Chapter 2 The Cardiac Catheterization Laboratory
CATHETERIZATION LABORATORY FACILITIES
Room Setup/Design/Equipment
The setup and design for the cardiac catheterization laboratory vary from a single room, as seen in a mobile catheterization laboratory or a small community hospital, to a multilaboratory facility, as is found in large tertiary care centers (Box 2-1). In these facilities with multiple laboratories, a central work area is needed to coordinate patient flow to each of the surrounding laboratories and for centralized equipment storage. Patient holding areas are used for observation and evaluation of patients before and after the procedure.
Physician Credentialing
The more experience an operator has with a particular procedure, the more likely this procedure will have a good outcome. The American College of Cardiology Task Force has established guidelines for the volume of individual operators in addition to the facility volumes mentioned earlier. The current recommendations for competence in diagnostic cardiac catheterization require a fellow perform a minimum of 300 angiographic procedures, with at least 200 catheterizations as the primary operator, during his or her training.1
PATIENT SELECTION FOR CATHETERIZATION
Indications for Cardiac Catheterization in the Adult Patient
Table 2-1 lists generally agreed on indications for cardiac catheterization. With respect to CAD, approximately 15% of the adult population studied will have normal coronary arteries. Coronary angiography is, for the moment, still consideredthe gold standard for defining CAD. With advances in magnetic resonance imaging and multislice computed tomography, the next decade may well see a further evolution of the catheterization laboratory to an interventional suite with fewer diagnostic responsibilities.
Table 2-1 Indications for Diagnostic Catheterization in the Adult Patient
Coronary Artery Disease |
Symptoms |
Unstable angina |
Postinfarction angina |
Angina refractory to medications |
Typical chest pain with negative diagnostic testing |
History of sudden death |
Diagnostic Testing |
Strongly positive exercise tolerance test |
Early positive, ischemia in > 5 leads, hypotension, ischemia present for > 6 minutes of recovery |
Positive exercise testing following after myocardial infarction |
Strongly positive nuclear myocardial perfusion test |
Increased lung uptake or ventricular dilation after stress |
Large single or multiple areas of ischemic myocardium |
Strongly positive stress echocardiographic study |
Decrease in overall ejection fraction or ventricular dilation with stress |
Large single area or multiple or large areas of new wall motion abnormalities |
Valvular Disease |
Symptoms |
Aortic stenosis with syncope, chest pain, or congestive heart failure |
Aortic insufficiency with progressive heart failure |
Mitral insufficiency or stenosis with progressive congestive heart failure symptoms |
Acute orthopnea/pulmonary edema after infarction with suspected acute mitral insufficiency |
Diagnostic Testing |
Progressive resting LV dysfunction with regurgitant lesion |
Decreased LV function and/or chamber dilation with exercise |
Adult Congenital Heart Disease |
Atrial Septal Defect |
Age > 50 with evidence of coronary artery diseaseSeptum primum or sinus venosus defects |
Ventricular Septal Defect |
Catheterization for definition of coronary anatomy |
Coarctation of the Aorta |
Detection of collateral vessels |
Coronary arteriography if increased age and/or risk factors are present |
Other |
Acute myocardial infarction therapy—consider primary PCI |
Mechanical complication after infarction |
Malignant cardiac arrhythmias |
Cardiac transplantation |
Pretransplant donor evaluation |
Post-transplant annual coronary artery graft rejection evaluation |
Unexplained congestive heart failure |
Research studies with institutional review board review and patient consent |
LV = left ventricular.
CARDIAC CATHETERIZATION PROCEDURE
Right-Sided Heart Catheterization
In the cardiac catheterization laboratory, right-sided heart catheterization is performed for diagnostic purposes. The routine use of right-sided heart catheterization during standard left-sided heart catheterization was studied by Hill and coworkers. Two hundred patients referred for only left-sided heart catheterization for suspected CAD underwent right-sided heart catheterization. This resulted in an additional 6 minutes of procedure time and 90 seconds of fluoroscopy. Abnormalities were detected in 35% of the patients. However, management was altered in only 1.5% of the patients. With this in mind, routine right-sided heart catheterization cannot be recommended. Box 2-2 outlines acceptable indications for right-sided heart catheterization during left-sided heart catheterization.
