8 Systemic Inflammation
Numerous advances in perioperative care have allowed increasingly high-risk patients to safely undergo cardiac surgery. Although mortality rates of 1% are quoted for “low-risk” cardiac surgery, results from large series of patients older than 65 years suggest that mortality rates are actually more substantial.1 For example, Birkmeyer et al1 reviewed a large number (n = 474,108) of “all-comers” undergoing coronary artery bypass surgery (CABG) or aortic valve surgery in the Medicare Claims Database. Notably, 30-day all-cause mortality was in the range of 4.0% to 5.4% after CABG and 6.5% to 9.1% after aortic valve replacement. The patient population studied, although elderly (age > 65), would not be considered to be particularly high risk by today’s standards. These data do not point to the cause of death. Nevertheless, they indicate that outcome after routine cardiac surgery is poor for many patients. Outcome after these procedures is even worse if the extent of postoperative complications is considered. Postoperative morbidity is common,2 and complications include atrial fibrillation, poor ventricular function requiring inotropic agents, and noncardiac-related causes such as infection, gastrointestinal dysfunction, acute lung injury, stroke, and renal dysfunction. For example, in Rady et al’s3 large series of patients ≥ 75 years of age undergoing cardiac surgery (n = 1,157), the mortality rate was 8%. The rate of serious complications, however, exceeded 50%.
Many postoperative complications appear to be caused by an exaggerated systemic proinflammatory response to surgical trauma.4–6 The most severe form of this inflammatory response leads to multiple organ dysfunction syndrome and death.5,6 Milder forms of a proinflammatory response cause less severe organ dysfunction, which does not lead to admission to an intensive care unit (ICU), but nevertheless causes suffering, increased hospital length of stay, and increased cost. The cause and clinical relevance of systemic inflammation after cardiac surgery are poorly understood. Systemic inflammation is a multifactorial process and has profound secondary effects on both injured and normal tissues. Proinflammatory mediators can have beneficial as well as deleterious effects on multiple organ systems. According to most theories, tissue injury, endotoxemia, and contact of blood with the foreign surface of the cardiopulmonary bypass (CPB) circuit are some of the major factors postulated to initiate a systemic inflammatory response. Nevertheless, controversy surrounds the cause and pathogenesis of inflammation in the perioperative period.
Terminology
The terminology of inflammation is confusing and has hampered effective communication among scientists and clinicians. Despite attempts to standardize the terminology, variation in usage still exists in the scientific literature, as well as the clinical setting.7 Much of the confusion relates to the term inflammation, defined as “a fundamental pathologic process consisting of a dynamic complex of cytologic and chemical reactions that occur in the affected blood vessels and adjacent tissues in response to an injury or abnormal stimulation caused by a physical, chemical, or biologic agent, including (1) the local reactions and resulting morphologic changes, (2) the destruction or removal of the injurious material, (3) the responses that lead to repair and healing.”8 This definition acknowledges the potential role of noninfectious causative factors; that is, infection is not a prerequisite for the development of inflammation. The American College of Chest Physicians/Society of Critical Care Medicine Consensus Conference has developed definitions for terms related to inflammation (Table 8-1). Figure 8-1 demonstrates the possible interrelations among many of these terms.
