CHAPTER13 The Electrodiagnostic Examination
Pathophysiology
Because axon loss and demyelinating conduction block stop nerve impulse transmission across the lesion site rather than merely slowing it, both can result in clinical weakness and sensory abnormalities whenever they affect a sufficient number of motor and sensory axons. Demyelinating conduction slowing does not affect muscle strength, however. This is because all of the nerve impulses ultimately reach their destination, although slightly later in time than they normally would.1
General Concepts of Electrodiagnostic Examination
Nerve Conduction Studies
Three basic types of NCS are available: motor, sensory, and mixed (Fig. 13–1). Motor and sensory NCS are generally performed on every patient. Mixed NCS are typically used in the evaluation of specific disorders, such as carpal tunnel syndrome, and are of limited value in the evaluation of spine-related nerve pathology. NCS protocols vary depending on the diagnosis in question and can be tailored to help exclude other diagnoses in the differential. Most electrodiagnostic laboratories have a routine protocol, however, for a general study of the upper extremity (Table 13–1) and lower extremity (Table 13–2).
Motor | Sensory |
---|---|
Standard | |
Median: thenar (C8, T1) | Median: index (C6, C7) |
Ulnar: hypothenar (C8, T1) | Ulnar: fifth (C8) |
Nonstandard | |
Ulnar: first dorsal interosseus (C8, T1) | Median: thumb (C6) |
Radial: extensor indicis proprius (C8) | Median: middle (C7) |
Radial: brachioradialis (C5, C6) | Ulnar: hand dorsum (C8) |
Musculocutaneous: biceps (C5, C6) | Radial: thumb base (C6, C7) |
Axillary: deltoid (C5, C6) | Lateral antebrachial cutaneous: forearm (C6) |
Medial antebrachial cutaneous: forearm (T1) |
Note: On each line, the nerve being studied is listed first, followed after the colon by the recording site and then, in parentheses, the root innervation (motor) or derivation (sensory). Underlined root provides major innervation.
TABLE 13–2 Nerve Conduction Studies in the Lower Limb
Motor | Sensory |
---|---|
Standard | |
Peroneal: extensor digitorum brevis (L5-S1) | Sural: lateral ankle (S1) |
Tibial: abductor hallucis (S1) | |
Nonstandard | |
Peroneal: tibialis anterior (L5) | Superficial peroneal sensory: dorsum ankle (L5) |
Tibial: abductor digiti quinti pedis (S1) | Saphenous: medial ankle (L4)† |
Tibial: gastrocnemii (S1)* | Lateral femoral cutaneous: lateral thigh (L3, L4)† |
Femoral: quadriceps (L3, L4) |
Note: On each line, the nerve being studied is listed first, followed after the colon by the recording site and then, in parentheses, the root innervation (motor) or derivation (sensory).
† Studies technically difficult to perform.
Motor Nerve Conduction Studies
For motor NCS, the recording electrode is placed over the muscle belly, and the reference electrode is affixed over the tendon. The nerve supplying that muscle is stimulated, and the resulting motor nerve response is a compound muscle action potential (CMAP), a biphasic waveform that represents summated muscle fiber action potentials (Fig. 13–2). In routine motor NCS, small muscles of the hand and feet serve as recording muscles, and the nerves supplying them are stimulated at two separate points along their course. For the upper extremity, the wrist (distal) and elbow (proximal) are used as stimulation sites, and for the lower extremity, the ankle (distal) and knee (proximal) are used as stimulation sites.
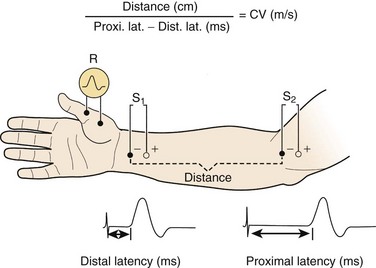
FIGURE 13–2 Various components of motor nerve conduction study. (The median nerve is being assessed.)
(Modified from Isley M, Krauss G, Levin K, et al: Electromyography/Electroencephalography. Redford, WA, Spacelabs Medical, 1993, p 40.)
Numerous parameters are assessed with each CMAP obtained, including amplitude, latency, and conduction velocity (Fig. 13–3). The CMAP amplitude represents the number of nerve fibers that responded to the stimulus and are capable of conducting impulses to the recorded muscle.1,2 It is measured from baseline to negative peak (negative being up) and reported in millivolts. The latency is the time interval between the instant the nerve was stimulated and the onset of CMAPs; these are reported in milliseconds. The conduction velocity is the speed of transmission over the fastest conducting nerve fibers assessed and is reported in meters per second. Conduction velocities are calculated by dividing the distance traveled along a nerve segment (as determined by surface measurements) by the latency difference between the responses to proximal and distal stimulation. Normal conduction velocity in the upper limb is greater than 50 m/sec; in the lower limb, it is greater than 40 m/sec.
