from extracapsular to phaco-emulsification surgery. Iris prolapse (top left) results from poor surgical technique, Valsalva manoeuvre or pressure on the eye; extensive hyphemas (top right) may be associated with raised pressure or corneal blood staining. Ultrasonography to delineate the intraocular anatomy is indispensable if further surgery is contemplated. Rupture of sutures occurs 1–2 years after surgery when the nylon digests and erodes through the epithelium (bottom left). Patients present with a foreign body sensation and a red discharging eye.
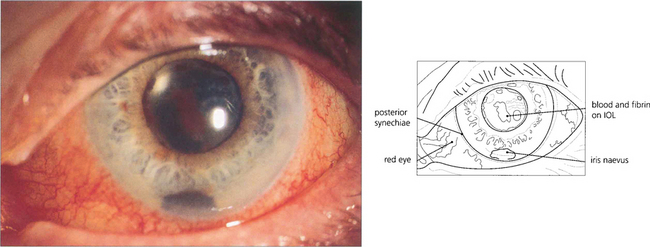
Fig. 11.50 Current rates of postoperative bacterial endophthalmitis are in the order of 2 per 1000 surgeries. After surgery a patient should have a progressively whiter and more comfortable eye with improving vision. Increasing pain, photophobia and redness suggest endophthalmitis. Such patients need to be seen as an acute emergency and, if examination confirms the diagnosis, require a vitreous tap for bacterial culture and intraviteal antibiotics. This patient presented 2 weeks after surgery. The vitreal tap grew a fully sensitive coagulase-negative staphlococcus.
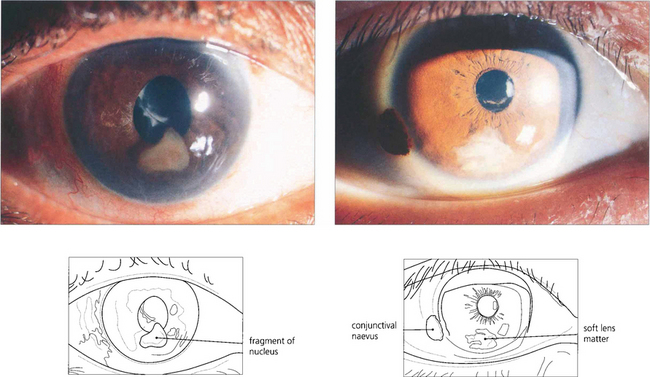
Fig. 11.51 Nuclear fragments (left) left in the eye after surgery will cause inflammation and glaucoma and must be removed. Soft lens matter (right) may be absorbed spontaneously if there is a limited amount but patients need to remain under observation until it has disappeared completely.
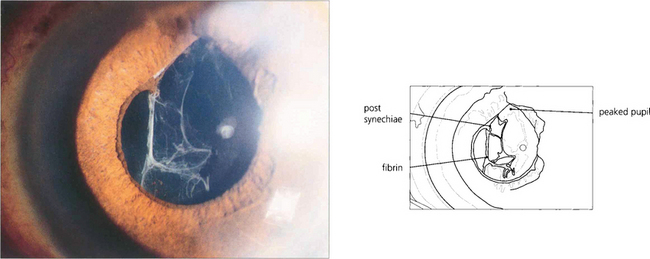
Fig. 11.52 A fibrinous reaction after routine surgery is unusual unless the operation was traumatic. This patient suffered iris damage when the operation was converted from phaco-emulsification to an extracapsular procedure. The anterior chamber cleared on treatment with topical steroids and mydriatics.
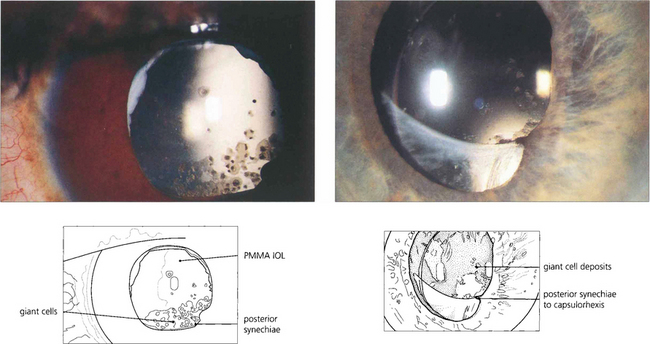
Fig. 11.53 Postoperative inflammation can cause a foreign-body giant cell reaction to the IOL. This seen most commonly with hydrophobic IOL materials as pigmented multinucleate cells on the anterior IOL surface and eyes with large numbers of cells (left) have a high incidence of posterior synechiae and cystoid macular oedema. Giant cells are often seen adjacent to posterior synechiae (right). The cells gradually reduce over many months with treatment or as the inflammation resolves.