Infection = microbial phenomenon characterized by an inflammatory response to the presence of microorganisms or the invasion of normally sterile host tissue by those organisms. |
Bacteremia = the presence of viable bacteria in the blood. |
Systemic inflammatory response syndrome (SIRS) = the systemic inflammatory response to a variety of severe clinical insults. The response is manifested by two or more of the following conditions: (1) temperature > 38°C or < 36°C; (2) heart rate > 90 beats per minute; (3) respiratory rate > 20 breaths per minute or PaCO2 < 32 mm Hg; and (4) white blood cell count > 12,000/mm3, < 4000/mm3, or > 10% immature (band) forms. |
Sepsis = the systemic response to infection, manifested by two or more of the following conditions as a result of infection: (1) temperature > 38°C or < 36°C; (2) heart rate > 90 beats per minute; (3) respiratory rate > 20 breaths per minute or PaCO2 < 32 mm Hg; and white blood cell count > 12,000/mm3, < 4000/mm3, or > 10% immune (band) forms. |
Severe sepsis = sepsis associated with organ dysfunction, hypoperfusion, or hypotension. Hypoperfusion and perfusion abnormalities may include, but are not limited to, lactic acidosis, oliguria, or an acute alteration in mental status. |
Septic shock = sepsis-induced with hypotension despite adequate fluid resuscitation together with the presence of perfusion abnormalities that may include, but are not limited to, lactic acidosis, oliguria, or an acute alteration in mental status. Patients who are receiving inotropic or vasopressor agents may not be hypotensive at the time that perfusion abnormalities are measured. |
Sepsis-induced hypotension = a systolic blood pressure < 90 mm Hg or a reduction of ≥ 40 mm Hg from baseline in the absence of other causes for hypotension. |
Multiple organ dysfunction syndrome (MODS) = presence of altered organ function in an acutely ill patient such that homeostasis cannot be maintained without intervention. |
From Bone RC, Balk RA, Cerra FB, et al: Definitions for sepsis and organ failure and guidelines for the use of innovative therapies in sepsis: ACCP/SCCM consensus conference. Chest 101:1644–1655, 1992.
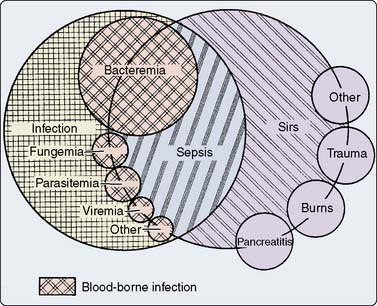
Figure 8-1 The interrelations among systemic inflammatory response syndrome (SIRS), sepsis, and infection.
(From Bone RC, Balk RA, Cerra FB, et al: Definitions for sepsis and organ failure and guidelines for the use of innovative therapies in sepsis: ACCP/SCCM Consensus Conference. Chest 101:1644–1655, 1992.)
In the surgical population, the use of the phrase and definition for SIRS has generated some controversy.9 This controversy relates to the fact that almost all patients after major surgery fulfill the criteria for SIRS. Most patients, however, clearly do not develop clinically significant organ dysfunction from their systemic inflammation. Critics may argue that the use of the term SIRS in cardiac surgery patients, therefore, is meaningless because it does not differentiate patients who will have a benign versus a complicated postoperative course. For these reasons, SIRS is used more commonly by investigators than by practicing physicians. Use of the phrase SIRS, nevertheless, has the benefit of increasing awareness regarding the many noninfectious causes of inflammation.
The acute-phase response to tissue injury and infection is characterized by leukocytosis, fever, increased vascular permeability, a negative nitrogen balance, changes in plasma steroid and metal concentrations, and increased synthesis of hepatic acute-phase proteins. Examples of these proteins include haptoglobin, fibrinogen, C-reactive protein (CRP), complement factors (C3, factor B), serum amyloid A, α1-acid glycoprotein, and α1-antichymotrypsin.10 The terms acute-phase response and systemic inflammation often are used interchangeably.
A common misconception relates to the terms bacteremia and endotoxemia. Whereas bacteremia refers to the presence of viable bacteria in the blood, endotoxemia refers to the presence of endotoxin in the blood. Endotoxin, also known as lipopolysaccharide (LPS), is a component of the cell membranes of gram-negative bacteria, and hence its presence does not require the existence of viable organisms. In fact, it has been clearly established that cardiac surgical patients have a high incidence of intraoperative endotoxemia despite simultaneously exhibiting a low incidence of culture-proven bacteremia. This observation is consistent with the observation that “sterile” instruments and solutions, including intravenous fluids and the CPB circuit, may be contaminated with endotoxin.11
Systemic Inflammation and Cardiac Surgery
The systemic inflammatory response after cardiac surgery is multifactorial. As described earlier, the term SIRS is not particularly helpful in clarifying the pathophysiology of inflammation in cardiac surgery.9 A schematic of the inflammatory process is depicted in Figure 8-2. There does not appear to be much disagreement with the statement that all of these processes may happen and may be responsible for causing complications in cardiac surgical patients. Tissue injury, endotoxemia, and contact of blood with the foreign surface of the CPB circuit are thought to initiate a systemic inflammatory response after cardiac surgery. What is least understood and of most controversy is the issue of which of these many processes is the most clinically relevant. It appears as if major surgery is an important cause of systemic inflammation, and that CPB further exacerbates the elaboration of proinflammatory mediators. Various causes and mediators of inflammation are reviewed in the subsequent sections.

