CHAPTER 42 The back
Most clinical disorders of the back present as low back pain with or without associated lower limb pain, so historically most attention has been paid to the anatomy of the lower (lumbosacral) back. In this Section, the term ‘the back’ will include the whole of the posterior aspect of the trunk and of the neck. The whole of this region has great clinical importance but its anatomy has often been neglected. Recent understanding of the detailed topography of the bony and soft-tissue elements of the lower back owes much to the work of Bogduk (2005).
The soft tissues of the back of the trunk and neck include the skin and subcutaneous fat, the underlying fascial layers and the musculature. The deep, ‘true’ or epaxial muscles lie within compartments in their own fascial ‘skeleton’. The bony framework to which the muscles and fasciae attach includes not only elements of the axial skeleton, i.e. the vertebral column and occiput, but also elements of the pectoral and pelvic girdles as well as the ribs. The occiput is described below, the scapula on page 793, the ribs on page 918 and the pelvis on page 1352.
SKIN
The skin of the back of the trunk is thick and highly protective, but has low discriminatory sensation. The superficial fascia is thick and fatty in most areas of the back. Its attachment to the deeper fascial layers is strong in the midline, especially in the neck, but becomes weaker more laterally. The skin of the back of the neck is thicker than that of the front of the neck, but thinner than that of the back of the trunk. The quantity, texture and distribution of hair vary with sex, race and the individual, though well-defined hair tracts have been delineated (Fig. 42.1).
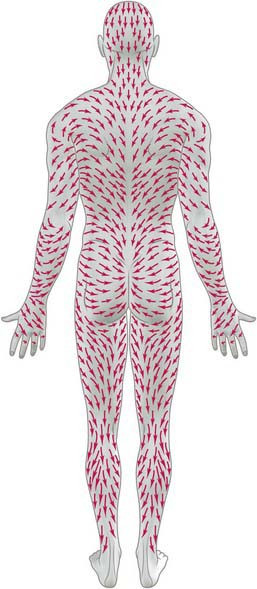
Fig. 42.1 Hair tracts on the dorsal surface of the body.
(Redrawn by permission from Wood Jones F (ed) 1949 Buchanan’s Manual of Anatomy, 8th edn. London: Baillière Tindall and Cox.)
Lines of skin tension run horizontally in the cervical and lumbosacral regions but form segments of two adjacent circles in the thoracic region (Fig. 42.2).
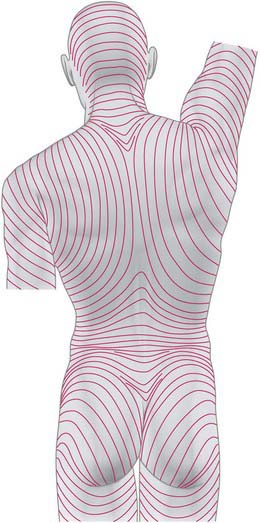
Fig. 42.2 Lines of skin tension on the dorsum of the trunk and head.
(From Kraissl CL, Plast Reconstruct Surg 8: 1–28, 1951. By permission from Lippincott Williams and Wilkins.)
CUTANEOUS INNERVATION AND DERMATOMES
The skin of the back of the neck and trunk is innervated by the dorsal (posterior primary) rami of the spinal nerves (see Fig. 43.6 and pp. 755–756 where dorsal rami are covered in detail). In the cervical and upper thoracic regions (down to T6) skin is supplied by the medial branches of these rami, while in the lower thoracic, lumbar and sacral regions it is supplied by the lateral branches. The total area supplied by these dorsal rami is shown in Fig. 43.6. The spinal nerves involved include C2 to C5, T2 to L3, S2 to S4, and Co1. The pattern of their dermatomes is shown in Fig. 42.3. There is about half a segment of overlap between these cutaneous ‘strips’: the strips supplied by the dorsal rami do not correspond exactly to those served by ventral rami, and differ slightly in both width and position.
CUTANEOUS VASCULAR SUPPLY AND LYMPHATIC DRAINAGE
The skin of the back of the trunk receives its arterial blood supply mainly from musculocutaneous branches of posterior intercostal, lumbar and lateral sacral arteries (Fig. 42.4), which all accompany the cutaneous branches of their respective dorsal rami. In addition, there is a supply from the dominant vascular pedicles of the superficial (extrinsic) back muscles. The skin over the scapula is supplied by branches of the suprascapular, dorsal scapular and subscapular arteries. The skin of the back of the neck is supplied mainly from the occipital and deep cervical arteries. The superficial cervical or transverse cervical artery supplies the skin of the lower part of the back of the neck (see Cormack & Lamberty 1994 in the Bibliography).
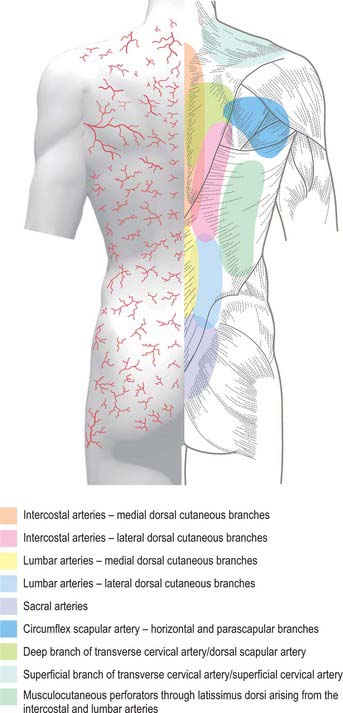
Fig. 42.4 Areas of cutaneous arterial supply on the dorsum of the trunk.
(Redrawn with permission from Cormack GC, Lamberty BGH 1994 The Arterial Anatomy of Skin Flaps. Edinburgh: Churchill Livingstone.)
Lymph from the skin of the back of the neck drains into occipital, lateral deep cervical and axillary nodes (Fig. 42.5). From the back of the trunk, drainage is to the posterior (subscapular) axillary nodes and to the lateral superficial inguinal nodes.
FASCIAL LAYERS
THORACOLUMBAR FASCIA
The thoracolumbar (lumbodorsal) fascia covers the deep muscles of the back and the trunk. Above, it passes anterior to serratus posterior superior and is continuous with the superficial lamina of the deep cervical fascia on the back of the neck. In the thoracic region the thoracolumbar fascia provides a thin fibrous covering for the extensor muscles of the vertebral column and separates them from the muscles connecting the vertebral column to the upper extremity. Medially it is attached to the spines of the thoracic vertebrae, and laterally to the angles of the ribs. In the lumbar region the thoracolumbar fascia is in three layers (Fig. 42.6, Fig. 42.7, Fig. 62.1). The posterior layer is attached to the spines of the lumbar and sacral vertebrae and to the supraspinous ligaments. The middle layer is attached medially to the tips of the lumbar transverse processes and the intertransverse ligaments, below to the iliac crest, and above to the lower border of the 12th rib and the lumbocostal ligament. The anterior layer covers quadratus lumborum and is attached medially to the anterior surfaces of the lumbar transverse processes behind the lateral part of psoas major; below, it is attached to the iliolumbar ligament and the adjoining part of the iliac crest; above, it forms the lateral arcuate ligament. The posterior and middle layers unite to form a tough raphe at the lateral margin of erector spinae, and at the lateral border of quadratus lumborum they are joined by the anterior layer to form the aponeurotic origin of transversus abdominis. At sacral levels, the posterior layer is attached to the posterior superior iliac spine and posterior iliac crest, and fuses with the underlying erector spinae aponeurosis. Bogduk (2005) describes two laminae in the posterior layer at lumbar levels, with varying orientation of the constituent collagen fibres relating to the biomechanical function of the fascia. The posterior and middle layers of the thoracolumbar fascia and the vertebral column together form an osteofascial compartment which encloses the erector spinae muscle group. The attachments of the fascia, especially those which give it continuity with the abdominal wall musculature, putatively give it an important role in lifting, though the exact details of this role remain controversial. The fascia may also play an important role in load transfer between the trunk and the limbs: its tension is affected by the actions of latissimus dorsi, gluteus maximus, and the hamstrings. An erector spinae compartment syndrome may be one cause of low back pain.
DEEP CERVICAL FASCIA
The investing layer of the deep cervical fascia forms the deep fascia of the posterior aspect of the neck (Fig. 28.4). It attaches in the midline to the external occipital protuberance, the ligamentum nuchae and the spine of the seventh cervical vertebra, and splits to enclose trapezius on each side. Inferiorly the posterior part of the investing layer attaches with trapezius to the spine and acromion of the scapula.
BONE
OCCIPITAL BONE
The occipital bone forms much of the back and base of the cranium (Fig. 42.8). It is trapezoid, internally concave and encloses the foramen magnum. It has four parts: basilar (basioccipital), which is the quadrilateral part in front of the foramen magnum; squamous, which is the expanded plate posterosuperior to the foramen; and two lateral (condylar or exoccipital), one on each side of the foramen magnum. The latter is anteromedian and wider behind, with an anteroposterior diameter greater than its transverse diameter.
Squamous part
The squamous part is an expanded plate posterosuperior to the foramen magnum, convex externally and concave internally. On its external surface the external occipital protuberance lies midway between its summit and the foramen magnum. On each side, two curved lines extend laterally from this protuberance. The upper, faintly marked and often almost imperceptible, is the highest nuchal line, and the lower is the superior nuchal line. The epicranial aponeurosis is attached to the medial part of the highest nuchal line. The median external occipital crest is often faint; it descends from the external occipital protuberance to the foramen magnum. On each side an inferior nuchal line spreads laterally from the midpoint of the crest.
The occipital part of occipitofrontalis is attached to the lateral part of the highest nuchal line (Fig. 42.8C). Trapezius attaches to the medial third of the superior nuchal line and to the external occipital protuberance. Sternocleidomastoid attaches to the lateral half of the superior nuchal line, with splenius capitis just below the lateral third of that line. Semispinalis capitis is attached to the medial part of the area between the superior and inferior nuchal lines; obliquus capitis superior attaches to the lateral part of this area. Rectus capitis posterior major attaches to the lateral part of the inferior nuchal line and to the bone immediately below, and rectus capitis posterior minor attaches to the medial part of the inferior nuchal line and to the bone between that line and the foramen magnum.
Ossification
At birth the occipital bone consists of four separate parts (Fig. 42.9), a basilar part, two lateral parts and a squamous part, all joined by cartilage and forming a ring around the foramen magnum. The squamous and lateral parts fuse together from the second year. The lateral parts fuse with the basilar part during years 3 and 4, but fusion may be delayed until the 7th year.
VERTEBRAL COLUMN
The vertebral column is a curved linkage of individual bones or vertebrae (Fig. 42.10). A continuous series of vertebral foramina runs through the articulated vertebrae posterior to their bodies, and collectively constitutes the vertebral canal, which transmits and protects the spinal cord and nerve roots, their coverings and vasculature (Fig. 42.11, 42.16). A series of paired lateral intervertebral foramina transmits the spinal nerves and their associated vessels between adjacent vertebrae. The linkages between the vertebrae include cartilaginous interbody joints and paired synovial facet (zygapophysial) joints, together with a complex of ligaments and overlying muscles and fasciae. The muscles directly concerned with vertebral movements and attached to the column lie mainly posteriorly. Several large muscles producing major spinal movements lie distant from the column and without direct attachment to it, e.g. the anterolateral abdominal wall musculature. Movements of the column and the muscles concerned are described on page 743. The column as a whole receives its vascular supply and innervation according to general anatomical principles which are considered below.
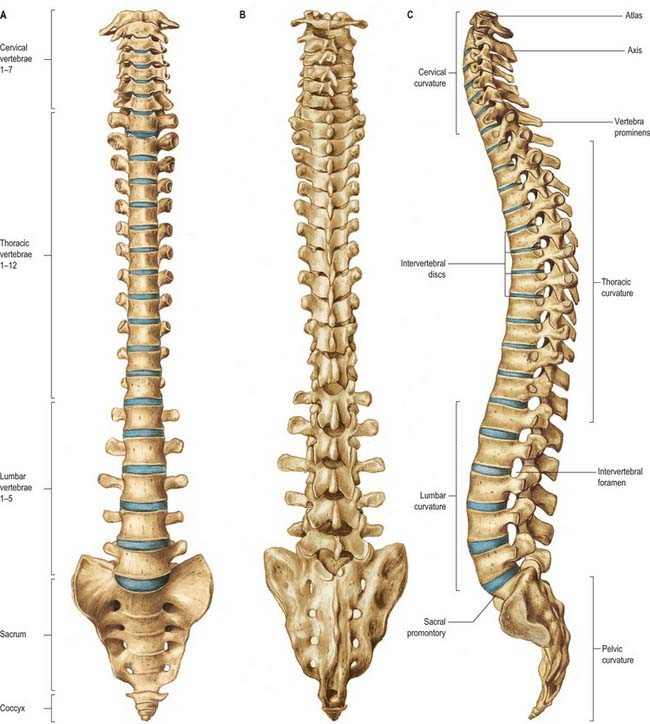
Fig. 42.10 The vertebral column: A, anterior aspect; B, posterior aspect; C, lateral aspect.
