Chapter 12 Subarachnoid Hemorrhage
Advances in imaging technology have marked the great milestones in the history of the diagnosis and management of aneurysmal subarachnoid hemorrhage (SAH) and its complications. The development of cerebral angiography allowed in vivo visualization of saccular aneurysms, accomplished for the first time by Egas Moniz in 1933.1 It was not until 1951 that Ecker and Riemenschneider achieved the first angiographic proof of delayed vasospasm.2 Since its introduction in the 1970s, computed tomography (CT) scan has become the most reliable tool for the acute diagnosis of SAH. It did not take long before it was recognized that the amount of blood in the subarachnoid cisterns (Figure 12-1) was highly predictive of the subsequent risk of vasospasm.3–5 Transcranial Doppler (TCD) later proved valuable for noninvasive screening and monitoring of vasospasm.6 More recently, magnetic resonance imaging (MRI) techniques have enhanced our understanding of ischemic damage after SAH7 and may represent a valuable tool for the acute diagnosis of ongoing ischemia from vasospasm.8 As spiral CT angiograms9 and CT perfusion scans10 move into the scene, we may see another transformation in the management of SAH led by imaging technology in the immediate future.
Timely and accurate diagnosis of SAH hinges on the use of brain imaging. CT scan remains by far the most widely applied technique. Its sensitivity for the acute diagnosis of SAH exceeds 95%.11 However, CT scan is most reliable early after the bleeding (particularly within the first 24–48 hours) and false-negative results become possible after a few days. Gradient-recall echo T2* MRI has comparable sensitivity to CT scan for the hyperacute diagnosis of SAH,12 and fluid-attenuated inversion recovery (FLAIR) MRI has greater sensitivity than CT scan in subacute cases (Figure 12-2).13
Currently, the use of MRI is limited because MR technology is often unavailable in the emergency setting, and CT scanning allows faster image acquisition (which becomes an important factor in patients with acute SAH who are neurologically or hemodynamically unstable) and easier visualization of extravasated blood (at least for examiners without extensive radiological training). False-positive diagnoses of SAH (pseudo-SAH) by CT scan may be caused by increased venous congestion, as seen in patients with massive cerebral edema or bilateral subdural hematomas.14–16
Although it is generally agreed that the amount of subarachnoid blood visualized on CT scan predicts the risk of subsequent vasospasm, there is still debate regarding the best way to quantify this risk. The grading originally proposed by C. M. Fisher et al. is still the most popular (Table 12-1; Figure 12-3).5 However, newer visual scales17 (Table 12-1) and software-based techniques18 have claimed better sensitivity and specificity. CT scan is also the modality of choice for the prompt diagnosis of hydrocephalus and rebleeding, and it clearly demonstrates the presence of global cerebral edema, a strong predictor of unfavorable outcome.19
TABLE 12-1 Predicting delayed cerebral ischemia by the appearance of the initial CT scan of the brain: Comparison of the traditional Fisher radiological grading scale and a proposed modification that incorporates the impact of IVH on the risk of vasospasm and delayed ischemic damage
Grade | Fisher scale | Modified Fisher scale |
---|---|---|
0 | No SAH or IVH | |
1 | No SAH or IVH | Minimal/thin SAH, no IVH in both lateral ventricles |
2 | Diffuse, thin SAH, no clot > 1 mm in thickness | Minimal/thin SAH with IVH in both lateral ventricles |
3 | Thick layer of SAH of localized subarachnoid clot > 1 mm in thickness | Thick SAH*, no IVH in both lateral ventricles |
4 | Predominant IVH or intracerebral hemorrhage without thick SAH | Thick SAH* with IVH in both lateral ventricles |
CT, computed tomography; IVH, intraventricular hemorrhage; SAH, subarachnoid hemorrhage.
* Completely filling ≥ 1 cistern or fissure.
Conventional cerebral angiography remains the gold standard for the diagnosis of intracranial aneurysms (Figure 12-4).20 Whereas noninvasive angiograms may be sufficient for screening of unruptured intracranial aneurysms, diagnosis of nontraumatic SAH demands the performance of catheter angiography. Newer imaging processing techniques currently allow three-dimensional reconstruction of the aneurysm, which greatly enhances the anatomical definition of the study.21 After diagnosis, endovascular coiling may be performed during the same catheterization. Conventional angiography is also considered the gold standard for the diagnosis of vasospasm and enables its treatment with angioplasty or superselective intra-arterial infusion of vasodilators in selected cases.22,23
The role of MRI in the management of SAH and vasospasm continues to grow. Initially it was used to define the extent of delayed ischemic damage with exclusive prognostic purposes. MRI has considerably greater sensitivity than CT scan for the recognition of small foci of subcortical ischemia that commonly occur as sequelae of SAH, predominantly affecting the basal ganglia, claustrum, and frontal white matter.7 More recently, diffusion-weighted imaging and perfusion-weighted imaging have started to be employed for the acute detection of cerebral ischemia during the phase of vasospasm.8,24,25 These techniques may demonstrate hypoperfusion and ischemia even in patients with no definite evidence of vasospasm by either TCD or angiogram, suggesting that mechanisms other than luminal narrowing of large intracranial vessels may be responsible.