Mechanisms of Inflammation-Mediated Injury
It is not entirely clear how inflammation ultimately damages cells and organ systems. Activation of neutrophils and other leukocytes is central to most theories regarding inflammation-induced injury.6,12–15 Neutrophil activation leads to the release of oxygen radicals, intracellular proteases, and fatty acid (i.e., arachidonic acid) metabolites. These products, as well as those from activated macrophages and platelets, can cause or exacerbate tissue injury.
In localized areas of infection, oxygen free radicals liberated by activated neutrophils aid in the destruction of pathogens.16 Complement, in particular, C5a, results in activation of leukocytes and oxygen free radical formation.17 These activated neutrophils liberate toxic amounts of oxygen free radicals such as hydrogen peroxide, hydroxyl radicals, and superoxide anion. Oxygen free radicals are thought to cause cellular injury, ultimately through damage to the lipid membrane.18–20 Increased levels of lipid peroxidation products, that is, products of oxidation of membrane lipids such as malondialdehyde, are thought to reflect the severity of free radical cellular damage.21 Consistent with this model of injury, Royston et al22 demonstrated increased levels of peroxidation products in cardiac surgical patients. In another study, oxygen free radicals were found to be increased in 21 patients undergoing cardiac surgery; however, the clinical relevance of these changes was not studied.21
A related mechanism of injury results from the degranulation of neutrophils. Activated neutrophils release granules that contain myeloperoxidase, as well as other toxic digestive enzymes such as neutrophil elastase, lactoferrin, β-glucuronidase, and N-acetyl-β-glucosaminidase.23–26 Release of these intracellular enzymes not only causes tissue damage but also reduces the number of cells that can participate in bacterial destruction. In one study, cardiac surgical patients who developed splanchnic hypoperfusion, a possible cause of inflammation, demonstrated increased neutrophil degranulation and increased plasma neutrophil elastase concentrations.26
Another mechanism of inflammation-mediated injury involves microvascular occlusion. Activation of neutrophils leads to adhesion of leukocytes to endothelium and formation of clumps of inflammatory cells, that is, microaggregates.14,27 Activated leukocytes have less deformable cell membranes, which affects their ability to pass through capillaries.28 Microaggregates can cause organ dysfunction through microvascular occlusion and reductions in blood flow and oxygen at the local level.22,28,29 After the disappearance of these microaggregates and restoration of microvascular flow, reperfusion injury may occur.

Physiologic Mediators of Inflammation
Cytokines
Cytokines are believed to play a pivotal role in the pathophysiology of acute inflammation associated with cardiac surgery.30,31 Cytokines are proteins released from activated macrophages, monocytes, fibroblasts, and endothelial cells, which have far-reaching regulatory effects on cells.32 They are small proteins that exert their effects by binding to specific cell-surface receptors. Many of these proteins are called interleukins because they aid in the communication between white blood cells (leukocytes).
Tumor Necrosis Factor
Endotoxemia unequivocally results in initiation of proinflammatory pathways, most likely through stimulation of TNF.33–36 Michie et al33 administered endotoxin intravenously to human volunteers and detected peak levels of TNF 90 to 180 minutes later. Peak concentrations of TNF correlated with increased temperature and heart rate (HR), as well as circulating levels of adrenocorticotropic hormone and epinephrine. In this and other studies, TNF levels soon appear after a proinflammatory stimulus and disappear quickly, which helps explain a common finding from clinical studies. TNF levels are often not increased when measured in patients with systemic inflammation, probably because test samples are obtained long after exposure to the primary inflammatory stimulus. This issue of sampling time may partially account for the fact that some cardiac surgical studies have detected increased TNF levels, whereas others have not.37–53
Interleukins
After the appearance of TNF, levels of IL-1 increase in cardiac surgical patients.47,50,52,54 Measured levels are low and may peak within several hours after CPB.54 Others have demonstrated maximum levels 1 day after cardiac surgery, which may explain the inability of some investigators to detect IL-1 during the intraoperative period.50 IL-1 may decrease systemic vascular resistance after CPB through induction of nitric oxide synthesis in vascular endothelial cells.55 Although IL-1 appears to be important in the initiation and propagation of the inflammatory cascade, it is not clear whether IL-1 levels cause deleterious effects or even serve as a marker for patients who will develop organ dysfunction after cardiac surgery. Some of the reported effects of IL-1 may be due instead to other cytokines, in particular, TNF, which are detected at the same time.