(From Sobotta 2006.)
The adult vertebral column usually consists of 33 vertebral segments. Each presacral segment (except the first two cervical) is separated from its neighbour by a fibrocartilaginous intervertebral disc. The functions of the column are to support the trunk, to protect the spinal cord and nerves, and to provide attachments for muscles. It is also an important site of haemopoiesis throughout life. Its total length in males is about 70 cm and in females about 60 cm. The intervertebral discs contribute about a quarter of this length in young adults, though there is some diurnal variation in this contribution (p. 744). Approximately 8% of overall body length is accounted for by the cervical spine, 20% by the thoracic, 12% by the lumbar and 8% by the sacrococcygeal regions. Although the usual number of vertebrae is 7 cervical, 12 thoracic, 5 lumbar, 5 sacral and 4 coccygeal, this total is subject to frequent variability, and there have been reports of variation between 32 and 35 bones. The demarcation of groups by their morphological characteristics may be blurred: thus there may be thoracic costal facets on the seventh cervical vertebra, giving it the appearance of an extra thoracic vertebra; lumbar-like articular processes may be found on the lowest thoracic vertebra; the fifth lumbar vertebra may be wholly or partially incorporated into the sacrum. As a result of these changes in transition between vertebral types, there may be 23–25 mobile presacral vertebrae.
Anterior aspect
The anterior aspect of the column is formed by the anterior surfaces of the vertebral bodies and of the intervertebral discs (Fig. 42.10A). It has important anatomical relations at all levels, and should be considered in continuity. It forms part of several clinically significant junctional or transitional zones, including the prevertebral/retropharyngeal zone of the neck, the thoracic inlet, the diaphragm and the pelvic inlet. The anterior aspect of the column is covered centrally by the anterior longitudinal ligament, which forms a fascial plane with the prevertebral and endothoracic fascia and with the subperitoneal areolar tissue of the posterior abdominal wall. Infection and other pathological processes may spread along this fascial plane.
Lateral aspect
The lateral aspect of the vertebral column is arbitrarily separated from the posterior by articular processes in the cervical and lumbar regions and by transverse processes in the thoracic region (Fig. 42.10C). Anteriorly it is formed by the sides of vertebral bodies and intervertebral discs. The oval intervertebral foramina, behind the bodies and between the pedicles, are smallest at the cervical and upper thoracic levels, and increase progressively in size in the thoracic and upper lumbar regions. The lumbosacral (L5/S1) intervertebral foramen is the smallest of the lumbar foramina. The foramina permit communication between the lumen of the vertebral canal and the paravertebral soft tissues (a ‘paravertebral space’ is sometimes described), which may be important in the spread of tumours and other pathological processes. The lateral aspects of the column have important anatomical relations, some of which vary considerably between the two sides.
Posterior aspect
The posterior aspect of the column is formed by the posterior surfaces of the laminae and spinous processes, their associated ligaments, and the facet joints (Fig. 42.10B). It is covered by the deep muscles of the back.
Curvatures
Embryonic and fetal curvatures
The embryonic body appears flexed. It has primary thoracic and pelvic curves which are convex dorsally. Functional muscle development leads to the early appearance of secondary cervical and lumbar spinal curvatures in the sagittal plane. The cervical curvature appears at the end of the embryonic period, and reflects the development of function in the muscles responsible for head extension, an important component of the ‘grasp reflex’. Radiographic examination of human fetuses aged from 8 to 23 weeks shows that the secondary cervical curvature is almost always present. Lumbar flattening has also been identified as early as the eighth week. Ultrasound investigations support the role of movement in the development of these curvatures. The early appearance of the secondary curves is probably accentuated by postnatal muscular and nervous system development at a time when the vertebral column is highly flexible and is capable of assuming almost any curvature.
Adult curvatures
The presence of these curvatures means that the cross-sectional profile of the trunk changes with spinal level. The anteroposterior diameter of the thorax is much greater than that of the lower abdomen. In the normal vertebral column there are well-marked curvatures in the sagittal plane and no lateral curvatures other than in the upper thoracic region, where there is often a slight lateral curvature, convex to the right in right-handed persons, and to the left in the left-handed. Compensatory lateral curvature may also develop to cope with pelvic obliquity such as that imposed by inequality of leg length. The sagittal curvatures are present in the cervical, thoracic, lumbar and pelvic regions (Fig. 42.10). These curvatures have developed with rounding of the thorax and pelvis as an adaptation to bipedal gait.
Vertebral column in the elderly
In mid-lumbar vertebrae the width of the body increases with age. In men there is a relative decrease of posterior to anterior body height, while in both sexes anterior height decreases relative to width. Twomey et al (1983) observed a reduction in bone density of lumbar vertebral bodies with age, principally as a result of a reduction in transverse trabeculae (more marked in females as a result of postmenopausal osteoporosis), which was associated with increased diameter and increasing concavity in their juxtadiscal surfaces (end-plates).
Vascular supply and lymphatic drainage
Arteries
The vertebral column, its contents and its associated soft tissues, all receive their arterial supply from derivatives of dorsal branches of the embryonic intersegmental somatic arteries (see Ch. 59). The named artery concerned depends on the level of the column. These intersegmental vessels persist in the thoracic and lumbar regions as the posterior intercostal and lumbar arteries. In the cervical and sacral regions, longitudinal anastomoses between the intersegmental vessels persist as longitudinal vessels which themselves give spinal branches to the vertebral column. In the neck the postcostal anastomosis becomes most of the vertebral artery, while the post-transverse anastomosis forms most of the deep cervical artery. The ascending cervical artery and the lateral sacral artery are persistent parts of the precostal anastomosis.
In the thorax and abdomen the primitive arterial pattern is retained by the paired branches of the descending aorta which supply the vertebral column (Fig. 42.12A). On each side, the main trunk of the artery (posterior intercostal or lumbar) passes around the vertebral body, giving off primary periosteal and equatorial branches to the body, and then a major dorsal branch. The latter gives off a spinal branch which enters the intervertebral foramen, before itself supplying the facet joints, the posterior surfaces of the laminae and the overlying muscles and skin. There is free anastomosis between these dorsal articular and soft-tissue branches, extending over several segments (Crock & Yoshizawa 1976; Boelderl et al 2002). At cervical and sacral levels the longitudinally running arteries described above have direct spinal branches. The spinal branches are the main arteries of supply to all bony elements of the vertebrae and to the dura and epidural tissues, and also contribute to the supply of the spinal cord and nerve roots via radicular branches. As they enter the vertebral canal the spinal arteries divide into postcentral, prelaminar and radicular branches. The postcentral branches, which are the main nutrient arteries to the vertebral bodies and to the periphery of the intervertebral discs, anastomose beneath the posterior longitudinal ligament with their fellows above and below as well as across the midline (Fig. 42.12A,B). This anastomosis also supplies the anterior epidural tissues and dura. The majority of the vertebral arch, the posterior epidural tissues and dura and the ligamentum flavum are supplied by the prelaminar branches and their anastomotic plexus on the posterior wall of the vertebral canal.
Veins
Veins of the vertebral column form intricate plexuses along the entire column, external and internal to the vertebral canal (Fig. 42.13). Both groups are devoid of valves, anastomose freely with each other, and join the intervertebral veins. Interconnections are widely established between these plexuses and longitudinal veins early in fetal life. When development is complete, the plexuses drain into the caval and azygos/ascending lumbar systems via named veins which accompany the arteries described above.
External vertebral venous plexuses
The external vertebral venous plexuses are anterior and posterior. They anastomose freely, and are most developed in the cervical region. Anterior external plexuses are anterior to the vertebral bodies, communicate with basivertebral and intervertebral veins, and receive tributaries from vertebral bodies. Posterior external plexuses lie posterior to the vertebral laminae and around spines, transverse and articular processes. They anastomose with the internal plexuses and join the vertebral, posterior intercostal and lumbar veins.
Internal vertebral venous plexuses
The internal vertebral venous plexuses occur between the dura mater and vertebrae, and receive tributaries from the bones, red bone marrow and spinal cord. (For the venous drainage of the spinal cord see p. 757 and Fig. 43.11.)
Intervertebral veins
The intervertebral veins accompany the spinal nerves through intervertebral foramina, draining the spinal cord and internal and external vertebral plexuses, and ending in the vertebral, posterior intercostal, lumbar and lateral sacral veins. Upper posterior intercostal veins may drain into the caval system via brachiocephalic veins, whereas the lower intercostals drain into the azygos system. Lumbar veins are joined longitudinally in front of the transverse processes by the ascending lumbar veins, in which they may terminate. Alternatively, they may proceed around the vertebral bodies to drain into the inferior vena cava. Whether the basivertebral or intervertebral veins contain effective valves is uncertain but experimental evidence strongly suggests that their blood flow can be reversed (Batson 1957). This may explain how pelvic neoplasms, e.g. carcinoma of the prostate, may metastasize in vertebral bodies: the cells spread into the internal vertebral plexuses via their connections with the pelvic veins when blood flow is temporarily reversed by raised intra-abdominal pressure or postural alterations.
Innervation
The innervation of the vertebral column and its associated soft tissues has been studied in greatest detail in the lumbar region (see below Fig. 42.44). The account given here relies particularly on the work of Bogduk, to whose textbook on the lumbosacral spine (Bogduk 2005) the interested reader is referred. See also the work of Groen et al (1990).
Innervation is derived from the spinal nerves where they branch, in and just beyond the intervertebral foramina. There is an input from the sympathetic system via either grey rami communicantes or directly from thoracic sympathetic ganglia. The branches of the spinal nerve concerned are the dorsal ramus and the recurrent meningeal or sinuvertebral nerves (usually more than one at each level) (p. 753 and also Fig. 43.5). The dorsal ramus branches to supply the facet joints, periosteum of the posterior bony elements, overlying muscles and skin. The exact origin and branching pattern of the sinuvertebral nerves is controversial, but they may be best considered to be recurrent branches of the ventral rami. They receive the sympathetic input described above, then re-enter the intervertebral foramina to supply the structures that form the walls of the vertebral canal, the dura and epidural soft tissues. Their subsequent course is described on page 754.
VERTEBRAE: GENERAL FEATURES
A typical vertebra has a ventral body, a dorsal vertebral (neural) arch, extended by lever-like processes, and a vertebral foramen, which is occupied in life by the spinal cord, meninges and their vessels (Fig. 42.14).
The cylindroid vertebral body varies in size, shape and proportions in different regions of the vertebral column. Its superior and inferior (discal) surfaces vary in shape from approximately flat (but not parallel) to sellar, with a raised peripheral smooth zone, formed from an ‘anular’ epiphysial disc, within which the surface is rough. These differences in texture reflect variations in the early structure of intervertebral discs. In the horizontal plane the profiles of most bodies are convex anteriorly, but concave posteriorly where they complete the vertebral foramen. Most sagittal profiles are concave anteriorly but flat posteriorly. Small vascular foramina appear on the front and sides, and posteriorly there are small arterial foramina and a large irregular orifice (sometimes double) for the exit of basivertebral veins (Fig. 42.15). The adult vertebral body is not coextensive with the developmental centrum but includes parts of the neural arch posterolaterally.
Pedicles are short, thick, rounded dorsal projections from the superior part of the body at the junction of its lateral and dorsal surfaces: the concavity formed by the curved superior border of the pedicle is shallower than the inferior one (Fig. 42.15). When vertebrae articulate by the intervertebral disc and facet joints, these adjacent vertebral notches contribute to an intervertebral foramen. The complete perimeter of an intervertebral foramen consists of the notches, the dorsolateral aspects of parts of adjacent vertebral bodies and the intervening disc, and the capsule of the synovial facet joint.