CT-based protocols combining CT perfusion with spiral CT angiograms may constitute a valuable alternative to the current practice of TCD screening and angiographic confirmation of vasospasm. These CT studies may provide direct evidence of the presence and magnitude of hypoperfusion and correlate that information with a fast and noninvasive depiction of the state of the major intracranial vessels.8–10,26 Further research to confirm the validity of these CT-based protocols is necessary to determine their optimal application.
As our understanding of the complex pathophysiological underpinnings of vasospasm improves, it appears increasingly evident that ischemia after SAH may be caused by disruption of microcirculatory function.20,27 New imaging modalities capable of providing dynamic information on the status of the microcirculation are likely to be the best candidates to forge the next revolution in the care of patients with SAH.
A 46-year-old woman developed thunderclap headache immediately followed by transient loss of consciousness associated with tonic posturing deemed suspicious for a seizure. She was emergently transferred to a local hospital. On initial examination, her Glasgow coma scale sum score was 13. She did not have cranial nerve deficits or hemiparesis (WFNS grade II). Her CT scan of the brain showed extensive SAH with a thick cisternal clot in the interpeduncular cistern (Fisher grade 3; Figure 12-5, upper row). There was also incipient hydrocephalus and a small left parasagittal frontal hematoma. Digital substraction angiography (DSA) disclosed a saccular aneurysm in the anterior communicating artery region, arising from the left A1 segment (Figure 12-5, lower row, left panel). The anatomical configuration of the aneurysm was much better visualized on the three-dimensional reconstructed images (Figure 12-5, lower row, left panel). The ruptured aneurysm was successfully coiled on the second day after the bleeding. Four days later, the patient developed some deterioration in the level of consciousness associated with doubling of the mean blood flow velocities in the left terminal carotid segment and the left A1 and M1 segments; similar but less severe changes were present on the right anterior circulation. The diagnosis of diffuse, severe vasospasm was confirmed angiographically. The patient improved rapidly with hemodynamic augmentation therapy and had no other complications. At 3 months, she still had mild neurobehavioral problems and occasional headaches. Six months later, she was feeling well and had returned to work, although she reported decreased endurance.
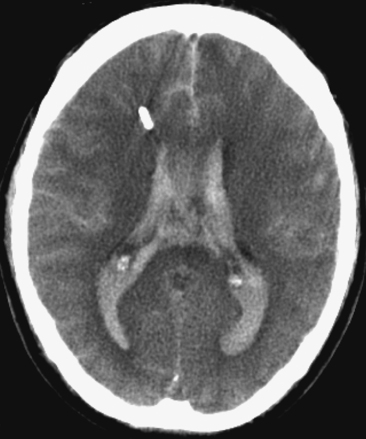
Figure 12-7 Example of extensive subarachnoid hemorrhage and intraventricular hemorrhage (“casted ventricles”).
CAUSES OF EARLY DETERIORATION: REBLEEDING AND HYDROCEPHALUS
GLOBAL CEREBRAL EDEMA
CEREBRAL VASOSPASM
VASOSPASM: ENDOVASCULAR TREATMENT
PATTERNS OF ISCHEMIA
SUBARACHNOID HEMORRHAGE WITH NEGATIVE ANGIOGRAM
Perimesencepahlic Subarachnoid Hemorrhage
Non-Perimesencephalic Subarachnoid Hemorrhage with Negative Angiogram
CONDITIONS MIMICKING ANEURYSMAL SUBARACHNOID HEMORRHAGE
Pseudo-Subarachnoid Hemorrhage
Traumatic Subarachnoid Hemorrhage
Causes of Sulcal Subarachnoid Hemorrhage
1 Moniz E. Anéurysme intra-crânien de la carotide interne droite rendu visible pa l’arteriographie cérébrale. Rev Otoneuroophtalmol. 1933;11:746-748.
2 Ecker A, Riemenschneider PA. Arteriographic demonstration of spasm of the intracranial arteries with special reference to saccular arterial aneurysms. J Neurosurg. 1951;8:660-667.