IL-8 is also believed to be an important component of the proinflammatory cascade. It is a potent chemoattractant of neutrophils to the site of injury or infection. IL-8 also is responsible for the activation, priming, and degranulation of neutrophils.56,57 The relevance of increases in IL-8 levels to outcome after cardiac surgery has not been established.42–44,46,47,51,53,58,59 Rothenburger et al60,61 observed a significant association between prolonged mechanical ventilation and postoperative IL-8 levels but not IL-6 levels.
IL-6 levels have been shown to increase in the setting of cardiac surgery, although this is not a universal finding.10,42,43,45–48,51–54,58,62–65 Peak levels of this cytokine appear after maximum values for TNF and IL-1. For example, Steinberg et al54 measured plasma cytokine levels in 29 patients undergoing CPB. IL-6 levels peaked at 3 hours after separation from CPB and remained increased 24 hours after surgery. No association was found between IL-6 levels and hemodynamic parameters or postoperative pulmonary function.
Anti-inflammatory Cytokines
The regulation of inflammation is complex and involves a balance between proinflammatory and anti-inflammatory cytokines. IL-10 is a potent inhibitor of the synthesis of TNF, IL-1, IL-6, and IL-8, and increases in the perioperative period.44,66–68 McBride et al68 obtained blood samples perioperatively from 20 patients undergoing cardiac surgery. Before and during CPB, increases were observed in the proinflammatory cytokines TNF, IL-1, and IL-8. At the same time that proinflammatory cytokine levels began to decrease, increases in the anti-inflammatory cytokines IL-10 and IL-1ra were observed. The authors suggest that the balancing effects of these two types of cytokines may determine whether a patient suffers from the effects of excessive systemic inflammation (i.e., postoperative organ dysfunction) or the effects of inadequate immune system enhancement (i.e., postoperative infection and poor wound healing). Using this theory to improve outcome has not been translated yet into a clinical trial involving surgical patients. One concern related to potentially deleterious effects of inhibiting proinflammatory mediators has been borne out in sepsis trials in which mortality was increased in the group given an anti-inflammatory agent.69 An understanding of the interaction between proinflammatory and anti-inflammatory mediators may result in the development of an effective and safe approach to reducing complications related to excessive systemic inflammation.
Complement System
The complement cascade is illustrated in Figure 8-3. The complement cascade can be triggered by either the classic pathway or the alternate pathway. In the alternate pathway, C3 is activated by contact of complement factors B and D with complex polysaccharides, endotoxin, or exposure of blood to foreign substances such as the CPB circuit. Contact activation (Figure 8-4) describes contact of blood with a foreign surface with resulting adherence of platelets and activation of factor XII (Hageman factor). Activated factor XII has numerous effects, including initiation of the coagulation cascade through factor XI and conversion of prekallikrein to kallikrein. Kallikrein leads to generation of plasmin, which is known to activate the complement and the fibrinolytic systems. Kallikrein generation also activates the kinin-bradykinin system.
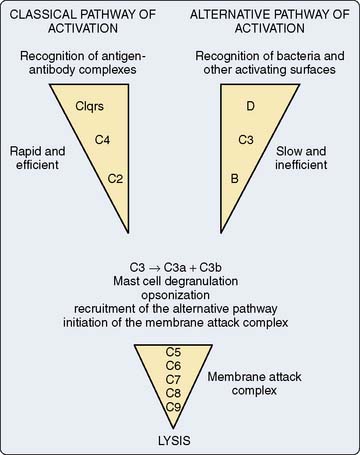
Figure 8-3 Simplified components of the complement system.
(From Haynes BF, Fauci AS: Introduction to clinical immunology. In Braunwald E, Isselbacher KJ, Petersdorf RG, et al [eds]: Harrison’s principles of internal medicine, 11th ed. New York: McGraw-Hill, 1987, pp 328–337.)