The thoracic transverse processes articulate with ribs, but at other levels the mature transverse process is a composite of ‘true’ transverse process and an incorporated costal element. Costal elements develop as basic parts of neural arches in mammalian embryos, but become independent only as thoracic ribs. Elsewhere they remain less developed and fuse with the ‘transverse process’ of descriptive anatomy (Fig. 44.11).
Vertebrae are internally trabecular, and have an external shell of compact bone perforated by vascular foramina (Fig. 42.15). The shell is thin on the superior and inferior body surfaces but thicker in the arch and its processes. The trabecular interior contains red bone marrow and one or two large ventrodorsal canals which contain the basivertebral veins.
Sexual dimorphism in vertebrae has received little attention, but Taylor & Twomey (1984) have described radiological differences in adolescent humans and have reported that female vertebral bodies have a lower ratio of width to depth. Vertebral body diameter has also been used as a basis for sex prediction in the analysis of skeletal material (MacLaughlin & Oldale 1992).
Vertebral canal
The vertebral canal (Fig. 42.16) extends from the foramen magnum to the sacral hiatus, and follows the vertebral curves. In the cervical and lumbar regions, which exhibit free mobility, it is large and triangular, but in the thoracic region, where movement is less, it is small and circular. These differences are matched by variations in the diameter of the spinal cord and its enlargements. In the lumbar region, the vertebral canal decreases gradually in size between L1 and L5, with a greater relative width in the female.
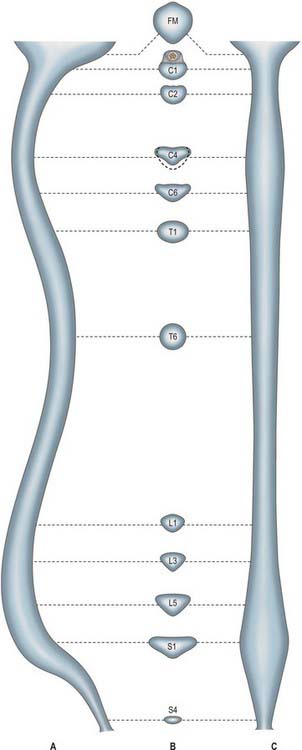
Fig. 42.16 The vertebral canal in section: A, sagittal; B, transverse (axial); C, coronal. FM: foramen magnum.
For clinical purposes it is useful to consider the vertebral canal as having three zones. These are a central zone, between the medial margins of the facet joints, and two lateral zones, beneath the facet joints and entering the intervertebral foramina. Each lateral zone, which passes into and just beyond the intervertebral foramen, can be further subdivided into subarticular (lateral recess), foraminal and extraforaminal regions (Macnab & McCulloch 1990). The lateral zone thus described forms the canal of the spinal nerve (the radicular or ‘root’ canal). The central zone of the canal is a little narrower than the radiological interpedicular distance if the lateral recess is considered to be part of the radicular canal rather than part of the central zone.
Intervertebral foramina
Intervertebral foramina (see also p. 712) are the principal routes of entry and exit to and from the vertebral canal, and are closely related to the main intervertebral articulations. (Minor routes occur between the median, often partly fused, margins of the ligamenta flava.) The same general arrangement applies throughout the vertebral column, between the axis and sacrum, although there are some quantitative and structural regional variations. Because of their construction, contents and susceptibilities to multiple disorders, the intervertebral foramina are loci of great biomechanical, functional and clinical significance. The specializations cranial to the axis and at sacral levels are described with the individual bones and articulations.
The boundaries of a generalized intervertebral foramen (Fig. 42.17) are anteriorly, from above downwards, the posterolateral aspect of the superior vertebral body, the posterolateral aspect of the intervertebral symphysis (including the disc), and a small (variable) posterolateral part of the body of the inferior vertebra; superiorly, the compact bone of the deep arched inferior vertebral notch of the vertebra above; inferiorly, the compact bone of the shallow superior vertebral notch of the vertebra below; and posteriorly a part of the ventral aspect of the fibrous capsule of the facet synovial joint. Cervical intervertebral foramina are distinct in having superior and inferior vertebral notches of almost equal depth which, in accord with the direction of the pedicles, face anterolaterally. External to them, and oriented in the same direction, is a transverse process. The thoracic and lumbar intervertebral foramina face laterally and their transverse processes are posterior. In addition, the anteroinferior boundaries of the first to tenth thoracic foramina are formed by the articulations of the head of a rib and the capsules of double synovial joints (with the demifacets on adjacent vertebrae and the intra-articular ligament between the costocapitular ridge and the intervertebral symphysis). Lumbar foramina lie between the two principal lines of vertebral attachment of psoas major. The walls of each foramen are covered throughout by fibrous tissue which is in turn periosteal (though the presence of a true periosteum lining the vertebral canal is controversial [Newell 1999]), perichondrial, anular and capsular. The more lateral parts of the foramina may be crossed at a variable level by narrow fibrous bands, the transforaminal ligaments (for detail of these ligaments see Bogduk 2005). The true foramen is the foraminal region of the canal of the spinal nerve (the radicular or ‘root’ canal). A foramen contains a segmental mixed spinal nerve and its sheaths, from two to four recurrent meningeal (sinuvertebral) nerves, variable numbers of spinal arteries, and plexiform venous connections between the internal and external vertebral venous plexuses. These structures, particularly the nerves, may be affected by trauma or one of the many disorders which may affect tissues bordering the foramen. In particular, nerve compression and irritation may be caused by intervertebral disc prolapse, or by bony entrapment as the size of the foramen decreases. This decrease may result from facet joint osteoarthritis, osteophyte formation, disc degeneration and degenerative spondylolisthesis, all of which may lead to lateral or foraminal spinal stenosis.
CERVICAL VERTEBRAE
The cervical vertebrae (Fig. 42.18, Fig. 42.19) are the smallest of the moveable vertebrae, and are characterized by a foramen in each transverse process. The first, second and seventh have special features and will be considered separately. The third, fourth and fifth cervical are almost identical, and the sixth, while typical in its general features, has minor distinguishing differences.
Typical cervical vertebra
A typical cervical vertebra (Fig. 42.20, Fig. 42.21) has a small, relatively broad vertebral body. The pedicles project posterolaterally and the longer laminae posteromedially, enclosing a large, roughly triangular vertebral foramen; the vertebral canal here accommodates the cervical enlargement of the spinal cord. The pedicles attach midway between the discal surfaces of the vertebral body, so the superior and inferior vertebral notches are of similar depth. The laminae are thin and slightly curved, with a thin superior and slightly thicker inferior border. The spinous process (‘spine’) is short and bifid, with two tubercles which are often unequal in size. The junction between lamina and pedicle bulges laterally between the superior and inferior articular processes to form an articular pillar (‘lateral mass’) on each side. The transverse process is morphologically composite around the foramen transversarium. Its dorsal and ventral bars terminate laterally as corresponding tubercles. The tubercles are connected, lateral to the foramen, by the costal (or intertubercular) lamella: these three elements represent morphologically the capitellum, tubercle and neck of a cervical costal element (p. 770, Fig. 44.11). The attachment of the dorsal bar to the pediculolaminar junction represents the morphological transverse process and the attachment of the ventral bar to the ventral body represents the capitellar process. In all but the seventh cervical vertebra, the foramen transversarium normally transmits the vertebral artery and vein and a branch from the cervicothoracic ganglion (vertebral nerve).
The vertebral body has a convex anterior surface. The discal margin gives attachment to the anterior longitudinal ligament. The posterior surface is flat or minimally concave, and its discal margins give attachment to the posterior longitudinal ligament. The central area displays several vascular foramina, of which two are commonly relatively larger. These are the basivertebral foramina which transmit basivertebral veins to the anterior internal vertebral veins. The superior discal surface is saddle-shaped, formed by flange-like lips which arise from most of the lateral circumference of the upper margin of the vertebral body; these are sometimes referred to as uncinate or neurocentral lips or processes. The inferior discal surface is also concave: the concavity is produced mainly by a broad projection from the anterior margin which partly overlaps the anterior surface of the intervertebral disc. The discal surfaces of cervical vertebrae are so shaped in order to restrict both lateral and anteroposterior gliding movements during articulation. The paired ligamenta flava extend from the superior border of each lamina below to the roughened inferior half of the anterior surfaces of the lamina above. The superior part of the anterior surface of each lamina is smooth, like the immediately adjacent surfaces of the pedicles, which are usually in direct contact with the dura mater and cervical root sheaths to which they may become loosely attached. The spinous process of the sixth cervical vertebra is larger, and is often not bifid.
C1, atlas
The atlas, the first cervical vertebra (Fig. 42.22), supports the head. It is unique in that it fails to incorporate a centrum, whose expected position is occupied by the dens, a cranial protuberance from the axis. The atlas consists of two lateral masses connected by a short anterior and a longer posterior arch. The transverse ligament retains the dens against the anterior arch.
The lateral masses are ovoid, their long axes converging anteriorly. Each bears a kidney-shaped superior articular facet for the respective occipital condyle, which is sometimes completely divided into a larger anterior and a smaller posterior part (Lang 1986). The inferior articular facet of the lateral mass is almost circular and is flat or slightly concave. It is orientated more obliquely to the transverse plane than the superior facet, and faces more medially and very slightly backwards. On the medial surface of each lateral mass is a roughened area which bears vascular foramina and a tubercle for attachment of the transverse ligament. In adults the distance between these tubercles is shorter than the transverse ligament itself, with a mean value of approximately 16 mm.
The transverse processes are longer than those of all cervical vertebrae except the seventh (Fig. 42.18). They act as strong levers for the muscles which make fine adjustments to keep the head balanced. Maximum atlantal width varies from 74–95 mm in males and 65–76 mm in females, and this affords a useful criterion for assessing sex in human remains. The apex of the transverse process, which is usually broad, flat and palpable between the mastoid process and ramus of the mandible, is homologous with the posterior tubercle of typical cervical vertebrae: the remaining part of the transverse process consists of the costal lamella. A small anterior tubercle is sometimes visible on the anterior aspect of the lateral mass. The costal lamella is sometimes deficient, which leaves the foramen transversarium open anteriorly.
The atlas is commonly ossified from three centres (Fig. 42.23). One appears in each lateral mass at about the seventh week, gradually extending into the posterior arch where they unite between the third and fourth years, usually directly but occasionally through a separate centre. At birth, the anterior arch is fibrocartilaginous, and a separate centre appears about the end of the first year. This unites with the lateral masses between the sixth and eighth year, the lines of union extending across anterior parts of the superior articular facets. Occasionally the anterior arch is formed by the extension and ultimate union of centres in the lateral masses and sometimes from two lateral centres in the arch itself.
C2, axis
The axis, the second cervical vertebra (Fig. 42.24, Fig. 42.25), acts as an axle for rotation of the atlas and head around the strong dens (odontoid process), which projects cranially from the superior surface of the body.
The pedicles are stout, and the superior surface carries part of the superior articular facet, which also projects laterally and downwards onto the transverse process. The anterolateral surface is deeply grooved by the vertebral artery, running beneath the thin lateral part of the inferior surface of the superior articular facet, which can become quite thin. The inferior surface of each pedicle bears a deep, smooth inferior intervertebral notch, in which the large root sheath of the third cervical nerve lies. The interarticular part of the pedicle is short and lies between the relatively small inferior posterior articular process (which is located at the pediculolaminar junction and bearing a small anteriorly facing facet) and the superior articular surface.
The laminae are thick, and give attachment to the ligamenta flava.
The axis is ossified from five primary and two secondary centres (Fig. 42.23). The vertebral arch has two primary centres and the centrum one, as in a typical vertebra. The former appear about the seventh or eighth week, and that for the centrum about the fourth or fifth month. The dens is largely ossified from bilateral centres, appearing about the sixth month and joining before birth to form a conical mass, deeply cleft above by cartilage. This cuneiform cartilage forms the apex of the odontoid process. A centre appears in it which shows considerable individual variation in both time of appearance and time of fusion to the rest of the dens: it most often appears between five and eight years, but sometimes even later, fusing with the main mass about the 12th year. The cartilage was thought to be part of the cranial sclerotomal half of the first cervical segment or pro-atlas. It has also been suggested that the apical centre for the dens is itself derived from the pro-atlas, which may also contribute to lateral atlantal masses. The dens is separated from the body by a cartilaginous disc, the circumference of which ossifies while its centre remains cartilaginous until old age; possible rudiments of adjacent epiphyses of atlas and axis may occur in the disc. A thin epiphysial plate is formed inferior to the body around puberty.