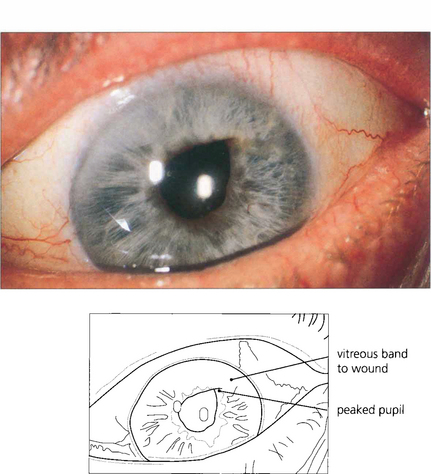
Fig. 11.55 Vitreous loss with entrapment in the wound and distortion of the pupil occurs from dialysis of the zonule or rupture of the posterior capsule at the time of surgery and an inadequate vitrectomy. Such eyes carry a risk of prolonged inflammation, cystoid macular oedema and retinal detachment. Small bands such as this can be cut with a YAG laser.
LATE POSTOPERATIVE COMPLICATIONS
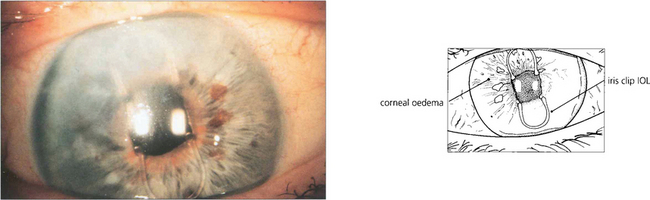
Fig. 11.57 Corneal oedema can result from damage to the corneal endothelium as a result of excessive phaco power or endothelial touch during surgery, from the instillation of incorrect solutions at operation because of a failure in procedure, from decompensation of a pre-existing endothelial dystrophy such as Fuchs’ dystrophy (see Ch. 6) or long-term endothelial touch from an anterior chamber lens in which case endothelial failure occurs many years later. In this eye with an iris clip lens the superior corneal endothelium was probably damaged during implant insertion leading to corneal oedema 5 years later.
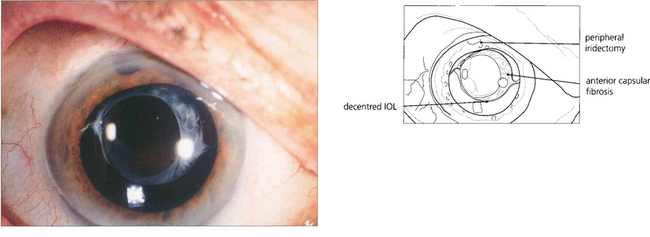
Fig. 11.58 Postoperative capsular fibrosis from a contracting capsular bag (often in association with a torn or asymmetrical rhexis or pseudoexfoliation of lens capsule) can displace and decentre the IOL. This patient, who had had a phaco trabeculectomy, presented with difficulty with night driving. Examination shows a mildly decentred PMMA IOL, bringing the lens edge into the visual axis with mydriasis.
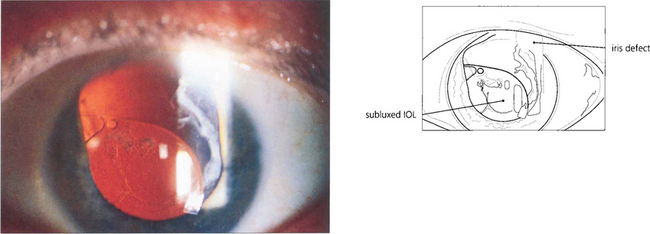
Fig. 11.59 This patient had a secondary sulcus placed IOL following a traumatic injury and broad iridectomy some years previously which subluxated inferiorly soon after surgery from unrecognized previous zonular dialysis. The IOL was repositioned and fixated with a scleral suture technique.
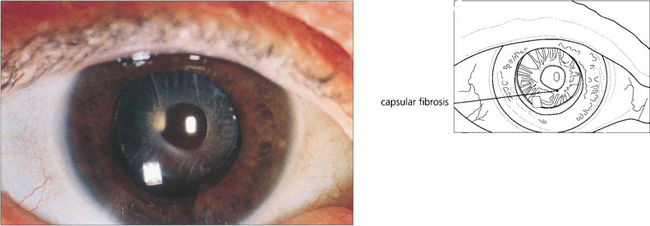
Fig. 11.60 Capsular phimosis is caused by excessive anterior capsular fibrosis. It can lead to blurred vision, glare or poor night vision. It is most commonly seen with pseudo lens exfoliation or some types of hydrophobic lens materials.
