19 Subcutaneous Implantable Cardioverter-Defibrillators
In the almost four decades since Michel Mirowski implanted the first automatic defibrillator, these devices have transitioned from controversial to a routine facet of patient care.1,2 At that pivotal moment in 1972, Dr. Mirowski was under tremendous scrutiny and heavy skepticism.3,4 The original device that he implanted was rudimentary by present standards, with a crude, probability-density detection algorithm for sensing ventricular fibrillation (VF) and the requirement of thoracotomy for placement of an epicardial electrode system. Nevertheless, this device performed reasonably well, despite its simple imperative of shocking VF using a coil in the superior vena cava and a patch over the cardiac apex. We remember Dr. Mirowski arguing passionately at the scientific meetings, when more complex devices were introduced, that his original device, a shock-only defibrillator, was all that was needed. Although no clinician currently would argue that a shock-only device is sufficient in the care of patients with diverse diseases, Mirowski’s passionate adherence to that premise should not be summarily dismissed in an age when the basic technology of an implanted defibrillator has become subservient to many, less critical functions. This chapter revisits Dr. Mirowski’s vision after almost 40 years, with some technologic twists along the way.
Background
Successive waves of technologic advances have created the modern, sophisticated device comprising a modest-sized, electrically active generator (i.e., active can) implanted pectorally, utilizing defibrillation-efficient biphasic waveforms, one to three transvenously inserted leads, and antibradycardia/antitachycardia and biventricular pacing capability, as well as programmable detection algorithms and defibrillation energy strengths. The success of this technology is mirrored by the estimated 200,000 devices implanted worldwide in 2009. Despite these remarkable achievements, implantable cardioverter-defibrillators (ICDs) still generate controversy, particularly in the United States, where both physicians and the public are questioning ICD reliability and safety. This peaked in 2009 with the withdrawal of the Fidelis lead from the market amid extensive and often critical publicity.5–11 Further complaints over lead reliability continue, together with concerns over engineering reliability.6,11
The growing number of lead failures has exposed the Achilles heel of ICDs: the need to attach an electrode directly to the heart via the vascular system. The complicated path that the lead must follow, the hostile environment of the body, and the requirement that leads be supple combine to threaten lead integrity. Moreover, the necessary adherence of the electrode tip to the myocardium and potential attachment of the body of the lead to the veins traversed result in an appreciable risk of injury and death if lead extraction becomes necessary. Reported ICD lead “survival” rates vary from 85% to 98% at 5 years and 60% to 72% at 8 years; Figure 19-1 plots data from 10 studies.10 Table 19-1 summarizes the reported incidence of complications when lead extraction is judged necessary, with mortality ranging from zero to 2.7%, the latter figure relating to associated overwhelming sepsis.12–19 These concerns, along with the well-known complications of routine transvenous lead insertion (perforation, tamponade, pneumothorax, hemothorax, sepsis, endocarditis), indicated the need to develop an ICD that would not require transvenous leads.
Early Investigation Experience with Subcutaneous Defibrillation
In August 2001 a collaborative research group explored the index question of how much energy was needed for subcutaneous defibrillation. With appropriate ethics committee approval and patient consent, the first test of subcutaneous defibrillation was performed in humans at Green Lane Hospital in Auckland, New Zealand.20 The original test was conducted in a 56-year-old man undergoing transvenous ICD implantation because of a recent cardiac arrest. A small incision was made over the low lateral chest wall to permit tunneling of an anteriorly and posteriorly situated subcutaneous oval disk electrode of approximately 5 cm2 in surface area. A standard transvenous right ventricular lead was used to induce ventricular fibrillation, which was terminated, to the team’s satisfaction, with the first shock, chosen at a strength of 70 joules using a biphasic waveform with a 50% tilt and 100-µF capacitance. This shock resulted in success at an energy level that was much less than was then known possible with transthoracic defibrillation, using two large surface pad electrodes. At that time, the lower limit of average defibrillation threshold efficacy was presumably 100 to 115 J. Clearly, avoiding the resistive effect of the skin was surprisingly beneficial. As discussed later, the energy requirements proved to be even more remarkable.