C7, seventh cervical vertebra
The seventh cervical vertebra, the vertebra prominens (Fig. 42.26), has a long spinous process which is visible at the lower end of the nuchal furrow. It ends in a prominent tubercle for the attachment of the ligamentum nuchae, and the muscles detailed below. The thick and prominent transverse processes lie behind and lateral to the foramina transversaria. The latter transmit vertebral veins, but not the vertebral artery, and each is often divided by a bony spicule. The costal lamella is relatively thin and may be partly deficient. It is grooved superiorly for the anterior ramus of the seventh cervical nerve, and usually carries a small and inconspicuous anterior tubercle. The posterior tubercle is prominent. The suprapleural membrane is attached to the anterior border of the transverse process.
THORACIC VERTEBRAE
Thoracic vertebrae in general and changes with descending level
All thoracic vertebral bodies display lateral costal facets and all but the lowest two or three transverse processes also have facets (Fig. 42.27). The facets articulate with the head of the rib (costocapitular facet) and its tubercle (costotubercular facet) respectively. The first and ninth to 12th vertebrae also have atypical features, but the remainder are very similar, except for relatively minor details.
The body is typically a waisted cylinder (Fig. 42.28) except where the vertebral foramen encroaches, and transverse and anteroposterior dimensions are almost equal. On each side there are two costal facets (which are really demifacets): the superior and usually larger pair at the upper border are anterior to the pedicles, while the inferior pair at the lower border are anterior to the vertebral notches. The vertebral foramen (Fig. 42.14) is small and circular, so the pedicles do not diverge as they do in cervical vertebrae: the thoracic spinal cord is smaller and more circular than the cervical cord. The laminae are short, thick and broad, and overlap from above downwards. The spinous process slants downward. The thin and almost flat superior articular processes project from the pediculolaminar junctions and face posteriorly and a little superolaterally. The inferior processes project down from the laminae and their facets are directed forwards and a little superomedially. The large, club-like transverse processes also project from the pediculolaminar junctions. Each passes posterolaterally and bears, near its tip, anterior oval facets for articulation with the tubercle of the corresponding rib.
T1
The first thoracic vertebra (Fig. 42.27) resembles a cervical vertebra in its body, both in shape and in the distinctive posterolateral ‘lipping’ which forms the anterior border of the superior vertebral notch. There are circular superior costal facets for articulation with the whole facet on the head of the first rib. The smaller, semilunar inferior facets articulate with a demifacet on the head of the second rib. The upper costal facet is often incomplete, in which case the first rib articulates with the seventh cervical vertebra and the intervening disc. A small, deep depression often occurs below the facet. The long, thick spine is horizontal and commonly as prominent as that of the seventh cervical vertebra.
T9
The ninth thoracic vertebra (Fig. 42.27) is otherwise typical, but it often fails to articulate with the tenth ribs, in which case the inferior demifacets are absent.
T10
The 10th thoracic vertebra (Fig. 42.27) only articulates with the 10th pair of ribs, so that superior facets only appear on the body. These are usually large and semilunar, but are oval when the tenth ribs fail to articulate with the ninth vertebra and intervening disc. The transverse process may or may not bear a facet for the tubercle of the 10th rib.
T11
The 11th thoracic vertebra (Fig. 42.27) articulates only with the heads of the 11th ribs. The circular costal facets are close to the upper border of the body and extend onto the pedicles. The small transverse processes lack articular facets. The 11th and 12th thoracic spinous processes are triangular, with blunt apices, a horizontal lower and an oblique upper border.
T12
The 12th thoracic vertebra (Fig. 42.27) articulates with the heads of the 12th ribs by circular facets somewhat below the upper border, spreading on to the pedicles. The body is large and the vertebra has some lumbar features. The transverse process is replaced by three small tubercles: the superior is largest, projects upwards and corresponds to a lumbar mammillary process, though it does not lie as close to the superior articular process; the lateral tubercle is the homologue of a transverse process; the inferior is the homologue of a lumbar accessory process. The superior and inferior processes are surprisingly long in some specimens.
LUMBAR VERTEBRAE
Lumbar vertebrae in general
The five lumbar vertebrae are distinguished by their large size and absence of costal facets and transverse foramina. The body is wider transversely (Fig. 42.29, see Fig. 42.33B). The vertebral foramen is triangular, larger than at thoracic levels but smaller than at cervical levels. The pedicles are short. The spinous process is almost horizontal, quadrangular and thickened along its posterior and inferior borders. The superior articular processes bear vertical concave articular facets facing posteromedially, with a rough mammillary process on their posterior borders (Fig. 42.30A). The inferior articular processes have vertical convex articular facets which face anterolaterally. The transverse processes are thin and long, except on the more substantial fifth pair. A small accessory process marks the posteroinferior aspect of the root of each transverse process. The accessory and mammillary processes are linked by a fine ligament, the mammillo-accessory ligament, which is sometimes ossified, and beneath which runs the medial branch of the dorsal primary ramus of the spinal nerve (Bogduk 2005).
Strong paired pedicles arise posterolaterally from each body near its upper border. Superior vertebral notches are shallow and the inferior ones are deep. The laminae are broad and short, but do not overlap as much as those of the thoracic vertebrae. The fifth spine is the smallest, and its apex is often rounded and down-turned. Upper lumbar superior articular processes are further apart than inferior ones, but the difference is slight in the fourth and negligible in the fifth. The articular facets are reciprocally concave (superior) and convex (inferior), which allows flexion, extension, lateral bending and some degree of rotation. There are sex differences in the angle of inclination and depth of curvature of the articular facets. The facets are sometimes asymmetrical. Transverse processes, except the fifth, are anteroposteriorly compressed and project posterolaterally. The lower border of the fifth transverse process is angulated, passes laterally and then superolaterally to a blunt tip, and the whole process presents a greater upward inclination than the fourth. The angle on the inferior border may represent the tip of the costal element and the lateral end the tip of the true transverse process. The lumbar transverse processes increase in length from first to third and then shorten (Fig. 42.31). The fifth pair incline both upwards and posterolaterally. The costal element is incorporated in the mature transverse process.
The first lumbar vertebral foramen contains the conus medullaris of the spinal cord, while lower foramina contain the cauda equina and spinal meninges (Fig. 42.11B, Fig. 42.32A). Variation occurs in the sagittal and coronal dimensions of the lumbar vertebral canal, both within and between normal populations.
Muscle and fascial attachments (see also p. 708)
Upper and lower borders of lumbar bodies give attachment to the anterior and posterior longitudinal ligaments. The upper bodies (three on the right, two on the left) give attachments to the crura of the diaphragm lateral to the anterior longitudinal ligament. Posterolaterally, psoas major is attached to the upper and lower margins of all the lumbar bodies, and between them, tendinous arches carry its attachments across their concave sides (see Fig. 62.5). The posterior lamella of the thoracolumbar fascia, erectores spinae, spinales thoracis, multifidi, interspinal muscles and ligaments, and supraspinous ligaments are all attached to spinous processes. All lumbar transverse processes present a vertical ridge on the anterior surface, nearer the tip, which marks the attachment of the anterior layer of the thoracolumbar fascia, and separates the surface into medial and lateral areas for psoas major and quadratus lumborum respectively. The middle layer of the fascia is attached to the apices of the transverse processes; the medial and lateral arcuate ligaments attach to the vertical ridge on the anterior aspect of the first pair, and the iliolumbar ligament attaches to the apices of the fifth pair. Posteriorly the transverse processes are covered by deep dorsal muscles, and fibres of longissimus thoracis are attached to them and to their accessory processes. The ventral lateral intertransverse muscles are attached to their upper and lower borders, while the dorsal attach cranially to the accessory process and caudally to the upper border of the transverse process. The mammillary process, homologous with the superior tubercle of the 12th thoracic vertebra, gives attachment to multifidus and the medial intertransverse muscle. The latter also attaches to the accessory process, which is sometimes difficult to identify.
SACRUM
The base (Fig. 42.34) is the upper surface of the first sacral vertebra, the least modified from the typical vertebral plan. The body is large and wider transversely, and its anterior projecting edge is the sacral promontory. The vertebral foramen is triangular, its pedicles are short and diverge posterolaterally. The laminae are oblique, inclining down posteromedially to meet at a spinous tubercle. The superior articular processes project cranially, with concave articular facets directed posteromedially to articulate with the inferior articular processes of the fifth lumbar vertebra. The posterior part of each process projects backwards and its lateral aspect bears a rough area homologous with a lumbar mammillary process.
The anteroinferior pelvic surface (Fig. 42.35) is vertically and transversely concave, but the second sacral body may produce a convexity. Four pairs of pelvic sacral foramina communicate with the sacral canal through intervertebral foramina, and transmit ventral rami of the upper four sacral spinal nerves. The large area between the right and left foramina, which is formed by the flat pelvic aspects of the sacral bodies, bears evidence of their fusion at four transverse ridges. The longitudinal bars between the foramina are costal elements, which fuse to the vertebrae. Lateral to the foramina the costal elements unite. Posteriorly they unite with the transverse processes to form the lateral part of the sacrum, which expands basally as the ala.
The posterosuperior aspect of the dorsal surface bears a raised, interrupted, median sacral crest with four (sometimes three) spinous tubercles which represent fused sacral spines (Fig. 42.36). Below the fourth (or third) tubercle there is an arched sacral hiatus in the posterior wall of the sacral canal. This hiatus is produced by the failure of the laminae of the fifth sacral vertebra to meet in the median plane, and as a result the posterior surface of the body of that vertebra is exposed on the dorsal surface of the sacrum. Flanking the median crest, the posterior surface is formed by fused laminae, and lateral to this are four pairs of dorsal sacral foramina. Like the pelvic foramina, they lead into the sacral canal through intervertebral foramina, and each transmits the dorsal ramus of a sacral spinal nerve. Medial to the foramina, and vertically below each articular process of the first sacral vertebra, is a row of four small tubercles, which collectively constitute the intermediate sacral crest. These are sometimes termed articular tubercles, and represent fused contiguous articular processes. The inferior articular processes of the fifth sacral vertebra are free and project downwards at the sides of the sacral hiatus as sacral cornua, connected to coccygeal cornua by intercornual ligaments. The interrupted roughened crest to the lateral side of the dorsal sacral foramina is the lateral sacral crest which is formed by fused transverse processes, whose apices appear as a row of transverse tubercles.
The upper three sacral spinal dorsal rami pierce multifidus as they emerge via dorsal foramina.
The lateral surface (Fig. 42.37A) is a fusion of transverse processes and costal elements. It is wide above, and rapidly narrows in its lower part. The broad upper part bears an auricular surface for articulation with the ilium, and the area posterior to this is rough and deeply pitted by the attachment of ligaments. The auricular surface, borne by costal elements, is like an inverted letter L. The shorter, cranial limb is restricted to the first sacral vertebra; the caudal limb descends to the middle of the third. Beyond this the lateral surface is non-articular and reduced in breadth. Caudally it curves medially to the body of the fifth sacral vertebra at the inferior lateral angle, beyond which the surface becomes a thin lateral border. A variable accessory sacral articular facet sometimes occurs, posterior to the auricular surface.
The sacral canal (Fig. 42.37B) is formed by sacral vertebral foramina, and is triangular in section (Fig. 42.34). Its upper opening, seen on the basal surface, appears to be set obliquely. The inclination of the sacrum means that it is directed cranially in the standing position. Each lateral wall presents four intervertebral foramina, through which the canal is continuous with pelvic and dorsal sacral foramina. Its caudal opening is the sacral hiatus. The canal contains the cauda equina and the filum terminale, and the spinal meninges. Opposite the middle of the sacrum, the subarachnoid and subdural spaces close: the lower sacral spinal roots and filum terminale pierce the arachnoid and dura mater at that level. The filum terminale with its meningeal coverings emerges below the sacral hiatus and passes downwards across the dorsal surface of the fifth sacral vertebra and sacrococcygeal joint to reach the coccyx. The fifth sacral spinal nerves also emerge through the hiatus medial to the sacral cornua, and groove the lateral aspects of the fifth sacral vertebra.