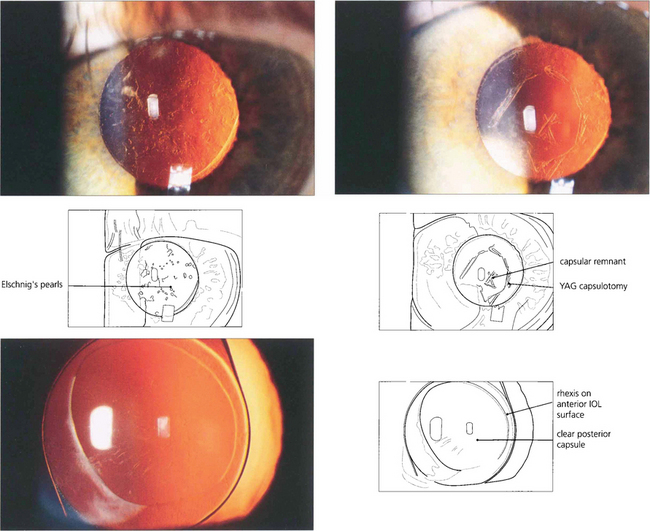
Fig. 11.61 Posterior capsule opacification (top left) is the commonest complication of routine phaco-emulsification surgery. It is caused by migration of the residual lens epithelial cells, inevitably left at the time of surgery, from the equator over the posterior capsule producing symptoms similar to those of the previous cataract 1–2 years later. The problem is easily treated by laser capsulotomy (top right), but this carries a small risk of medical complications and is inconvenient and expensive. The use of IOLs with a square edge profile (bottom left) inhibits this process and the rates of this complication are starting to fall significantly as a result.
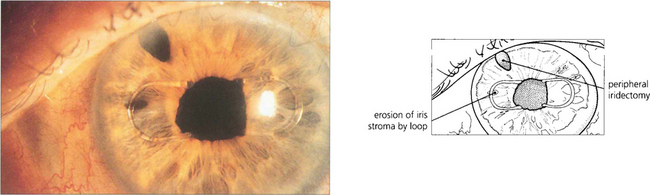
Fig. 11.62 The IOL, especially if placed in the sulcus, can chafe the iris or iris pigment epithelium resulting in uveitis, pigmentary glaucoma or recurrent hyphema (the UGH syndrome). This was a particular problem with iris clip IOLs which chaffed on the iris surface. This patient presented with recurrent hyphema. Two years later this produced a full-thickness hole and the symptoms resolved.
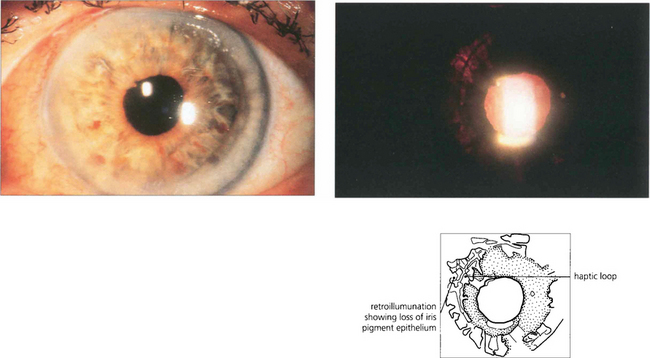
Fig. 11.63 In another patient a posterior chamber sulcus placed IOL can be seen on retroillumination to chaff the iris pigment epithelium. This patient had pigmentary glaucoma.
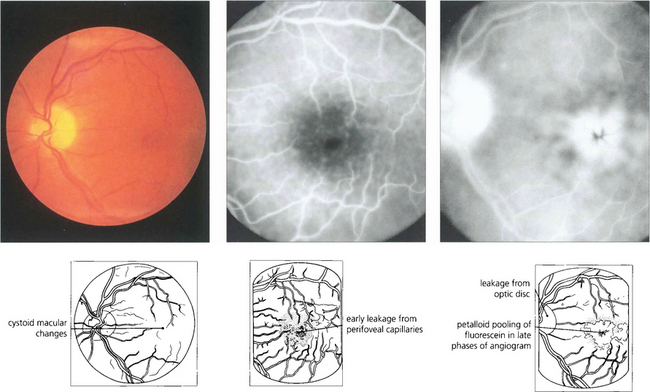
Fig. 11.64 Visually significant pseudo-phakic cystoid macular oedema occurs in 1–2 per cent of patients after surgery and subclinical changes may be seen on fluorescein angiography in many more patients. Most patients have transient symptoms, but a few have permanent visual loss. Treatment with topical steroids and a nonsteroidal agent such as ketorolac for 3 months leads to resolution in the majority of cases.
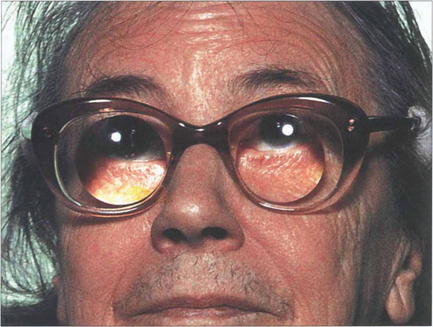
Fig. 11.65 If, for some reason, an IOL cannot be inserted the postoperative aphakic must be corrected by means of glasses or contact lenses. Aphakic glasses magnify by 30 per cent; they produce visual distortion from spherical aberration and a restricted visual field from the prismatic effects of the lens, which also produces a roving ‘Jack-in-the-box’ scotomatous effect. Contact lenses produce about 4–5 per cent magnification and allow fusion in a monocular aphakic patient at the expense of the problems of contact lens management.