Sensory Nerve Conduction Studies
For sensory NCS, a sensory nerve or the sensory component of a mixed nerve is stimulated at one point with recording electrodes placed distally, usually on the fingers or on the ankle with routine studies. This stimulation results in a sensory nerve action potential (SNAP), which is a biphasic or triphasic waveform that represents summated nerve action potentials. In contrast to CMAPs, which are generated by muscle fibers and are measured in millivolts, SNAPs are generated directly by the nerve fibers. SNAPs are 100 times smaller and are measured in microvolts. Generally, only two sensory NCS measurements are reported: (1) the amplitude, which is the height of the response measured from baseline to negative peak and represents the number of sensory axons that depolarize, and (2) the peak latency, which is the time interval between the moment the nerve was stimulated and the negative peak of the response, reported in milliseconds (see Fig. 13–3).1
Late Responses (H Responses and F Waves)
The H response is the electrophysiologic correlate of the Achilles tendon reflex and is named after Hoffmann, who first described it in 1918. To obtain the H response, the tibial nerve is stimulated in the popliteal fossa using low voltage to activate sensory fibers (as opposed to motor fibers), which carry the nerve impulse proximally to the spinal cord (Fig. 13–4). The fibers synapse there with motor neuron cells to complete a monosynaptic reflex arc. The nerve impulse travels down the motor efferent nerve to the gastrocnemius where the recording electrode captures the response. Although the amplitude and the latency of the H response are analyzed, the amplitude is more reliable for diagnostic purposes in the authors’ laboratory.
Needle Electrode Examination
At-Rest Phase
During the at-rest phase, electrical silence ordinarily is noted. Various types of spontaneous activity may appear, however, with neuromuscular pathology. Only three of these are relevant to spine-related nerve disease: fibrillation potentials, fasciculation potentials, and complex repetitive discharges.2–4
Fibrillation potentials are the most reliable and objective manifestation of active or recent motor axon loss. They can be neither produced nor abolished voluntarily by the patient. They are very sensitive indicators of such loss because the degeneration of a single motor axon can result in hundreds of individual muscle fibers fibrillating within a given muscle, depending on the innervation ratio of the latter. Fibrillation potentials objectively can show that motor axon loss has occurred, when the lesion is far too mild in degree to produce clinical muscle weakness, atrophy, or loss of CMAP amplitude on motor NCS.3 Showing fibrillation potentials in a myotome distribution has been the principal method of identifying root lesions in the electrodiagnostic laboratory for more than half a century.5,6
Complex repetitive discharges are produced when a single muscle fiber is depolarized and that depolarization is spread by ephaptic transmission to adjacent muscle fibers, which reactivate the initial muscle fiber. A recurrent cycle of firing is established. These potentials have a bizarre configuration and fire at high frequency. For many years, they were known as bizarre high-frequency discharges. Although they are abnormal, they are nonspecific, being seen with neuropathic and myopathic disorders. Generally, they appear when there is grouped atrophy (i.e., denervation, reinnervation, and subsequent denervation) and are evidence of chronicity. Although these potentials are not helpful in localization, they are frequently encountered on NEE of the cervical paraspinal muscles in patients with chronic cervical root lesions.3
Activation Phase
Recruitment
Reduced MUP recruitment, also known as a neurogenic MUP firing pattern, is observed whenever numerous motor units in the muscle being sampled cannot be activated on maximal effort because either conduction block or axon loss affects their axons. The fewer MUPs seen on maximal effort, the weaker the muscle is clinically. MUPs that are capable of firing are noted to do so in decreased numbers and often faster than their basal firing rate of 5 to 10 Hz.3,7 The rapid rate of firing of the still functioning motor units is important because, similar to fibrillation potentials, it is unequivocal evidence of involuntary interruption of motor axon impulse transmission. Conversely, if the muscle was weak because of an upper motor neuron lesion or because voluntary effort was simply submaximal (e.g., because of malingering or pain on activation), incomplete MUP activation would be seen—that is, MUPs would fire in equally decreased numbers but at a slow to moderate rate.
Morphology
Chronic neurogenic MUP changes generally develop about 4 to 6 months after an axon loss injury has occurred because it takes this much time for such configurational remodeling to occur. After chronic neurogenic MUP changes develop, they can persist indefinitely. With many remote, proximal neurogenic lesions (e.g., radiculopathies and particularly poliomyelitis), they are the sole electrical residuals detected during the entire electrodiagnostic examination.3,7,8
Electrodiagnostic Findings in Radiculopathy
The electrodiagnostic examination has been used to assess patients with possible radiculopathies for more than 50 years. Root lesions were one of the first focal peripheral nerve fiber disorders for which the diagnostic utility of NEE was shown.5,6 For many years, lumbosacral radiculopathies were the most common reason for referral to the electrodiagnostic laboratory.9,10 Although several other electrodiagnostic procedures have been introduced over the past half-century, NEE remains the mainstay for diagnosing radiculopathies. The amplitudes of motor NCS are also helpful when root damage is severe, extensive, or both.8,9