The pelvic surface gives attachment to piriformis in its second to fourth segments, to iliacus superolaterally, and to coccygeus inferolaterally. The dorsal surface gives attachment to the aponeurosis of erector spinae along a U-shaped area of spinous and transverse tubercles, covering multifidus which occupies the enclosed area (Fig. 42.34). On the lateral border below the auricular surface, gluteus maximus is attached dorsal and coccygeus is attached ventral to the sacrotuberous and sacrospinous ligaments.
The sacrum resembles typical vertebrae in the ossification of its segments (Fig. 42.38). Primary centres for the centrum and each half vertebral arch appear between the 10th and 20th weeks. Primary centres for the costal elements of the upper three or more segments appear superolateral to the pelvic sacral foramina, between the sixth and eighth prenatal months. Each costal element unites with its half vertebral arch between the second and fifth years, and the conjoined element so formed unites anteriorly with the centrum and posteriorly with its opposite fellow at about the eighth year. Thereafter the upper and lower surfaces of each sacral body are covered by an epiphysial plate of hyaline cartilage which is separated from its neighbour by the fibrocartilaginous precursor of an intervertebral disc. Laterally, successive conjoined vertebral arches and costal elements are separated by hyaline cartilage; a cartilaginous epiphysis, sometimes divided into upper and lower parts, develops on each auricular and adjacent lateral surface. Soon after puberty the fused vertebral arches and costal elements of adjacent vertebrae begin to coalesce from below upwards. At the same time individual epiphysial centres develop for the upper and lower surfaces of bodies, spinous tubercles, transverse tubercles and costal elements.
COCCYX
The coccyx (Fig. 42.39) is a small triangular bone and is often asymmetrical in shape. It usually consists of four fused rudimentary vertebrae, although the number varies from three to five, and the first is sometimes separate. The bone is directed downwards and ventrally from the sacral apex: its pelvic surface is tilted upwards and forwards, its dorsum downwards and backwards. Orientation varies with mobility and between individuals.
Muscle and ligament attachments
The lateral parts of the pelvic surface, including the rudimentary transverse processes, give attachment to the levatores ani and coccygei. The anterior sacrococcygeal ligament is attached to the front of the first and sometimes second coccygeal vertebral bodies (Fig. 80.8). The cornua give attachment to the intercornual ligaments. The lateral sacrococcygeal ligament connects the transverse process to the inferolateral sacral angle. Gluteus maximus is attached to the dorsal surface, and both levator ani and sphincter ani externus are attached to the tip of the bone. The median area gives attachment to the deep and superficial posterior sacrococcygeal ligaments, the superficial descending from the margins of the sacral hiatus and sometimes closing the sacral canal. The filum terminale, which is situated between the two ligaments, blends with them on the dorsum of the first coccygeal vertebra.
LIGAMENTS OF THE VERTEBRAL COLUMN
ANTERIOR LONGITUDINAL LIGAMENT
The anterior longitudinal ligament (Fig. 42.40B) is a strong band extending along the anterior surfaces of the vertebral bodies. It is broader caudally, thicker and narrower in thoracic than in cervical and lumbar regions, and is also relatively thicker and narrower opposite vertebral bodies than at the levels of intervertebral symphyses. It extends from the basilar part of the occipital bone to the anterior tubercle of C1 and the front of the body of C2, then continues caudally to the front of the upper sacrum. Its longitudinal fibres are strongly adherent to the intervertebral discs, hyaline cartilage end-plates and margins of adjacent vertebral bodies, and are loosely attached at intermediate levels of the bodies, where the ligament fills their anterior concavities, flattening the vertebral profile. At these various levels ligamentous fibres blend with the subjacent periosteum, perichondrium and periphery of the anulus fibrosus. The anterior longitudinal ligament has several layers. The most superficial fibres are the longest and extend over three or four vertebrae, the intermediate extend between two or three, and the deepest from one body to the next. Laterally, short fibres connect adjacent vertebrae.
POSTERIOR LONGITUDINAL LIGAMENT
The posterior longitudinal ligament (Fig. 42.41) lies on the posterior surfaces of the vertebral bodies in the vertebral canal, attached between the body of C2 and the sacrum, and continuous with the membrana tectoria above. Its smooth glistening fibres, attached to intervertebral discs, hyaline cartilage end-plates and adjacent margins of vertebral bodies, are separated between attachments by basivertebral veins and the venous channels which drain them into anterior internal vertebral plexuses. At cervical and upper thoracic levels the ligament is broad and of uniform width, but in lower thoracic and lumbar regions it is denticulated, narrow over vertebral bodies and broad over discs. Its superficial fibres bridge three or four vertebrae, while deeper fibres extend between adjacent vertebrae as perivertebral ligaments, which are close to and, in adults, fused with, the anulus fibrosus of the intervertebral disc. The layers of the posterior longitudinal ligament and the relationship of the ligament to associated membranes in the epidural space are fully discussed by Loughenbury et al (2006).
LIGAMENTA FLAVA
The ligamenta flava (Fig. 42.40B, Fig. 42.42) connect laminae of adjacent vertebrae in the vertebral canal. Their attachments extend from facet joint capsules to the point where laminae fuse to form spines. Here their posterior margins meet and are partially united; the intervals between them admit veins which connect the internal and posterior external vertebral venous plexuses. Their predominant tissue is yellow elastic tissue, whose almost perpendicular fibres descend from the lower anterior surface of one lamina to the posterior surface and upper margin of the lamina below. The anterior surface of the ligaments is covered by a fine, continuous smooth lining membrane (Newell 1999). The ligaments are thin, broad and long in the cervical region, thicker in the thoracic and thickest at lumbar levels. They arrest separation of the laminae in spinal flexion, preventing abrupt limitation, and also assist restoration to an erect posture after flexion, perhaps protecting discs from injury.
INTERSPINOUS LIGAMENTS
Interspinous ligaments (Fig. 42.40B) connect the facing edges of consecutive spinous processes, and extend ventrally as far as the ligamentum flavum and dorsally to the supraspinous ligament, when this ligament is present (see below). They differ structurally in thoracic, lumbar and cervical levels. The thoracic interspinous ligaments are narrow and elongated, whereas those at lumbar levels are thick and quadrilateral, and occur as closely applied pairs, the left and right ligaments being separated by a narrow or potential cleft. In the lumbar ligaments, collagen fibres run obliquely inferiorly and ventrally and only the deepest fibres are truly ligamentous. The more dorsal fibres are derived from tendons of longissimus thoracis that dip into the interspinous space to gain attachment to the superior edge of the spinous process rather than to its tip. Distinctive interspinous ligaments are not evident at cervical levels, where they are represented by the median septum of the ligamentum nuchae as it passes between the cervical spinous processes.
SUPRASPINOUS LIGAMENT
The supraspinous ligament (Fig. 42.40B) is a strong fibrous cord which connects the tips of spinous process from C7 to L3 or L4. It is regularly deficient. The most superficial fibres extend over three or four vertebrae, the deeper span two or three, and the deepest connect adjacent spines and are continuous with the interspinous ligament. Most of the ligament is formed by the tendons of muscles with posterior midline attachments, i.e. semispinalis, longissimus, trapezius and latissimus dorsi. Only the most superficial fibres lack any connection with muscle. Below L4 the ligament is replaced by the decussating fibres of latissimus dorsi.
INTERTRANSVERSE LIGAMENTS
Intertransverse ligaments run between adjacent transverse processes. At cervical levels they consist of a few, irregular fibres which are largely replaced by intertransverse muscles; in the thoracic region they are cords intimately blended with adjacent muscles; in the lumbar region they are thin and membranous. For the detailed anatomy of the lumbar intertransverse ligaments, see Bogduk (2005).
JOINTS
INTERVERTEBRAL JOINTS
Joints between the vertebral bodies
Articulating surfaces: intervertebral discs
The intervertebral discs are the chief bonds between the adjacent surfaces of vertebral bodies from C2 to the sacrum. Except at the sites of the uncovertebral (neurocentral) joints of Luschka, disc outlines correspond with the adjacent bodies. Their thickness varies in different regions and within individual discs. Each disc consists of an outer lamellated anulus fibrosus and an inner nucleus pulposus. (Fig. 42.40A1).
In cervical and lumbar regions the discs are thicker anteriorly, contributing to the anterior convexity of the vertebral column. In the thoracic region they are nearly uniform, and the anterior concavity is largely due to the vertebral bodies. Discs are thinnest in the upper thoracic region and thickest in the lumbar region. They adhere to thin layers of cartilage on the superior and inferior vertebral surfaces, the vertebral end-plates. The latter do not reach the periphery of the vertebral bodies but are encircled by ring apophyses. The end-plates contain both hyaline cartilage and fibrocartilage. The fibrocartilaginous component lies nearer to the disc, and is sometimes considered not to be part of the end-plate itself. The fibrocartilaginous components of the end-plates above and below the nucleus pulposus, together with the innermost lamellae of the anulus fibrosus, form a flattened sphere of collagen which surrounds and encloses the nucleus (Fig. 42.43). The overall proportion of fibrocartilage in the end-plate increases with age. While all discs are attached to the anterior and posterior longitudinal ligaments, discs in the thoracic region are additionally tied laterally, by intra-articular ligaments, to the heads of ribs articulating with adjacent vertebrae. Intervertebral discs form about a quarter of the length of the postaxial vertebral column: cervical and lumbar regions make a greater contribution than the thoracic and are thus more pliant.
The anulus fibrosus has a narrow outer collagenous zone and a wider inner fibrocartilaginous zone. Its lamellae, which are convex peripherally when seen in vertical section, are incomplete collars. The internal vertical concavity of the lamellae conforms to the surface profile of the nucleus pulposus. In all quadrants of the anulus, about half the lamellae are incomplete; the proportion increases in the posterolateral region. The exact nature of the interlamellar substance remains in some doubt. Posteriorly, lamellae join in a complex manner. Fibres in the rest of each lamella are parallel and run obliquely between vertebrae at about 65° to the vertical (Fig. 42.40A2). Fibres in successive lamellae cross each other obliquely in opposite directions, thus limiting rotation. The obliquity of fibres in deeper zones varies in different lamellae. Posterior fibres may sometimes be predominantly vertical, which possibly predisposes them to herniation.
This standard description of the anulus may not apply at all spinal levels: a recent cadaveric study indicates that the anulus is usually incomplete posteriorly in adult cervical discs (Mercer & Bogduk 1999) (Fig. 42.40A3).
The nucleus pulposus is better developed in cervical and lumbar regions and lies between the centre of the disc and its posterior surface. At birth it is large, soft, gelatinous and composed of mucoid material. It contains a few multinucleated notochordal cells and is invaded by cells and collagen fibres from the inner zone of the adjacent anulus fibrosus. Notochordal cells disappear in the first decade, and the mucoid material is gradually replaced by fibrocartilage, derived mainly from the anulus fibrosus and the plates of hyaline cartilage adjoining the vertebral bodies. The nucleus pulposus becomes less differentiated from the remainder of the disc as age progresses, and gradually becomes less hydrated and increasingly fibrous. The type II collagen of the nucleus becomes more like the type I of the anulus as its fibril diameter increases. The quantity of aggregated proteoglycans in the nucleus decreases, while the keratan sulphate/chondroitin sulphate ratio increases. As increased cross-linking occurs between collagen and the proteoglycans the discs lose their water-binding capacity, become stiffer and more liable to injury. Contrary to what was previously thought, it has now been shown that lumbar discs do not decrease in overall height as a part of normal ageing. The anulus gradually loses height as its radial bulge increases, but the nucleus retains height and may increase in convexity as it increasingly indents the end-plate. Loss of trunk height with age results from a decrease in vertebral body height (Bogduk 2005). When the disc is not loaded, pressure in the nucleus pulposus is low at all ages.
For a review of the structure and function of the human intervertebral disc see Adams et al (2006).
Small offshoots of spinal branches of arteries supplying the vertebral column (see p. 714) form an anastomosis on the outer surface of the anulus fibrosus and supply its most peripheral fibres. Normal discs are otherwise avascular and are dependent for their nutrition on diffusion from vertebral bone beneath adjacent end-plates and from the peripheral anulus. Vascular and avascular parts differ in their reaction to injury. Venous drainage is via the external and internal vertebral venous plexuses to the intervertebral veins and thence to the larger named veins which drain the vertebral column. Lymphatic drainage of the vertebral column is briefly considered above (see p. 715). Nothing specific is known about the lymphatic drainage of the disc.