Diagnostic Catheterization Complications
Complications are related to multiple factors, but severity of disease is important. Mortality rates are shown in Table 2-2. Complications are specific for both right- and left-heart catheterization (Table 2-3). Although advances in technology continue, these complication rates are still present today, most likely due to the higher risk patient undergoing catheterization.
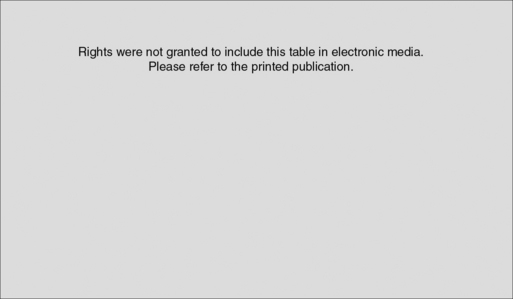
Table 2-2 Cardiac Catheterization Mortality Data
Rights were not granted to include this table in electronic media. Please refer to the printed book.
From Pepine CJ, Allen HD, Bashore TM, et al: ACC/AHA guidelines for cardiac catheterization and cardiac catheterization laboratories. Circulation Nov, 84(5): 2213–2247
Definition of Pressure Waveforms—Cardiac Cycle
Right-Sided Heart Pressures
The right-sided heart pressures, as measured in the cardiac catheterization laboratory, consist of the central venous pressure (CVP) or right atrial (RA) pressure (RAP), right ventricular (RV) pressure (RVP), PAP, and PCWP. The CVP consists of three waves and two descents (Fig. 2-1, Box 2-3). The A wave occurs synchronously with the Q wave of the ECG and accompanies atrial contraction. Next, a smaller C wave appears, which results from tricuspid valve closure and bulging of the valve into the right atrium as the right ventricle begins to contract. After this, with the tricuspid valve in the closed position, the atrium relaxes, resulting in the X descent. This is followed by the V wave, which corresponds to RA filling that occurs during RV systole with a closed tricuspid valve. As the RV relaxes, the RVP then becomes less than the RAP, the tricuspid valve opens, and the atrial blood rapidly empties into the ventricle. This is signified by the Y descent.
Left-Sided Heart Pressures
The LA, LV, aortic, and peripheral pressures are commonly measured in the cardiac catheterization laboratory. The LAP can be measured directly if a transseptal catheter is placed. Because this is not commonly done, the PCWP is used to estimate LAP. The LAP has a very similar appearance (A, C, V waves; X, Y descent) to that in the RA, although the pressures seen are about 5 mm Hg higher. The A wave in the RA tracing is normally larger than the V wave whereas the opposite is true in the LA (or PCWP). The PCWP provides reasonable estimations of the LAP, although the waveform is often damped and also delayed in time compared with the LAP (Fig. 2-2).
LV pressure also has many similar characteristics to the RVP, although because this is a thick-walled chamber, the generated pressures are higher than those reached in the RV. The central aortic pressure displays a higher diastolic pressure than that seen in the ventricle due to the properties of resistance in the arterial tree and the presence of a competent aortic valve. The dicrotic notch, which signifies the aortic valve closure, is a prominent feature of the aortic pressure wave in the central aorta. As the site of pressure measurement moves more distally in the arterial tree, there is a progressive distortion of the arterial waveform, usually demonstrated as an increase in systolic pressure. This is thought to be due to the addition of the pressure wave of reflected waves from the elastic arterial wall. Summation of reflected pressure waves has been postulated as a contributing factor in aneurysm formation. Additionally, the rapid propagation of reflected waves along stiff arteries has been advanced as an explanation of the systolic hypertension seen in the elderly. Table 2-4 displays the range of normal pressures on the right and left side of the heart.
Valvular Pathology
Stenotic Lesions
Stated another way, for any given orifice size, the transvalvular pressure gradient is a function of the square of the transvalvular flow rate. For example, with mitral stenosis, as the valve area progressively decreases, a modest increase in the rate of flow across the valve causes progressively larger increases in the pressure gradient across the valve (Fig. 2-3).