The classic pathway involves the activation of C1 by antibody-antigen complexes. In the case of cardiac surgery, there are two likely mechanisms for the activation of the classic pathway. Endotoxin can be detected in the serum of almost all patients undergoing cardiac surgery. Endotoxin forms an antigen-antibody complex with antiendotoxin antibodies normally found in serum, which can then activate C1. The administration of protamine after separation from CPB has been reported to result in heparin/protamine complexes, which also can activate the classic pathway70,71 (see Chapters 28 through 31). Others, however, have not observed this effect.72 Contact activation leads to activation of factor XII, which results in the generation of plasmin. Plasmin is capable of activating complement factors C1 and C3. Table 8-2 is a summary of the physiologic effects of the complement system.
TABLE 8-2 Biologically Significant Effects of the Various Complement-Split Products
Biologic Effect | Complement-Split Products |
---|---|
Mast cell degranulation, contraction of smooth muscle, increased vascular permeability | C3a, C5a |
Chemotaxis of neutrophils | C5a, C5a des Arg |
Neutrophil aggregation | C5a, C5a des Arg |
Lysosomal enzyme release | C5a, C3b |
Leukocytosis | C3e |
Immune adherence/opsonization | C3b, C4b |
Membrane lysis | C5b-9 (membrane attack complex) |
From Knudsen F, Anderson LW: Immunological aspects of cardiopulmonary bypass. J Cardiothorac Anesth 4:245, 1990.
Although some elements of complement activation have been elucidated, clinicians are only now learning about the clinical relevance of this process to patients undergoing cardiac surgery. Several studies have reported increased complement levels during cardiac surgery.38,58,73–78 Chenoweth et al73 measured plasma C3a and C5a levels at different time points in 15 adults undergoing cardiac surgery with CPB. Although C3a levels were not affected by surgical stimulation, complement activation increased significantly during CPB (Figure 8-5). This and other studies did not test the association between increased complement levels and adverse postoperative outcome. Thus, they do not provide any evidence that complement activation causes clinically significant systemic inflammation. Kirklin et al.75 measured plasma C3a levels in 116 patients undergoing cardiac surgery with CPB and 12 patients undergoing operations without CPB. In this study, an increase of complement activation during CPB was associated with postoperative morbidity. Patients undergoing procedures without CPB did not demonstrate increases in complement. This result suggests that a factor unique to CPB causes activation of complement. This study, however, did not pinpoint the clinical relevance of complement activation or of the CPB circuit, in part because even patients without postoperative morbidity had increased complement levels. Furthermore, confounding factors capable of causing SIRS, such as endotoxin, were not measured and accounted for in this study.
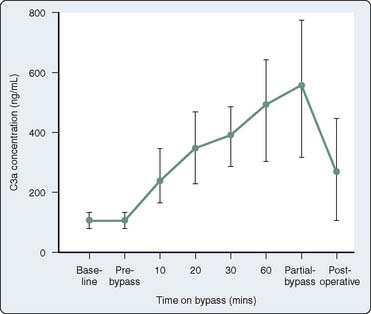
Figure 8-5 Plasma levels of C3a in patients undergoing cardiopulmonary bypass.
(From Chenowith DE, Cooper SW, Hugli TE, et al: Complement activation during cardiopulmonary bypass: Evidence for generation of C3a and C5a anaphylatoxins. N Engl J Med 304:497, 1981. Copyright 1981 Massachusetts Medical Society. All rights reserved.)
The results from several large, randomized clinical trials in which complement activation was selectively blocked have become available.79–81 These studies indicate that attenuation of complement activation results in less myocardial injury; however, there did not appear to be an impact on complications such as pulmonary and renal dysfunction and severe vasodilation. These results suggest that complement activation may not play as large a role in the development of systemic inflammation-mediated morbidity as previously thought. These trials are discussed in more detail later in this chapter.