The nerve supply of intervertebral discs has been studied in detail in the lumbar region (Fig. 42.44). The outer third of the anulus is innervated by the sinuvertebral nerves (see p. 754): each sinuvertebral nerve supplies both the disc at the level of its spinal nerve of origin and the disc one level above. The anterior part of the anulus is supplied by the sympathetic (grey ramus communicans) rather than by the mixed nerve. In damaged and degenerate discs the nerves may penetrate more centrally into the disc substance. The sinuvertebral nerves are condensations within extensive nerve plexuses which lie on the posterior longitudinal ligament. Similar plexuses have been demonstrated anteriorly, covering the anterior longitudinal ligament, and laterally in the fetus.
A prolapsed intervertebral disc most commonly affects the 20–55 year age group, and is most often seen at the L4/5 and lumbosacral levels. It may also affect the cervical discs, particularly at C5/6 and C6/7. The thoracic discs are rarely affected. Acute tearing or chronic degeneration of the posterior lamellae of the anulus fibrosus allows deformation and herniation of the disc contents. The disc most often prolapses just lateral to the posterior longitudinal ligament and can compress one or two spinal nerves unilaterally (Fig. 42.45). Much less commonly, the prolapse is central, in the midline posteriorly. The compression of neural structures may then be bilateral, affecting the cord itself or the whole cauda equina. If the damaged anulus ruptures completely, some of the nuclear tissue may escape into the vertebral and ‘root’ canals. This sequestrated material may migrate within the canals and cause nerve compression at spinal levels distant from that of the disc rupture. The disc material itself may have an irritative effect on the spinal nerve.
Regarding the anatomy of the vertebral canal and intervertebral foramen in relation to disc prolapse, it is important to understand that one or both of two spinal nerves and their roots may be affected by a single prolapse, depending upon the exact site of the prolapse in the horizontal plane. At the level of each disc and foramen, there are two spinal nerves (and their roots) to consider: these are the exiting nerve and the traversing nerve (Macnab & McCulloch 1990) (Fig. 42.46). The nerve usually affected at lumbar levels is the traversing nerve, which crosses the back of the disc on its way to become the exiting nerve at the level below. Thus a lumbosacral (i.e. L5/S1) disc prolapse usually compresses the S1 nerve. However, a prolapse may affect the exiting nerve at its own level. This is especially likely if the prolapse is in the extraforaminal zone of the ‘root’ canal, the so-called ‘far lateral’ prolapse. At cervical levels, because the roots and nerve leave the vertebral canal almost horizontally, the prolapse usually affects the exiting nerve. This nerve will still bear the number of the vertebra below the affected disc, because cervical nerves exit the canal above the pedicle of their numerically corresponding vertebra. Neurological presentation will include signs and symptoms of spinal nerve damage at the affected level. Thus pain and sensory loss will be dermatomal in distribution. Sensory changes usually precede motor loss.
For more detail on disc pathology and its consequences, see Adams et al (2006).
Facet (zygapophysial) joints
Detailed description of the anatomy of these joints is to be found in Bogduk (2005), where Bogduk explains why the term ‘facet joint’ is both incorrect and essentially ambiguous, on the grounds that facets are not restricted to zygapophysial articular processes and occur, for example, in both costovertebral and costotransverse joints.
Ligaments which work in conjunction with, and modify the function of, the facet joints throughout the vertebral column are described on page 728.
The posterior spinal branches of the arteries that supply the vertebral column form arterial anastomoses around the facet joints. Venous drainage is via the external and internal posterior vertebral venous plexuses to the intervertebral veins and thence to the larger named veins that drain the vertebral column. Lymphatic drainage follows the principles described for the vertebral column (p. 715).
CRANIOVERTEBRAL JOINTS
Atlanto-occipital joints
The atlas articulates with the occipital bone of the skull by a pair of synovial joints. The bones are connected by articular capsules and by the anterior and posterior atlanto-occipital membranes (Fig. 42.47, Fig. 42.48).
The ligaments are the anterior and posterior atlanto-occipital membranes.
Anterior atlanto-ocipital membrane
The anterior atlanto-occipital membrane (Fig. 42.47, Fig. 42.49) is a broad, dense fibrous structure which connects the anterior margin of the foramen magnum to the upper border of the anterior arch of the atlas. Laterally, it blends with the joint capsule, and medially it is strengthened by a median cord, which is the anterior longitudinal ligament stretching between the basilar occipital bone and anterior atlantal tubercle.
Posterior atlanto-occipital membrane
The posterior atlanto-occipital membrane (Fig. 42.48, Fig. 42.49) is broad, but relatively thin, and connects the posterior margin of the foramen magnum to the upper border of the posterior atlantal arch, blending laterally with the joint capsules. It arches over the grooves for the vertebral arteries, venous plexuses and first cervical nerve, and forms the ‘floor’ of the suboccipital triangle. The ligamentous border of this arch is sometimes ossified. The membrane is usually attached by a soft-tissue ‘bridge’, which may contain muscle or tendon fibres, to rectus capitis posterior minor, and is firmly attached anteriorly to the spinal dura in the same area (Zumpano et al 2006). The ligaments connecting the axis and the occipital bone are functionally involved with the posterior atlanto-occipital membrane.
Atlanto-axial joints
Anteriorly, the vertebral bodies are connected by the anterior longitudinal ligament (Fig. 42.47, Fig. 42.49): here a strong, thickened band attaches above to the lower border of the anterior tubercle of the anterior arch of the atlas and below to the front of the axial body. Posteriorly the vertebral arches are joined by the ligamenta flava (Fig. 42.48, Fig. 42.49) which are attached to the lower border of the atlantal arch above, and to the upper borders of the axial laminae. At this level these ligaments form a thin membrane, pierced laterally by the second cervical nerves.
Transverse atlantal and cruciform ligaments
The transverse atlantal ligament (Fig. 42.50) is a broad, strong band which arches across the atlantal ring behind the dens: its length varies about a mean of 20 mm. It is attached laterally to a small but prominent tubercle on the medial side of each atlantal lateral mass, and broadens medially where it is covered anteriorly by a thin layer of articular cartilage. It consists almost entirely of collagen fibres, which, in the central part of the ligament, cross one another at an angle to form an interlacing mesh. From its upper margin a strong median longitudinal band arises which inserts into the basilar part of the occipital bone between the apical ligament of the dens and membrana tectoria, and from its inferior surface a weaker and less consistent longitudinal band passes to the posterior surface of the axis. These transverse and longitudinal components together constitute the cruciform ligament. The transverse ligament divides the ring of the atlas into unequal parts (Fig. 42.22). The posterior two-thirds surrounds the spinal cord and meninges, the anterior third contains the dens, which the transverse ligament retains in position even when all other ligaments are divided.
LUMBOSACRAL JUNCTION
The major ligament associated with the lumbosacral junction is the iliolumbar ligament (Fig. 80.8, Fig. 42.51).
The iliolumbar ligament is attached to the tip and anteroinferior aspect of the fifth lumbar transverse process, and sometimes has a weak attachment to the fourth transverse process. It radiates laterally and is attached to the pelvis by two main bands. A lower band passes from the inferior aspect of the fifth lumbar transverse process and the body of the fifth lumbar vertebra across the anterior sacroiliac ligament to reach the posterior margin of the iliac fossa. An upper band, part of the attachment of quadratus lumborum, passes to the iliac crest anterior to the sacroiliac joint, and is continuous above with the anterior layer of the thoracolumbar fascia. The lower ligament has a more vertical component that reaches the posterior iliopectineal line: this component is a lateral relation of the L5 ventral ramus. A posterior component of the iliolumbar ligament passes behind quadratus lumborum to attach to the ilium.
The lumbosacral junction is innervated by branches derived from the fourth and fifth lumbar spinal nerves: the pattern of distribution is as described on page 707.
SACROCOCCYGEAL JUNCTION
The anterior sacrococcygeal ligament consists of irregular fibres that descend on the pelvic surfaces of both sacrum and coccyx, and is attached in the same way as the anterior longitudinal ligament. The flat superior posterior sacrococcygeal ligament passes from the margin of the sacral hiatus to the dorsal coccygeal surface, roofing the lower sacral canal (Fig. 42.51). The deep posterior sacrococcygeal ligament passes from the back of the fifth sacral vertebral body to the dorsum of the coccyx and corresponds to the posterior longitudinal ligament. On each side, the lateral sacrococcygeal ligaments connect the coccygeal transverse processes to the inferolateral sacral angles, completing foramina for the fifth sacral spinal nerves. Similarly, the intercornual ligaments connect the sacral and coccygeal cornua, and a fasciculus connects the sacral cornua to the coccygeal transverse processes.
MUSCLES
The musculature of the back is arranged in a series of layers, of which only the deeper are true, intrinsic, back muscles (Fig. 42.52). The true back muscles are characterized by their position and by their innervation by branches of the posterior (dorsal) rami of the spinal nerves. The true back muscles below the neck lie deep to the posterior layer of the thoracolumbar fascia. In the lumbar region, where the layers of the thoracolumbar fascia are well-defined, they occupy the compartment between its posterior and middle layers.
Lying superficial to the true, intrinsic muscles are the extrinsic, ‘immigrant’ muscles (Fig. 42.52). The most superficial of these run between the upper limb and the axial skeleton, and consist of trapezius, latissimus dorsi, levator scapulae and the rhomboid muscles. Beneath this layer lie the serratus posterior group, superior and inferior, which are variably developed but usually thin muscles whose function may be respiratory or possibly proprioceptive. All the extrinsic muscles are innervated by ventral rami.
Trapezius, latissimus dorsi, levator scapulae, rhomboid major and rhomboid minor are described on pages 809–811; serratus posterior superior and inferior are described on pages 925–926. The muscles of the posterior abdominal wall are described on page 1070.
Muscle attachments and fibre direction
It has been shown (Bogduk 2005) that in the lumbar spine it is more appropriate morphologically and biomechanically to view the muscles in the reverse direction – from above downwards. It is reasonable to argue that this view may also be applied to the cervical and thoracic muscles, and so for uniformity in this section, all muscles have been described in this way.
Ligamentum nuchae
The ligamentum nuchae is not a ligament of the neck, for it does not connect adjacent bones and lacks the internal structure typical of a ligament. It is a unique arrangement of tendons and fascia between the posterior muscles of the neck. It consists of a dorsal raphe and a median septal portion. The dorsal raphe lies superficially along the posterior midline of the neck. It is attached to the external occipital protruberance superiorly, and the tip of the C7 spinous process inferiorly. In its superior half it consists of the aggregated tendons of the most medial fibres of the cervical trapezius. Because of their longitudinal arrangement, these tendons have been described as forming the funicular portion of the ligamentum nuchae (Mercer & Bogduk 2003). In its inferior half the funicular portion is joined by the obliquely orientated tendons of splenius capitis and rhomboid minor. Across the midline the tendons of splenius capitis are continuous with those of rhomboid minor on the other side, and they interweave with the tendons of the reciprocal sets of muscles to produce the raphenous structure of the ligamentum nuchae. From the ventral surface of the dorsal raphe, a median fascial septum extends deeply towards the vertebral column and separates semispinalis capitis from its opposite partner. A lateral expansion of the septum extends laterally ventral to semispinalis capitis, separating it from multifidus and semispinalis cervicis. The median continuation of the septum reaches the tips of the cervical spinous processes, and extends into the cervical interspinous spaces as far as the ligamentum flavum. At upper cervical levels the septum is attached to the external occipital crest and the posterior tubercle of the atlas, and it blends with the posterior atlanto-occipital and posterior atlanto-axial membranes. Passing through these membranes it is attached to the posterior surface of the dura mater (Dean & Mitchell 2002). Only the dorsal raphe affords attachment to muscles: no muscles arise from the median septum.
Splenius capitis
Splenius capitis arises from the mastoid process and the rough surface on the occipital bone just below the lateral third of the superior nuchal line. Its fibres pass downwards and medially to reach the midline. The lower fibres insert into the tips of the spinous processes of the seventh cervical and upper three or four thoracic vertebrae and the intervening supraspinous ligaments. The tendons of the upper fibres interlace in the midline with those of the opposite side in the dorsal raphe of the ligamentum nuchae in the lower half of the cervical region (Fig. 42.53).