3 Takemae T, Mizukami M, Kin H, Kawase T, Araki G. [Computed tomography of ruptured intracranial aneurysms in acute stage—relationship between vasospasm and high density on CT scan (author’s transl.)]. No To Shinkei. 1978;30:861-866.
4 Kistler JP, Crowell RM, Davis KR, Heros R, Ojemann RG, Zervas T, et al. The relation of cerebral vasospasm to the extent and location of subarachnoid blood visualized by CT scan: a prospective study. Neurology. 1983;33:424-436.
5 Fisher CM, Kistler JP, Davis JM. Relation of cerebral vasospasm to subarachnoid hemorrhage visualized by computerized tomographic scanning. Neurosurgery. 1980;6:1-9.
6 Sloan MA, Haley ECJr, Kassell NF, Henry ML, Stewart SR, Beskin RR, et al. Sensitivity and specificity of transcranial Doppler ultrasonography in the diagnosis of vasospasm following subarachnoid hemorrhage. Neurology. 1989;39:1514-1518.
7 Shimoda M, Takeuchi M, Tominaga J, Oda S, Kumasaka A, Tsugane R. Asymptomatic versus symptomatic infarcts from vasospasm in patients with subarachnoid hemorrhage: serial magnetic resonance imaging. Neurosurgery. 2001;49:1341-1348.
8 Leclerc X, Fichten A, Gauvrit JY, Riegel B, Steinling M, Lejeune JP, et al. Symptomatic vasospasm after subarachnoid haemorrhage: assessment of brain damage by diffusion and perfusion-weighted MRI and single-photon emission computed tomography. Neuroradiology. 2002;44:610-616.
9 Goldsher D, Shreiber R, Shik V, Tavor Y, Soustiel JF. Role of multisection CT angiography in the evaluation of vertebrobasilar vasospasm in patients with subarachnoid hemorrhage. AJNR Am J Neuroradiol. 2004;25:1493-1498.
10 Harrigan MR, Magnano CR, Guterman LR, Hopkins LN. Computed tomographic perfusion in the management of aneurysmal subarachnoid hemorrhage: new application of an existent technique. Neurosurgery. 2005;56:304-317.
11 van der Wee N, Rinkel GJ, Hasan D, van Gijn J. Detection of subarachnoid haemorrhage on early CT: is lumbar puncture still needed after a negative scan? J Neurol Neurosurg Psychiatry. 1995;58:357-359.
12 Sohn CH, Baik SK, Lee HJ, Lee SM, Kim IM, Yim MB, et al. MR imaging of hyperacute subarachnoid and intraventricular hemorrhage at 3T: a preliminary report of gradient echo T2*-weighted sequences. AJNR Am J Neuroradiol. 2005;26:662-665.
13 Noguchi K, Ogawa T, Seto H, Inugami A, Hadeishi H, Fujita H, et al. Subacute and chronic subarachnoid hemorrhage: diagnosis with fluid-attenuated inversion-recovery MR imaging. Radiology. 1997;203:257-262.
14 Cucchiara B, Sinson G, Kasner SE, Chalela JA. Pseudo-subarachnoid hemorrhage: report of three cases and review of the literature. Neurocrit Care. 2004;1:371-374.
15 Given CA, Burdette JH, Elster AD, Williams DWIII. Pseudo-subarachnoid hemorrhage: a potential imaging pitfall associated with diffuse cerebral edema. AJNR Am J Neuroradiol. 2003;24:254-256.
16 Rabinstein AA, Pittock SJ, Miller GM, Schindler JJ, Wijdicks EF. Pseudosubarachnoid haemorrhage in subdural haematoma. J Neurol Neurosurg Psychiatry. 2003;74:1131-1132.
17 Claassen J, Bernardini GL, Kreiter K, Bates J, Du YE, Copeland D, et al. Effect of cisternal and ventricular blood on risk of delayed cerebral ischemia after subarachnoid hemorrhage: the Fisher scale revisited. Stroke. 2001;32:2012-2020.
18 Friedman JA, Goerss SJ, Meyer FB, Piepgras DG, Pichelmann MA, McIver JI, et al. Volumetric quantification of Fisher Grade 3 aneurysmal subarachnoid hemorrhage: a novel method to predict symptomatic vasospasm on admission computerized tomography scans. J Neurosurg. 2002;97:401-407.
19 Claassen J, Carhuapoma JR, Kreiter KT, Du EY, Connolly ES, Mayer SA. Global cerebral edema after subarachnoid hemorrhage: frequency, predictors, and impact on outcome. Stroke. 2002;33:1225-1232.