Endotoxin
Endotoxin, also called LPS, is a component of the cell membrane of gram-negative bacteria. It is a potent activator of complement and cytokines, and appears to be one of the initial triggers of systemic inflammation, as summarized in Figure 8-2.12,82–84 Although the LPS constituent varies from one bacterial species to another, it generally may be described with reference to Figure 8-6 as consisting of three structural regions: (a) lipid A, (b) core, and (c) O-polysaccharide outer region. The lipid region of lipid A is embedded in the outer leaflet of the outer membrane. The oligosaccharide core region is positioned between lipid A and the O-polysaccharide outer region. Lipid A has the same basic structure in practically all gram-negative bacteria and is the toxic component of endotoxin. The LPS core region shows a high degree of similarity among various bacteria. It usually consists of a limited number of sugars. For example, the inner core region is constituted of heptose and 3-deoxy-d-manno-2-octulosonate (KDO) residues, whereas the outer core region comprises galactose, glucose, or N-acetyl-d-glucosamine residues displayed in various manners depending on the strain. The O-polysaccharide outer region (also called O-specific antigen or O-specific side chain) is highly variable and is composed of one or more oligosaccharide repeating units characteristic of the serotype.
Endotoxemia
Endotoxemia refers to the presence of endotoxin in the blood. Endotoxemia is common in cardiac surgical patients.10,11,38,41,60,64,65,85–95 It is not surprising that some investigators have failed to detect endotoxemia during cardiac surgery given its transient and intermittent nature, although differences in endotoxin-assaying techniques used also may contribute to this discrepancy.51,52,96,97 Andersen et al11 measured circulating endotoxin levels in 10 patients undergoing cardiac surgery. All preoperative blood samples were free of endotoxin; however, substantial levels of endotoxin were detected intraoperatively. Blood endotoxin levels from eight typical patients undergoing cardiac surgery are presented in Figure 8-7.85 Although endotoxin can be found in sterile fluids administered to patients, it is believed that the majority of endotoxin arises through a patient’s impaired gut barrier.11 Rothenburger et al60 studied the association of endotoxin levels with prolonged mechanical ventilation in 78 cardiac surgical patients. Endotoxin levels were three times greater in patients with a postoperative mechanical ventilation time longer than 24 hours (n = 13) compared with patients with ventilator time less than 24 hours.
Normally, intestinal flora contain a large amount of endotoxin from gram-negative microorganisms.98 The average human colon contains approximately 25 billion nanograms of endotoxin, which is an enormous quantity when 300 ng endotoxin is toxic to humans.33,34 The leakage of live bacterial cells into the bloodstream can result in infection as these viable bacteria multiply.99 However, many of the bacteria in the intestine are dead, and thus endotoxin also can enter the bloodstream contained within cell membrane fragments of dead bacteria. In this case, infection per se does not develop. Instead, endotoxin may initiate a systemic inflammatory response through potent activation of macrophages and other proinflammatory cells.100 A plasma endotoxin concentration of only 1 ng/mL has been reported to be lethal in humans.101
On entry to the bloodstream, endotoxin forms complexes with numerous intravascular compounds including high-density lipoprotein, LPS-binding protein, and endotoxin-specific immunoglobulins. Endotoxin has been linked to dysfunction in every organ system of the body and may be the key initiating factor in the development of systemic inflammation.12,82–84,93

Normal Host Defenses against Endotoxemia
Early Tolerance
If endotoxemia is deleterious to patients, it would be logical to assume that patients have defense mechanisms against this ubiquitous toxin. Tolerance to endotoxin was studied extensively by Greisman and Hornick in the early 1970s.102 Two distinct types of tolerance to endotoxin exist and are classified as early tolerance and late tolerance.102 Early tolerance to endotoxin represents a reduction in the proinflammatory effects of LPS when administered several hours after a prior infusion of LPS.103 It appears to be due to an LPS-induced refractory state of macrophages in which they release less TNF in response to endotoxin. This early refractive state shows no LPS specificity and can be overcome with increased doses of endotoxin. The degree of this tolerance is directly proportional to the dose, and hence intensity of the initial LPS-induced inflammatory state. Early tolerance begins within hours of LPS exposure and decreases almost to baseline within 2 days. It cannot be transferred with plasma. Early tolerance may protect the host from lethal systemic inflammation after an overwhelming exposure of LPS.