Splenius cervicis
Splenius cervicis is confluent with splenius capitis, but covers more caudal regions of the neck and thoracic region (Fig. 42.53). It arises from the transverse process of the atlas, the tip of the transverse process of the axis and the posterior tubercle of the third cervical vertebra. Its fibres pass downwards and medially, wrapping around the other posterior intrinsic neck muscles, to insert into the third to sixth thoracic spinous processes.
Erector spinae
Erector spinae (Fig. 42.6, Fig. 42.7, Fig. 42.54, Fig. 42.55) is a large musculotendinous mass which differs in size and composition at different vertebral levels. It consists of fascicles that assume systematic attachments to homologous parts of the skull, the cervical, thoracic, and lumbar vertebrae, the sacrum, and the ilium. Individual muscles are defined by the attachments of their fascicles and the regions that they span. There are three muscles each with three regional parts:
Spinalis | Longissimus | Iliocostalis |
---|---|---|
Spinalis thoracis | Longissimus capitis | Iliocostalis cervicis |
Spinalis cervicis | Longissimus cervicis | Iliocostalis thoracis |
Spinalis capitis | Longissimus thoracis | Iliocostalis lumborum |
Longissimus cervicis is a long thin muscle that arises by tendons from the posterior tubercles of the transverse processes of the second to sixth cervical vertebra. It descends into the thoracic region, between the tendons of longissimus capitis and longissimus thoracis, to insert by tendons into the transverse processes of the upper four or five thoracic vertebrae.
From the upright posture, the trunk can flex forwards under the influence of gravity. This movement is initiated by flexor muscles, such as rectus abdominis, in order to bring the centre of gravity of the trunk forwards. Thereafter, erectores spinae control the descent of the thorax under gravity. When the trunk is fully flexed, many parts of erector spinae cease to contract and become electromyographically silent. In this position, flexion is limited by passive tension in the back muscles, and tension in the thoracolumbar fascia, the posterior spinal ligaments, and the intervertebral discs. Similarly, lateral flexion under gravity is controlled by the contralateral erector spinae, with input from the abdominal oblique muscles.
Spinotransverse group
Rotatores | Multifidus | Semispinalis |
---|---|---|
Rotatores thoracis | Multifidus | Semispinalis cervicis |
Rotatores cervicis | Semispinalis thoracis | |
Rotatores capitis | Semispinalis capitis |
Rotatores thoracis (Fig. 42.56) consist of eleven pairs of small quadrilateral muscles. The first pair lies between the first and second thoracic vertebrae, and the last between the 11th and 12th thoracic vertebrae. One or more may be absent from the upper or lower ends of the series. In each segment, a rotator brevis connects the lower border and lateral surface of the lamina above to the upper, posterior part of the transverse process of the vertebra immediately below; a rotator longus connects the base of the spinous process above to the transverse process two levels below. Rotatores are not obviously present at lumbar and cervical levels, where they may be represented by some of the deeper fascicles of multifidus.
At cervical, thoracic, and upper lumbar levels, multifidus lies lateral to the spinous processes and covers the laminae of the underlying vertebrae, but at lumbosacral levels it expands to cover the posterior surface of the sacrum (Fig. 42.57). At each segmental level, multifidus is formed by several fascicles that arise from the caudal edge of the lateral surface of the spinous process and from the caudal end of its tip. They radiate caudally to insert into the transverse elements of vertebrae two, three, four and five levels below (Macintosh et al 1986). These sites are represented at cervical levels by the superior articular processes, at thoracic levels by the posterior surface of each transverse process near its base, and at lumbar levels by the mammillary processes. Fascicles that extend beyond the fifth lumbar vertebra insert into the dorsal surface of the sacrum. The longest fascicles from the first and second lumbar vertebrae insert into the dorsal segment of the iliac crest. From each spinous process the shortest fascicles pass inferiorly and laterally to their insertion; the longer fascicles assume a progressively steeper course and are arranged progressively more medially. The fascicles from a given segment are flanked and overlapped dorsolaterally by fascicles from successively higher segments, an arrangement that endows the intact muscle with a laminated structure.
The semispinalis muscles are formed by the longest fascicles of the spinotransverse group. Three divisions are defined essentially by their origins (Fig. 42.58).
Vascular supply of the true back muscles
The detailed pattern of the arterial supply of the deep muscles of the back has been described by Michel Salmon (Taylor & Razaboni 1994). These muscles are supplied by dorsal branches of the posterior intercostal and lumbar arteries. In the thoracic and upper lumbar regions, where the components of erector spinae run in well-defined longitudinal columns, arterial trunks from these dorsal branches run in the sulci between the columns and between erector spinae and multifidus, giving off branches to supply the muscles. This vascular pattern is less regular in the lumbar region.
Suboccipital muscles
The suboccipital muscles (Fig. 42.59) are four small muscles that connect the occipital bone, atlas and axis posteriorly. They lie inferior to the anterior part of the occipital bone, where three of the muscles form the boundaries of the suboccipital triangle: rectus capitis posterior major lies above and medially; obliquus capitis superior lies above and laterally, and obliquus capitis inferior lies below and laterally. With the head in the anatomical position the suboccipital triangle lies almost in the horizontal plane.
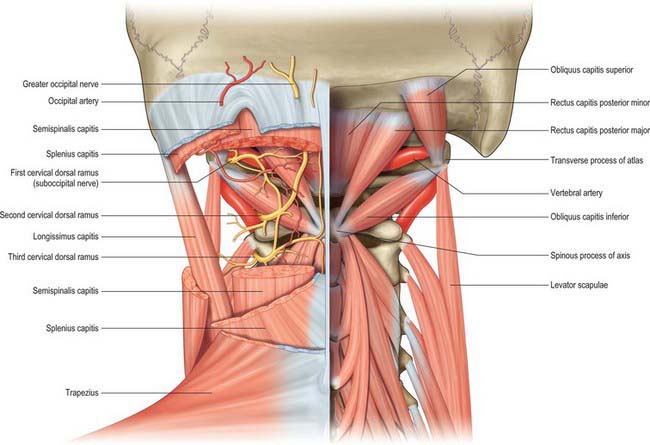
Fig. 42.59 Posterior view of the suboccipital triangles.
(From Drake, Vogl, Mitchell, Tibbitts and Richardson 2008.)
Rectus capitis posterior minor
Rectus capitis posterior minor is attached by a narrow pointed tendon to the tubercle on the posterior arch of the atlas. As it ascends it broadens before attaching to the medial part of the inferior nuchal line and to the occipital bone between the inferior nuchal line and the foramen magnum. Either muscle may be doubled longitudinally. There is usually a soft-tissue attachment to the posterior atlanto-occipital membrane, which itself is firmly attached anteriorly to the spinal dura in the same area (Zumpano et al 2006).
MOVEMENTS OF THE VERTEBRAL COLUMN
Spinal movements between individual vertebrae cannot be measured accurately by skin-surface techniques: only biplanar radiography is sufficient for this purpose. The best values for the normal adult lumbar spine are from Pearcy (1984a,b). Movements of the entire lumbar spine can be measured using skin-surface techniques, but the results are of limited clinical use because there is high inter- and intra-observer variation.
Cervical motion can be considered to involve the upper (i.e. the atlanto-occipital and atlanto-axial complexes) and the lower cervical spine (C3–7). Two physiological movements take place at the atlanto-occipital joints, those of flexion-extension and lateral flexion. The atlanto-axial joints allow flexion-extension and rotation. Some studies have suggested that maximum flexion-extension occurs between the occiput and C1; however, Frobin et al (2002) noted between 12.6° and 14.5° at this level, which is less than at some of the other cervical levels. Global cervical flexion ranges from 45° to 58° depending on the method of assessment, age and sex: older subjects and females exhibit less motion (Ordway et al 1997; Trott et al 1996). At an intersegmental level, motion increases from the second cervical level, peaks at the mid cervical level (14–17° recorded at C4/5), before reducing at the junction of the cervical and thoracic spine (9.8–11.5° noted at C6/7) (Frobin et al 2002). Global ranges of lateral flexion range from 32° to 47°, with a gradual reduction in range with age and sex, while rotational movements range from 63° to 78°. Intersegmental ranges of motion vary from 4.7° to 6° for lateral flexion between C2 and C7, and 2–12° for rotation (White & Panjabi 1990).
Movement in the thoracic spine is frequently regionalized to upper, mid- and lower thoracic. In the upper thoracic, flexion ranges from 7.8° to 9.5°, increasing to 10–11.4° in the mid-thoracic and 12.5° to 12.8° in the lower thoracic (Willems et al 1996). Extension is more consistent, ranging from 7.1° to 9.7° throughout the thoracic spine. Lateral flexion is greater in the lower thoracic spine, ranging from 5.6° to 6.2° in the upper thoracic, 7.9° to 8.1° in the mid-thoracic and 11.9° to 13.2° in the lower thoracic. However, rotation is greatest in the mid-thoracic region, being 11.8° to 15.9° in the upper thoracic, 21.5° to 25.3° in the mid-thoracic, and 8.3° to 11.8° in the lower thoracic.
During flexion of the lumbar spine there is an unfolding or straightening of the lumbar lordosis. Thus in full flexion the lumbar spine assumes a straight alignment or is curved slightly forwards. Normal ranges of global lumbar flexion range from 58° to 72° in under 40-year-olds and 40° to 60° in the over forties: females exhibit a reduced range compared with males (McGregor et al 1995; Dvorák et al 1995). At an intersegmental level, the L3/4 junction and L4/5 junction exhibit the greatest mobility, approximately 12° and 13° respectively, while at the lowest level (L5/S1) there is only 9°, and at the upper lumbar levels only 8° and 10° respectively (Pearcy et al 1984). Movements into extension are the converse of those seen in flexion. Normal ranges of global extension range from 25° to 30° in under 40-year-olds and 15° to 20° in those over forty. At an intersegmental level, L5/S1 and L1/2 exhibit the greatest mobility at approximately 5°, while the remaining levels exhibit less than 5° of extension. Ranges of lateral flexion and rotation in the lumbar spine are reduced compared to other regions of the spine. Global lateral flexion ranges from 20° to 35°, and rotation ranges from 25° to 40°: the ranges of both movements are reduced with age. Assessment of intersegmental rotation and lateral flexion has proven difficult because of the limitations of measurement techniques.
FACTORS INVOLVED IN STABILITY
Full stability and load-bearing capacity both require intact vertebral bodies and intervertebral discs. Earlier views regarding the relative importance of the disc-body complex and the posterior elements have proved somewhat simplistic. Clinical observation led to the ‘three-column concept’ of spinal stability (Denis 1983), in which the column is divided into three longitudinal parts rather than two. The anterior column is formed by the anterior longitudinal ligament, the anterior half of the vertebral body and the anterior anulus fibrosus. The middle column is made up of the posterior longitudinal ligament, the posterior half of the vertebral body and pedicles and the posterior anulus fibrosus. The posterior column consists of the neural arch and facet joints and the posterior ligamentous complex. Failure of any column can lead to some instability, the status of the middle column being the most important. The more columns that are affected, the worse is the instability: an injury to two columns is usually unstable.
Both body height and spinal stability are subject to a marked diurnal variation. Body height is affected by changes from recumbency to the upright posture. These diurnal variations appear to be due to changes that occur within the cervical, thoracic and lumbar regions of the spine. Investigations using stereophotogrammetry have demonstrated that 40% of diurnal changes occur in the thoracic spine and affect the degree of kyphosis, and a further 40% in the lumbar spine, but do not affect the lordosis (Wing et al 1992). The greatest change in vertebral column length is found in adolescents and young adults. The height loss occurs within 3 hours of rising in the morning, with an overall loss of about 15 mm.
All ligaments of the column, as well as the facet joint capsules, are important in the maintenance of stability. The anterior longitudinal ligament is very strong, and resists translational displacement (shear) of the vertebrae as well as extension. All the ligaments of the posterior complex resist flexion and rotation, and their integrity determines the range of movements allowed. These ligaments can support the whole column when the muscles are inactive, e.g. in quiet standing. At the limit of lumbar flexion the column is supported mainly by the thoracolumbar fascia and by collagenous tissue within the electrically silent muscles of the back.