ANGIOGRAPHY
Ventriculography
Determination of Ejection Fraction
Ventriculography is routinely performed in the single-plane 30-degree right anterior oblique (RAO) or biplane 60-degree left anterior oblique (LAO) and 30-degree RAO projections using 20 to 45 mL of contrast agent with injection rates of 10 to 15 mL/s (Box 2-4). Complete opacification of the ventricle without inducing ventricular extrasystole is necessary for accurate assessment during ventriculography. These premature contractions not only alter the interpretation of mitral regurgitation (MR) but also result in a false increase in the global ejection fraction (EF).
BOX 2-4 Angiography: Coronary Anatomy
The EF is a global assessment of ventricular function and is calculated as follows:
Abnormalities in Regional Wall Motion
Each wall segment is identified as outlined in Figure 2-4 for both the LAO and RAO projections. These segments correspond roughly to vascular territories.
Coronary Arteriography
Description of Coronary Anatomy
The left main coronary artery is 1 to 2.5 cm in length (Fig. 2-5). It bifurcates into the circumflex (CX) and left anterior descending (LAD) arteries. Occasionally, the CX and LAD arteries may arise from separate ostia or the left main artery may trifurcate, giving rise to a middle branch, the ramus intermedius, which supplies the high lateralventricular wall. Both septal perforators and diagonal branch vessels arise from the LAD artery, which is described as proximal, mid, and distal based on the location of these branch vessels. The proximal LAD artery is before the first septal and first diagonal branch; the mid LAD artery is between the first and second septal and diagonal branches; and the distal LAD artery is beyond the major septal and large diagonal vessels. The distal LAD artery provides the apical blood supply in two thirds of patients, with the distal right coronary artery (RCA) supplying the apex in the remaining one third.
Coronary Collaterals
Common angiographically defined coronary collaterals are described in Table 2-5. Although present at birth, these vessels become functional and enlarge only if an area of myocardium becomes hypoperfused by the primary coronary supply. Angiographic identification of collateral circulation requires both the knowledge of potential collateral source as well as prolonged imaging to allow for coronary collateral opacification.
Left Anterior Descending Coronary Artery (LAD) |
Right-to-Left |
Conus to proximal LAD |
Right ventricular branch to mid LAD |
Posterior descending septal branches at mid vessel and apex |
Left-to-Left |
Septal to septal within LAD |
Circumflex-OM to mid-distal LAD |
Circumflex Artery (Cx) |
Right-to-Left |
Posterior descending artery to septal perforator |
Posterior lateral branch to OM |
Left-to-Left |
Cx to Cx in AV groove (left atrial circumflex) |
OM to OM |
LAD to OM via septal perforators |
Right Coronary (RCA) |
Right-to-Right |
Kugels—proximal RCA to AV nodal artery |
RV branch to RV branch |
RV branch to posterior descending |
Conus to posterior lateral |
Left-to-Right |
Proximal mid and distal septal perforators from distal LAD OM to posterior lateral |
OM to AV nodal |
AV groove Cx to posterior lateral |
AV = atrioventricular; OM = obtuse marginal; RV = right ventricular.
INTERVENTIONAL CARDIOLOGY: PERCUTANEOUS CORONARY INTERVENTION
This section is designed to present the current practice of interventional cardiology (Box 2-5). Although begun by Andreas Gruentzig in September 1977 as percutaneous transluminal coronary angioplasty (PTCA), catheter-based interventions have dramatically expanded beyond the balloon to include a variety of percutaneous coronary interventions (PCIs). Worldwide, this field has expanded to include approximately 900,000 PCI procedures annually.
1977 | Percutaneous transluminal coronary angioplasty |
1991 | Directional atherectomy |
1993 | Rotational atherectomy |
1994 | Stents with extensive antithrombotic regimen |
1995 | Abciximab approved |
1996 | Simplified antiplatelet regimen after stenting |
2001 | Distal protection |
2003 | Drug-eluting stents |
General Topics for All Interventional Devices
Indications
Box 2-6 provides a summary of current clinical indications for PCI. Although initially reserved only for patients who were also suitable candidates for CABG, PCI is routinely performed in patients who are not candidates for CABG. In considering both the indications as well as the appropriateness of PCI, the physician must review the patient’s historical presentation, including functional class, treadmill results with or without perfusion data, and wall motion assessment.