Although defibrillation with our initial configuration was successful, the posterior space proved too difficult to access and therefore was subsequently abandoned. Attempting to access a posterior subcutaneous space from an anterior approach with a supine patient clearly was impractical, regardless of how low the defibrillation energy would prove to be. Four more patients were tested using various anterior/anterolateral configurations in the latter half of 2001, and defibrillation was successful in all. Each of these pilot explorations led to a more definitive and sequential approach to vector and electrode selection. Our original intention had been to develop a curved generator, resembling a flattened banana, which would mold to the natural contour of the intercostal space (Fig. 19-2). In fact, early prototypes with this shape were tested, but unfortunately it proved too difficult to accommodate the necessary components of the generator to this design and was reluctantly abandoned. Subsequent studies undertaken with additional help (Johannes Sperzel, Jorg Neuzner, and Stefan Spritzer in Germany; Andrew Grace at Papworth Hospital in the United Kingdom; Andrei Ardashev at Burdenko Hospital in Moscow, as well as at Green Lane; Jon Hunt of Cameron Health) allowed rapid progress in identifying a practical lead system.
Optimal Defibrillation Configuration
Having demonstrated proof of concept, the research aim was then to evaluate systematically which of many electrode configurations would be most effective, as well as most practical from a surgical perspective. Although multiple systems were examined and abandoned, four viable configurations were eventually chosen for detailed study. In this next phase of development, these four defibrillation systems compared the utility of retaining the conventional pectoral generator site versus a novel, left lateral placement of the generator. In addition, a variety of electrode shapes, lengths, and positions were examined. The specific four combinations chosen were as follows (Fig. 19-3):
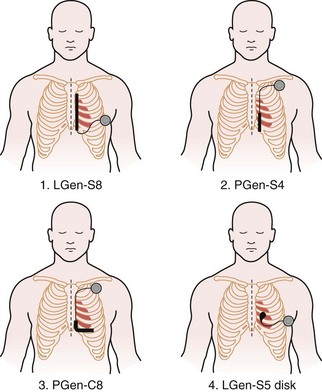
Figure 19-3 Four lead systems acutely tested for the “optimal” system.
(Modified from Bardy GH, Smith WM, Hood MA, et al: An entirely subcutaneous implantable cardioverter-defibrillator, N Engl J Med 363:36-44, 2010.)
Defibrillation testing of these temporary lead configurations was completed immediately before permanent transvenous ICD implantation in a total of 78 patients in a number of centers, most at Green Lane and Papworth Hospitals. These data have been published previously.21 A Latin Square design was used for testing with analysis of variance (ANOVA). A step-up/step-down protocol was used to determine defibrillation thresholds (DFTs) using a 50%-tilt biphasic waveform. An initial test shock of 40 J was delivered after 10 seconds of induced VF, incrementing to a maximum of 80 J or decrementing to a minimum of 10 J. Bardy et al.21 fully describe the DFT testing protocol. Unsuccessful shocks were followed by prompt, transthoracic rescue defibrillation.
For the four configurations just described, the mean DFT (±SD) was as follows, respectively (Fig 19-4):
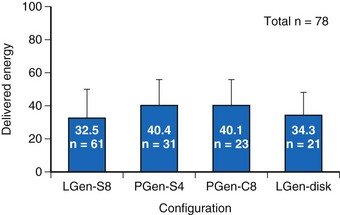
Figure 19-4 Delivered defibrillation threshold energies.
Defibrillation threshold data (mean ± SD) for four practical lead/electrode configurations (see Fig. 19-3) in acute human trials (total of 78 patients).
(Modified from Bardy GH, Smith WM, Hood MA, et al: An entirely subcutaneous implantable cardioverter-defibrillator, N Engl J Med 363:36-44, 2010.)