POSTURE AND ERGONOMICS
Ergonomics has been defined as ‘the way humans work’, and it permits an appreciation of the effects of tasks and the work environment on underlying postural biomechanics. Nachemson (1975) showed that discs were loaded maximally in sitting and in lifting in a forward leaning position, so sitting posture and lifting have received considerable ergonomic attention.
SURFACE ANATOMY
SKELETAL LANDMARKS
Vertebral spines
In the midline a median furrow runs from the external occipital protuberance above to the natal cleft below (Fig. 42.60, Fig. 42.61, Fig. 42.62). The furrow is most shallow in the lower cervical region and is deepest in the midlumbar zone. Inferiorly, it widens out into a flattened, triangular area, the apex of which lies at the start of the natal cleft and corresponds to the third sacral spine. Palpation of the median furrow reveals the sagittal curves of the spine: the cervical curve is convex forwards (lordosis) and extends from the first cervical to the second thoracic vertebra; the thoracic curvature is concave forwards (kyphosis) and extends from the second to the 12th thoracic vertebra; the lumbar curvature is convex forwards and extends from the 12th thoracic vertebra to the lumbosacral prominence. The external occipital protuberance is subcutaneous and can be felt and often seen (Fig. 42.61), and it can be palpated without difficulty when it is approached from below. The inion is the point situated on this protuberance in the median plane. The tips of the spines of the cervical vertebrae are obscured by the overlying ligamentum nuchae. The tubercle on the posterior arch of the atlas is impalpable; the first bony prominence which is encountered when the finger is drawn downwards in the midline from the external occipital protuberance is the spine of the second cervical vertebra. The ligamentum nuchae terminates inferiorly at the spine of the seventh cervical vertebra, which is the highest, and sometimes the only, visible projection in this region (vertebra prominens). Immediately below this the spine of the first thoracic vertebra is palpable; it is usually more prominent than the seventh cervical vertebra. The spine of the second thoracic vertebra can also often be felt. The third thoracic spinous process is level with the spine of the scapula (Fig. 42.60), and the seventh with the inferior scapular angle when the arm is by the side. The identification of the remaining thoracic spines is not easy, even in a thin subject when the trunk is fully flexed, because of the manner in which they overlap one another in the midthoracic region. In the upper and lower thoracic regions, the tips of the thoracic spines lie opposite the upper part of the body of the immediately subjacent vertebra. In the midthoracic region, they lie opposite the lower part of the vertebra below. The tip of the spine of each lumbar vertebra can usually be palpated without difficulty, especially if the trunk is flexed. Each lies opposite the inferior part of its own body. The body of the fourth lumbar vertebra is level with the summits of the iliac crests, so the fourth lumbar spine overlies the L4/5 interspace (a point useful in lumbar puncture). The second sacral spine is level with the posterior superior iliac spines. Sacral and coccygeal landmarks are described below.
Spinal levels of viscera
The palpable vertebral spines can be used as landmarks for the levels of the viscera. Some of the more important are shown in Table 42.1 (minor differences between the two sides are ignored).
Spine | Viscera |
---|---|
C5 | Cricoid cartilage, start of oesophagus |
C7 | Apex of lung |
T3 | Aorta reaches spine. Tracheal bifurcation |
T4 | Aortic arch ends. Upper border of heart |
T8 | Lower border of heart. Central tendon of diaphragm |
T10 | Lower border of lung. Cardia of stomach. Upper border of kidney |
T12 | Lowest level of pleura. Pylorus |
L1 | Hilum of kidney. Renal arteries. Superior mesenteric artery |
L2 | Spinal cord terminates. Pancreas. Duodenojejunal flexure |
L3 | Lower border of kidney |
L4 | Bifurcation of aorta |
L5 | Inferior vena cava begins |
Scapular landmarks
The scapula overlies the second to seventh ribs. Its superior angle is palpable beneath trapezius, and its inferior angle is just covered by latissimus dorsi: these angles are joined by the medial border of the scapula which runs vertically (Fig. 42.60, Fig. 42.61). The scapular spine runs subcutaneously and is easily palpable from its root medially to the acromion process laterally.
The root of the spine of the scapula lies opposite the third thoracic spine and the inferior angle lies opposite the seventh thoracic spine when the arm is by the side.
Posterior pelvic and sacrococcygeal bony landmarks
At the lower part of the back the iliac crest can be palpated throughout its whole length, and can be traced backwards and upwards from the anterior superior iliac spine to its highest point, and then downwards and medially to the posterior superior iliac spine, which is overlain by a dimple in the skin (Fig. 42.61). A line joining these sacral dimples passes through the second sacral spine and the body of the second sacral vertebra, and is a useful landmark for the lower end of the adult dural sac. There may also be a less prominent pair of dimples at the level of the L4/5 intervertebral disc. The posterior superior iliac spine lies over the centre of the sacroiliac joint. The supracristal plane joins the highest points of the iliac crest on each side. It passes through the body of the fourth lumbar vertebra and has been used as a clinical landmark when performing a lumbar puncture (p. 760) though difficulty in its definition reduces its reliability in this procedure. The tip of the coccyx can be felt deeply near the centre of the natal cleft. As the examining finger passes cranially, the sacral cornua can be felt on either side: these demarcate the sacral hiatus, and form the landmark for performing a caudal anaesthetic block.
MUSCULOTENDINOUS LANDMARKS
In the upper and middle cervical region the median furrow lies between the cylindrical prominences formed mainly by the semispinalis muscles, which are accentuated by neck extension against resistance. In the thoracic and lumbar regions, a broad elevation produced by the erector spinae muscle group extends for about one hand’s breadth on either side of the median furrow and is present between the iliac crest and the 12th rib (Fig. 42.62). The lateral border of this elevation then crosses the ribs at their angles, passing medially as it ascends. The muscle group can be demonstrated by extending the back against resistance.
Trapezius is a flat, triangular muscle that covers the back of the neck and shoulder (Fig. 42.61). Together, the two trapezius muscles resemble a trapezium or quadrilateral in which two of the angles correspond to the shoulders, a third to the occipital protuberance and the fourth to the spine of the 12th thoracic vertebra. The two muscles cover the back of the neck and shoulders like a monk’s cowl, hence the ancient name of the trapezius was musculus cucullaris. The upper part of trapezius is demonstrated by elevation of the shoulders, or by extension and lateral flexion of the neck, against resistance. The lower fibres are best seen when the subject pushes both hands hard against a wall with the elbows extended. The anterosuperior border of the muscle forms the posterior boundary of the posterior triangle of the neck and can be seen in muscular subjects, especially during elevation of the shoulder against resistance.
In a well-muscled subject the outline of latissimus dorsi can easily be traced, particularly if the arm is adducted against resistance. The triangle of auscultation (Fig. 42.52) lies between the upper border of latissimus dorsi, the lower lateral border of trapezius and the medial border of the scapula.
The lumbar triangle (Fig. 42.52), one of the sites of the rare primary lumbar hernia, lies inferiorly, between the lowermost outer border of latissimus dorsi, the posterior free border of external oblique, and the iliac crest.
Adams MA, Bogduk N, Burton K, Dolan P. The Biomechanics of Back Pain, 2nd edn. Edinburgh: Churchill Livingstone, 2006.
Batson OV. The vertebral vein system. Am J Roentgenol. 1957;78:195-212.
Boelderl A, Daniaux H, Kathrein A, Maurer H. Danger of damaging the medial branches of the posterior rami of spinal nerves during a dorsomedian approach to the spine. Clin Anat. 2002;15:77-81.
Bogduk N. Clinical Anatomy of the Lumbar Spine and Sacrum, 4th edn. Edinburgh: Elsevier Churchill Livingstone, 2005.
Crock HV. Atlas of Vascular Anatomy of the Skeleton and Spinal Cord. London: Martin Dunitz, 1996.
Crock HV, Yoshizawa H. The blood supply of the lumbar vertebral column. Clin Orthop. 1976;115:6-21.
Dean NA, Mitchell BS. Anatomic relation between the nuchal ligament (ligamentum nuchae) and the spinal dura mater in the craniocervical region. Clin Anat. 2002;15:182-185.
Denis F. The three column spine and its significance in the classification of acute thoracolumbar spinal injuries. Spine. 1983;8:817-831.
Seminal paper for the understanding and classification of spinal instability..
Dvorák J, Vajda EG, Grob D, Panjabi MM. Normal motion of the lumbar spine related to age and gender. Eur Spine J. 1995;4:18-23.
Frobin W, Leivseth G, Biggeman M, Brinckmann P. Sagittal plane segmental motion of the cervical spine. A new precision measurement protocol and normal motion data of healthy adults. Clin Biomech. 2002;17:21-31.
Groen G, Baljet B, Drukker J. The nerves and nerve plexuses of the human vertebral column. Amer J Anat. 1990;188:282-296.
Lang J. Craniocervical region, osteology and articulations. Neuro Orthop. 1986;1:67-92.
Loughenbury PR, Wadhwani S, Soames RW. The posterior longitudinal ligament and peridural (epidural) membrane. Clin Anat. 2006;19:487-492.
Macintosh JE, Valencia F, Bogduk N, Munro RR. The morphology of the lumbar multifidus muscles. Clin Biomech. 1986;1:196-204.
MacLaughlin SM, Oldale KNM. Vertebral body diameters and sex prediction. Ann Hum Biol. 1992;19:285-293.
Describes the archaeological and forensic examination of skeletal material..
Macnab I, McCulloch J. Backache, 2nd edn. Baltimore: Williams and Wilkins, 1990. Chapter 1.
McGregor AH, McCarthy ID, Hughes SPF. Motion characteristics of the lumbar spine in the normal population. Spine. 1995;20(22):2421-2428.
Mercer S, Bogduk N. The ligaments and anulus fibrosus of human adult cervical intervertebral discs. Spine. 1999;24:619-628.
Mercer SR, Bogduk N. Clinical anatomy of ligamentum nuchae. Clin Anat. 2003;16:484-493.
Nachemson A. Towards a better understanding of low-back pain: a review of the mechanics of the lumbar disc. Rheumatol Rehab. 1975;14:129-143.
Newell RLM. The spinal epidural space. Clin Anat. 1999;12:375-379.
Review of the morphological, developmental and topographical aspects of the spinal epidural space..
Ordway NR, Seymour R, Donelson RG, Hojnowski L, Lee E, Edwards T. Cervical sagittal range of motion using three methods. Spine. 1997;22:501-508.
Pearcy M, Protek I, Shepherd J. Three-dimensional X-ray analysis of normal movement in the lumbar spine. Spine. 1984;9:294-297.
Pearcy M, Tibrewal SB. Axial rotation and lateral bending in the normal lumbar spine. Spine. 1984;9:582-587.
Piper JG, Traynelis VC. Congenital malformations of the craniovertebral junction. In: Dickman CA, Spetzler RF, Sonntag VKH. Surgery of the Craniovertebral Junction. New York: Thieme, 1998.
Taylor GI, Razaboni RM. Michel Salmon: Anatomic Studies, Book 1. Arteries of the Muscles of the Extremities and Trunk. St Louis: Quality Medical Publishing, 1994.
Taylor JR, Twomey LT. Sexual dimorphism in human vertebral shape. J Anat. 1984;138:281-286.
Trott PH, Pearcy MJ, Ruston SA, Fulton I, Brien C. Three-dimensional analysis of active cervical motion: the effect of age and gender. Clin Biomech. 1996;11:201-206.
Twomey L, Taylor J, Furniss B. Age changes in the bone density and structure of the lumbar vertebral column. J Anat. 1983;136:15-25.
White AA, Panjabi MM. Clinical Biomechanics of the Spine, 2nd edn. Philadelphia: JB Lippincott, 1990.
Willems JM, Jull GA, Ng JK-F. An in vivo study of the primary and coupled rotations of the thoracic spine. Clin Biomech. 1996;11:311-316.
Wing P, Tsang I, Gagnon F, Susak L, Gagnon R. Diurnal changes in the profile shape and range of motion of the back. Spine. 1992;17:761-765.
Zumpano MP, Hartwell S, Jagos CS. Soft tissue connection between rectus capitis posterior minor and the posterior atlanto-occipital membrane. Clin Anat. 2006;19:522-527.