Equipment and Procedure
Guide catheters are available in multiple shapes and sizes for coronary and graft access, device support, and radial artery entry. Guidewires offer more flexible tips for placement in tortuous vessels as well as stiffer shafts to allow for the support of the newer devices during passage within the vessel. Separate guidewire placement within branch vessels may be required for coronary lesions at vessel bifurcations (Fig. 2-6). In selecting the appropriate device for the lesion, quantitative angiography and/or intravascular ultrasound (IVUS) may be used to determine the size of the vessel and composition of the lesion.
Restenosis
The major gains in combating restenosis have been in the area of stenting. Intracoronary stents maximize the increase in lumen area during the PCI procedure and decrease late lumen loss by preventing recoil and negative remodeling. However, neointimal hyperplasia is enhanced owing to a “foreign body–like reaction” to the stents. Different stent designs as well as varying strut thickness lead to different restenosis rates. Systemic administration of antiproliferate drugs decreases restenosis but causes significant systemic side effects. Drug-eluting stents, with a polymer utilized to attach the antiproliferative drug to the stent, have shown the best results to date for decreasing restenosis.2
Anticoagulation
Thrombosis is a major component in acute coronary syndromes as well as acute complications during PCI; its management is in constant evolution (Box 2-7). During interventional procedures, the guide catheter, guidewire, and device in the coronary artery serve as nidi for thrombus. Additionally, most catheter interventions disrupt the vessel wall, exposing thrombogenic substances to blood. Table 2-6 summarizes the current anticoagulation agents utilized in the setting of PCI.
Outcomes: Success and Complications
In the 20 years of catheter-based interventional procedures, the marked improvement in success rates with simultaneous decreases in adverse events clearly reflects both the significant technologic advancement as well as increased operator experience. PCI was once considered successful with the luminal narrowing reduced to less than 50% residual stenosis. In current practice with stent placement, seldom is a residual stenosis greater than 20% accepted, and excellent stent expansion without edge dissection is required before termination of the procedure. The initial National Heart, Lung, and Blood Institute (NHLBI) PTCA registry from 1979 to 1983 reported a success rate of 61% and a major coronary event rate of 13.6%. The 1985 to 1986 NHLBI registry reported a success rate of 78%, with the incidence of acute myocardial infarction as 4.3% and the emergency CABG rate as 3.4%. In the stent era, success rates are over 90% and emergent surgery rates less than 1% in laboratories performing more than 400 PCIs.3
General Management for Failed Percutaneous Coronary Intervention
Several possible scenarios may result from a failed PCI (Box 2-8). First, the interventional procedure may not successfully open the vessel but no coronary injury has occurred; the patient often remains in the hospital until a CABG can be scheduled. The second type of patient has a patent vessel with an unstable lesion. This most often occurs when a dissection cannot be contained by stents but the vessel remains open. The third patient type has an occluded coronary vessel after a failed PCI with stenting either not an option or unsuccessful. In this instance, myocardial ischemia/infarction ensues dependent on the degree of collateralization. This patient most commonly requires emergent surgical intervention.
In addition, long delays due to not having a rapid surgical alternative will lead to increases in morbidity and mortality. The paradigm shift in cardiovascular medicine toward PCIs and away from surgery will be slowed if significant numbers of serious complications occur due to prolonged delays in moving the patient to surgery.4,5
Controversies in Interventional Cardiology
Therapy for Acute Myocardial Infarction: Primary Percutaneous Coronary Intervention Versus Thrombolysis
Thrombolytic therapywas introduced for patients with acute myocardial infarction in the 1970s (Box 2-9). The decades of the 1980s and 1990s have seen extensive multicenter trials comparing the benefits of (1) thrombolytic therapy versus no thrombolytic therapy, (2) one thrombolytic agent compared with another, (3) different adjunctive medications given with thrombolytic therapy (platelet glycoprotein inhibitors, LMWHs, direct thrombin inhibitors), and (4) thrombolytic therapy versus primary PCI (bringing the patient directly to the catheterization laboratory). Table 2-7 lists the currently available drugs used for thrombolytic therapy in patients with acute myocardial infarction.