Late Tolerance
Late tolerance to endotoxin is due to the synthesis of immunoglobulins, that is, antibodies, directed against the offending LPS.102 Late tolerance begins approximately 72 hours after exposure to LPS, which correlates with the appearance of the early-appearing IgM class of antibodies. This form of tolerance persists for at least 2 weeks and correlates with the presence of serum immunoglobulins. In contrast with early tolerance, the late response is not proportional to the intensity of the initial LPS-induced inflammatory response but is related to the immunogenicity of the initial LPS. Furthermore, late tolerance does not generally protect against a subsequent challenge with a dissimilar type of LPS. In other words, late tolerance is most pronounced when the same, that is, homologous, LPS serotype is used for both the initial and the subsequent challenge. It is not definitively understood how antiendotoxin antibodies responsible for late tolerance confer protection from LPS-induced systemic inflammation. Proposed mechanisms include increased clearance of endotoxin into the reticuloendothelial system, as well as direct neutralization through binding.
Understanding of the host’s normal humoral defense against endotoxin is further complicated because of the numerous serotypes of endotoxin.98 Serotype-specific antibodies, that is, antibodies synthesized in response to a particular LPS, exhibit high-affinity binding to and protection from the specific serotype of endotoxin. These serotype, that is, O-specific, antiendotoxin antibodies, however, do not recognize the many possible variations of endotoxin O-polysaccharide side chains, and thus are ineffective at conferring protection against the numerous serotypes of endotoxin likely to be encountered in the clinical setting. Antibodies, however, directed against the well-conserved inner core structure of endotoxin should theoretically be cross-reactive against many clinically relevant serotypes of endotoxin. Cardiac surgical patients are exposed to a wide variety of endotoxin types. For example, at least 164 O-antigens exist for Escherichia coli, the gram-negative bacteria most commonly isolated in high-risk surgical and ICU patients.104,105

Criticisms of Endotoxin as a Causative Factor
There are several criticisms of the theory that endotoxemia is an important cause of postoperative morbidity. A common criticism relates to the low incidence of culture-proven bacteremia in surgical and ICU patients.106–109 Endotoxemia, however, is clearly prevalent in these patients and usually exists in the setting of negative blood cultures.10,11,24,38,41,64,65,85–95 In fact, studies attempting to detect endotoxemia probably underestimate its incidence given its intermittent nature.
The failure of two anti-lipid A monoclonal antibodies (HA-1A, Centocor, Malvern, PA; and E5, Xoma, Berkeley, CA) to improve outcome on an “intention-to-treat” basis in ICU patients with established sepsis also has been used to suggest that endotoxemia is not clinically relevant.110,111 These monoclonal antibodies may not bind to endotoxin with high affinity, which may explain, in part, their lack of demonstrable efficacy.112 In addition, they were tested in patients with established sepsis and organ failure, which is an entirely different setting from elective surgical patients, who are more likely to benefit from prophylaxis with endotoxin-neutralizing drugs. Arguing against the clinical relevance of endotoxin is the negative result from a trial of prophylactic administration of a lipid A antagonist (E5564) in cardiac surgery.113
Splanchnic Perfusion
Splanchnic hypoperfusion appears to be an important cause of systemic inflammation.114–117 The gut is one of the most susceptible organs to hypoperfusion during conditions of trauma or stress.117–119 In the 1960s, Price et al118 removed 15% of the blood volume from healthy volunteers, causing a 40% reduction in splanchnic blood volume. In this study, cardiac output (CO), blood pressure (BP), and HR did not change from baseline. A study was conducted by Hamilton-Davies et al, in which 25% of the blood volume was removed from six healthy volunteers.120 Gastric mucosal perfusion, as measured by saline tonometry, was the first variable to decline (in five of the six subjects). Stroke volume (SV) also decreased; however, routinely measured cardiovascular variables such as HR, BP, and CO did not change significantly enough from baseline values to cause suspicion of a hypovolemic state. Based on these types of studies, the Advanced Trauma Life Support (ATLS) course teaches that a 15% blood loss (Class I hemorrhage) rarely results in changes in HR, BP, or urine output.121 Significant decreases in systolic BP are a late sign of shock, which typically occurs after Class III hemorrhage (30–40% blood loss).
These studies suggest that during periods of hypovolemia, the gut vasoconstricts, thus shunting blood toward “more vital organs” such as the heart and brain.117–119 In addition to hypovolemia, endogenously released vasoconstrictors during CPB, such as angiotensin II, thromboxane A2, and vasopressin, also may result in decreased splanchnic perfusion.122–125