The recently published guidelines by the ACC/AHA on management of patients with ST-segment elevation myocardial infarction emphasize early reperfusion and discuss the choice between thrombolytic therapy and primary PCI.6 If a patient presents within 3 hours of symptom onset, the guidelines express no preference for either strategy with the following caveats: Primary PCI is preferred if (1) door-to-balloon time is less than 90 minutes and is performed by skilled personnel (operator annual volume > 75 cases with 11 primary PCI, and laboratory volume > 200 cases with 36 primary PCI); (2) thrombolytic therapy is contraindicated; and (3) the patient is in cardiogenic shock. Thrombolytic therapy should be considered if symptom onset is less than 3 hours and door to balloon time is more than 90 minutes. Patients older than age 75 years should be individually assessed, because they have a higher mortality from the myocardial infarction but a higher risk of complications, particularly intracranial bleeding, with thrombolytic therapy.
Therapy for acute myocardial infarction is evolving. With encouraging results from PCI in experienced hands when a facility is immediately available, more centers are considering acute primary PCI as standard of care, some in catheterization laboratories without operating room backup.7 Many patients present late or undergo thrombolytic therapy. If such patients are hemodynamically or electrically unstable, or if they have recurrent symptoms, a consensus would favor catheterization and revascularization. If such patients are stable, their management is controversial, although many cardiologists in the United States would recommend catheterization and revascularization.
PCI VERSUS CABG
The choice of therapy for multivessel CAD must be made by comparing PCI with CABG. In the mid 1980s, when PCI consisted only of balloon PTCA, the first comparisons of catheter intervention to CABG were begun. By the early to mid 1990s, nine randomized clinical trials had been published comparing PTCA with CABG in patients with significant CAD. Only the Bypass Angioplasty RevascularizationInvestigation (BARI) trial was statistically appropriate for assessing mortality. These results are summarized in Figure 2-7. The conclusions of these studies included similarities between the two approaches with respect to relief of angina and 5-year mortality. Costs were initially lower in the PCI group, but by 5 years they had converged because of repeat PCI procedures precipitated by restenosis, which occurred in 20% to 40% of the PCI group.8
To address the changes in PCI and CABG therapy, four more randomized trials were undertaken, and these are included in Figure 2-7. The results of these newer studies were similar to the results of the earlier ones. In the arterial revascularization therapy study (ARTS) trial, diabetic patients had poorer outcomes with PCI. Repeat procedures, although higher in the PCI group at 20%, were significantly lower than with the earlier trials. CABG patients also had improved outcomes; for instance, cognitive impairment occurred in fewer patients in the recent studies. A meta-analysis of all 13 randomized trials identified a 1.9% absolute survival advantage at 5 years in the CABG patients, but no significant difference at 1, 3, or 8 years.9 As with the first generation of PCI versus CABG trials, the second-generation trials were outdated before publication due to the advent of the drug-eluting stents. The ARTS II and BARI II trials are now in progress and will address this issue.
In conclusion, the physician must weigh the data and explain the advantages and disadvantages of both techniques to each patient. CABG offers a more complete revascularization with survival advantages in selective groups and a decreased need for repeat procedures. The disadvantages of a CABG are the higher early risk, longer hospitalization and recovery, initial expense, increased difficulty of second procedures, morbidity associated with leg incisions, and limited durability of venous grafts. The current high cost of drug-eluting stents will negate the initial cost advantage of PCI if multiple stents are used. From the perspective of a hospital administrator in the United States, current reimbursement policies favor CABG over the placement of multiple drug-eluting stents.10
SPECIFIC INTERVENTIONAL DEVICES
Interventional Diagnostic Devices
IVUS is the only method by which the vessel wall of the coronary artery can be visualized in vivo. A miniature transducer mounted on the tip of a 3-Fr catheter is advanced over the standard guidewire into the coronary artery. The IVUS transducer is about 1 mm in diameter with frequencies of about 30 MHz. These high frequencies allow for excellent resolution of the vessel wall. By comparison, contrast angiography images only the lumen, with the status of the vessel wall inferred from the image of the lumen.11 IVUS is useful in evaluating equivocal left main lesions, ostial stenoses, and vessels overlapping angiographically (Fig. 2-8). IVUS is superior to angiography in the early detection of the diffuse, immune-mediated arteriopathy of cardiac transplant allografts.
Atherectomy Devices: Directional and Rotational
Atherectomy devices are designed to remove some amount of plaque or other material from an atherosclerotic vessel. Of these devices, directional coronary atherectomy (DCA; Guidant Corporation, Indianapolis, IN) became the first nonballoontechnology to gain U.S. Food and Drug Administration (FDA) approval, in 1991. DCA removes tissue from the coronary artery, thus “debulking” the area of stenosis utilizing a low-pressure balloon located on one side of the metal housing, which, when inflated, forces tissue into an elliptical opening on the opposite side of the housing. A cylindrical cutting blade shaves the tissue and stores it in the distal nose cone of the device. Although tissue removal is an attractive concept, application of DCA was limited by the need for large (9.5 to 11 Fr) guiding catheters with early devices. Trials comparing DCA with PTCA did not show improved angiographic restenosis rates, and higher rates of acute complications were seen with DCA. Newer iterations of the device can be used with smaller (7 to 8 Fr) guide catheters. DCA is used infrequently in most institutions because its clinical benefit is inconclusive.12
Intracoronary Laser
Excimer laser coronary angioplasty (ELCA) (Spectranetics, Colorado Springs, CO) uses xenon chloride (XeCl) and operates in the ultraviolet range (308 nm) to photochemically ablate tissue. Currently, ELCA is indicated for use in lesions that are long (>2 mm in length), ostial, in saphenous vein bypass grafts, and unresponsive to PTCA. With the development of the eccentric directional laser, treatment of eccentric or bifurcation lesions can be approached with increased success. Also, in-stent restenosis can be effectively treated with the excimer laser.13 The Prima FX laser wire (Spectranetics, Colorado Springs, CO) is a 0.018-inch wire with the ability to deliver excimer laser energy to areas of chronic, total occlusion. With conventional equipment, failure to cross such lesions with a guidewire is frequent. The Prima FX has CE mark approval in Europe but is investigational in the United States. The optimal wavelength for the treatment of coronary atheroma has yet to be determined.
Intracoronary Stent
The use of intracoronary stents exploded during the mid 1990s (Box 2-10). Receiving FDA approval in April 1993, the Gianturco-Roubin (Cook Flex stent), a coiled balloon-expandable stent was approved for the treatment of acute closure after PCI. Use of the Gianturco-Roubin stent was limited by difficulties with its delivery and high rates of restenosis. The first stent to receive widespread clinical application was the Palmaz-Schatz (Johnson and Johnson, New Brunswick, NJ) tubular slotted stent approved for the treatment of de novo coronary stenosis in 1994. Throughout the 1990s, multiple stents were introduced with improved support and flexibility and thinner struts, resulting in improved delivery and decreased restenosis rates.
Rapamycin, a macrolide antibiotic, is a natural fermentation product produced by Streptomyces hygroscopicus, which was originally isolated in a soil sample from Rapa Nui (Easter Island). Rapamycin was soon discovered to have potent immunosuppressant activities, making it unacceptable as an antibiotic but attractive for prevention of transplant rejection. Rapamycin works through inhibition of a protein kinase called the mammalian target of rapamycin (mTOR), a mechanism that is distinct from other classes of immunosuppressants. Because mTOR is central to cellular proliferation as well as immune responses, this agent was an inspired choice for a stent coating. The terms rapamycin and sirolimus are often used interchangeably. A metal stent does not hold drugs well and permits little control over their release. These limitations required that polymers be developed to attach a drug to the stent and to allow the drug to slowly diffuse into the wall of the blood vessel, while eliciting no inflammatory response.14 The development of drug-eluting stents would not have been possible without these (proprietary) polymers. This led to the true revolution in PCI, which occurred with the approval in April 2003 of the first drug-eluting stent. Johnson and Johnson/Cordis introduced their Cypher stent. This is their Velocity stent and polymer, which elutes rapamycin over 14 days; the drug is completely gone by 30 days post implantation.
The RAVEL trial randomized 238 patients to receive either a sirolimus-eluting stent (SES) or a bare metal stent. Remarkably, there was no restenosis in the group that received a sirolimus-eluting stent. The SIRIUS trial randomized 1058 patients to a sirolimus-eluting stent or a bare metal stent. At 9 months, restenosis rates were 8.9% in the sirolimus-eluting stent group and 36.3% in the bare metal stent group, with no difference in adverse events. Clinically driven repeat procedures were required in 3.9% and 16.6%, respectively. This benefit was sustained, if not slightly improved, at 12 months. Although initially approved only for use in de novo lesions in native vessels of stable patients, subsequent publications have shown similar benefits in every clinical scenario that has been studied.15 Initial concerns regarding subacute stent thrombosis have proved unjustified with the rate of thrombosis approximately 1%, equal to that seen in bare metal stent patients.
Currently, stents are placed at the time of most PCI procedures, if the size and anatomy of the vessel permit. There are several reasons not to use a drug-eluting stent in every procedure. First, drug-eluting stents are available in fewer sizes. Second, a longer course of thienopyridine is required, and this may not be desirable if, for instance, a surgical procedure is urgently needed. Stent thromboses, myocardial infarctions, and deaths have been reported when antiplatelet therapy is interrupted. Finally, the cost of a drug-eluting stent is about three times that of a bare metal stent, and this increment is not fully reflected in reimbursement. As additional drug-eluting stents reach the market, prices may decline. With the significant reduction in restenosis, the drug-eluting stent may give PCI an advantage over CABG in multivessel disease. The consequences of this may be dramatic, as hospitals (and cardiac surgeons and cardiac anesthesiologists) see reduced CABG volumes and reduced volumes of repeat PCI in restenotic vessels. If these profitable procedures are replaced by money-losing ones, as placement of multiple drug-eluting stents currently is, many hospitals will suffer.16
Intravascular Brachytherapy
Brachytherapy, using either a gamma or beta emitter, has proved effective for the treatment of in-stent restenosis. After brachytherapy, clopidogrel must be continued for at least 6 to 12 months to prevent late stent thrombosis that occurs due to delayedendothelialization of the stent. The future for brachytherapy in the era of drug-eluting stents is unknown.17 The drug-eluting stent has significantly decreased in-stent restenosis. If restenosis does occur with drug-eluting stents, whether brachytherapy should be undertaken or a repeat drug-eluting stent placement performed is unclear. Because of the complexity of brachytherapy, unless it is truly proved superior to other modalities, its use in the interventional suite will be limited.
OTHER CATHETER-BASED PERCUTANEOUS THERAPIES
Percutaneous Valvular Therapy
Mitral Balloon Valvuloplasty
All patients must undergo transesophageal echocardiography to exclude LA thrombus as well as transthoracic echocardiography to classify the patient by anatomic groups. The most widely used classification, the Wilkins score, addresses leaflet mobility, valve thickening, subvalvular thickening, and valvular calcification. These scoring systems, as well as operator experience, predict outcomes. In experienced hands, the procedure is successful in 85% to 99% of cases. Risks of PMC include a procedural mortality of 0% to 3%, hemopericardium in 0.5% to 12%, and embolism in 0.5% to 5%. Severe MR occurs in 2% to 10% of procedures and often requires emergent surgery.18 Although peripheral embolization occurs in up to 4% of patients, long-term sequelae are rare.
Percutaneous Valve Replacement
The first percutaneous aortic valve replacement in humans was performed in France in 2002. This valve is created by shaping bovine pericardium into leaflets and mounting them within a balloon-expandable stent. Both retrograde and antegrade approaches have been used. Early results are encouraging, as improvements in symptoms and ventricular function are seen after percutaneous aortic valve replacement.19
THE CATHETERIZATION LABORATORY AND THE ANESTHESIOLOGIST
The objective of this chapter has been to provide a broad overview of the catheterization laboratory for the anesthesiologist. As success rates for coronary interventions have increased and complication rates have decreased, there have been fewer opportunities for the cardiologist and the anesthesiologist to interact in the catheterization suite. However, in the 21st century, the role of the anesthesiologist in the catheterization laboratory is destined to change. In this dynamic field of interventional cardiology, more complex and prolonged procedures, such as percutaneous valvular therapy, may well require the renewed collaboration of the interventional cardiologist and the cardiac anesthesiologist.20
SUMMARY
1. Hirshfeld J.W., Balter S.Jr., Brunker J.A., et al. ACC Clinical Competence Statement. Recommendations for the assessment and maintenance of proficiency in coronary interventional procedures. Statement of the American College of Cardiology. J Am Coll Cardiol. 1998;31:722.
2. Sousa J.E., Serruys P.W., Costa M.A. New frontiers in cardiology: Drug-eluting stents: I. Circulation. 2003;107:2274.
3. Williams D.O., Holubkov R., Yeh W., et al. Percutaneous coronary intervention in the current era compared with 1985. The National Heart, Lung, and Blood Institute Registries. Circulation. 2000;102:2945.
4. Holmes D.R., Firth B.G., Wood D.L. Paradigm shifts in cardiovascular medicine. J Am Coll Cardiol. 2004;43:507.
5. Lotfi M., Mackie K., Dzavik V., Seidelin P.H. Impact of delays to cardiac surgery after failed angioplasty and stenting. J Am Coll Cardiol. 2004;43:337.
6. Antman E.M., Anbe D.T., Armstrong P.W., et al. ACC/AHA guidelines for the management of patients with ST-elevation myocardial infarction: executive summary. Circulation. 2004;110:588.
7. Waters R.E., Singh K.P., Roe M.T., et al. Rationale and strategies for implementing community-based transfer protocols for primary percutaneous coronary intervention for acute ST-segment elevation myocardial infarction. J Am Coll Cardiol. 2004;43:2153.
8. Casey C., Faxon D.P. Multi-vessel coronary disease and percutaneous coronary intervention. Heart. 2004;90:341.
9. Hoffman S.N., TenBrook J.A., Wolf M.P., et al. A meta-analysis of randomized controlled trials comparing coronary artery bypass graft surgery with percutaneous transluminal coronary angioplasty: One- to eight-year outcomes. J Am Coll Cardiol. 2003;41:1293.
10. Holmes D.R. Stenting small coronary arteries: Works in progress. JAMA. 2004;292:2777.
11. vonBirgelen C., Hartmann M., Mintz G.S., et al. Relationship between cardiovascular risk as predicted by established risk scores versus plaque progression as measured by serial intravascular ultrasound in left main coronary arteries. Circulation. 2004;110:1579.
12. Tsuchikane E., Sumitsuji S., Awata N., et al. Final results of the Stent versus Directional Coronary Atherectomy Randomized Trial (START). J Am Coll Cardiol. 1999;34:1050.
13. Mehran R., Dangas G., Mintz G.S., et al. Treatment of in-stent restenosis with excimer laser coronary angioplasty versus rotational atherectomy: Comparative mechanisms and results. Circulation. 2000;101:2484.
14. Serruys P., Kutryk M., Ong A. Coronary artery stents. N Engl J Med. 2006;354:486.
15. Lemos P.A., Saia F., Hofma S.H., et al. Short- and long-term clinical benefit of sirolimus-eluting stents compared with conventional bare stents for patients with acute myocardial infarction. J Am Coll Cardiol. 2004;43:704.
16. Lemos P.A., Serruys P.W., Sousa J.E. Drug-eluting stents: Cost versus clinical benefit. Circulation. 2003;107:3003.
17. Teirstein P.S., King S. Vascular radiation in a drug-eluting stent world: It’s not over til it’s over. Circulation. 2003;108:384.
18. Vahanian A., Palacios I.F. Percutaneous approaches to valvular disease. Circulation. 2004;109:1572.
19. Bauer F., Eltchaninoff H., Tron C., et al. Acute improvement in global and regional left ventricular systolic function after percutaneous heart valve implantation in patients with symptomatic aortic stenosis. Circulation. 2004;110:1473.
20. O’Neill W., Dixon S., Grimes C. The year in interventional cardiology. J Am Coll Cardiol. 2005;45:1017.