20 Rabinstein AA, Wijdicks EF. Cerebral Vasospasm in subarachnoid hemorrhage. Curr Treat Options Neurol. 2005;7:99-107.
21 Beck J, Rohde S, Berkefeld J, Seifert V, Raabe A. Size and location of ruptured and unruptured intracranial aneurysms measured by 3-dimensional rotational angiography. Surg Neurol. 2006;65:18-25.
22 Polin RS, Coenen VA, Hansen CA, Shin P, Baskaya MK, Nanda A, et al. Efficacy of transluminal angioplasty for the management of symptomatic cerebral vasospasm following aneurysmal subarachnoid hemorrhage. J Neurosurg. 2000;92:284-290.
23 Elliott JP, Newell DW, Lam DJ, Eskridge JM, Douville CM, Le Roux PD, et al. Comparison of balloon angioplasty and papaverine infusion for the treatment of vasospasm following aneurysmal subarachnoid hemorrhage. J Neurosurg. 1998;88:277-284.
24 Beck J, Raabe A, Lanfermann H, Seifert V, Weidauer S. Tissue at risk concept for endovascular treatment of severe vasospasm after aneurysmal subarachnoid haemorrhage. J Neurol Neurosurg Psychiatry. 2004;75:1779-1781.
25 Condette-Auliac S, Bracard S, Anxionnat R, Schmitt E, Lacour JC, Braun M, et al. Vasospasm after subarachnoid hemorrhage: interest in diffusion-weighted MR imaging. Stroke. 2001;32:1818-1824.
26 Wintermark M, Ko NU, Smith WS, Liu S, Higashida RT, Dillon WP. Vasospasm after subarachnoid hemorrhage: utility of perfusion CT and CT angiography on diagnosis and management. AJNR Am J Neuroradiol. 2006;27:26-34.
27 Hansen-Schwartz J. Cerebral vasospasm: a consideration of the various cellular mechanisms involved in the pathophysiology. Neurocrit Care. 2004;1:235-246.
28 Karttunen AI, Jartti PH, Ukkola VA, Sajanti J, Haapea M. Value of the quantity and distribution of subarachnoid haemorrhage on CT in the localization of a ruptured cerebral aneurysm. Acta Neurochir (Wien). 2003;145:655-661.
29 Friedman JA, Rabinstein AA, Meyer FB. Perisylvian frontotemporal hematoma due to rupture of an anterior communicating artery aneurysm. Case illustration. J Neurosurg. 2002;97:493.
30 Krishnaney AA, Rasmussen PA, Masaryk T. Bilateral tentorial subdural hematoma without subarachnoid hemorrhage secondary to anterior communicating artery aneurysm rupture: a case report and review of the literature. AJNR Am J Neuroradiol. 2004;25:1006-1007.
31 Smith ML, Abrahams JM, Chandela S, Smith MJ, Hurst RW, Le Roux PD. Subarachnoid hemorrhage on computed tomography scanning and the development of cerebral vasospasm: the Fisher grade revisited. Surg Neurol. 2005;63:229-234.
32 Dorai Z, Hynan LS, Kopitnik TA, Samson D. Factors related to hydrocephalus after aneurysmal subarachnoid hemorrhage. Neurosurgery. 2003;52:763-769.
33 Sheehan JP, Polin RS, Sheehan JM, Baskaya MK, Kassell NF. Factors associated with hydrocephalus after aneurysmal subarachnoid hemorrhage. Neurosurgery. 1999;45:1120-1127.
34 Vermeij FH, Hasan D, Vermeulen M, Tanghe HL, van Gijn J. Predictive factors for deterioration from hydrocephalus after subarachnoid hemorrhage. Neurology. 1994;44:1851-1855.
35 Findlay JM, Jacka MJ. Cohort study of intraventricular thrombolysis with recombinant tissue plasminogen activator for aneurysmal intraventricular hemorrhage. Neurosurgery. 2004;55:532-537.
36 Pietila TA, Heimberger KC, Palleske H, Brock M. Influence of aneurysm location on the development of chronic hydrocephalus following SAH. Acta Neurochir (Wien). 1995;137:70-73.
37 Grote E, Hassler W. The critical first minutes after subarachnoid hemorrhage. Neurosurgery. 1988;22:654-661.
38 Friedman JA, Goerss SJ, Meyer FB, Piepgras DG, Pichelmann MA, McIver JI, et al. Volumetric quantification of Fisher Grade 3 aneurysmal subarachnoid hemorrhage: a novel method to predict symptomatic vasospasm on admission computerized tomography scans. J Neurosurg. 2002;97:401-407.
39 Schmidt JM, Rincon F, Fernandez A, Resor C, Kowalski RG, Claassen J, et al. Cerebral infarction associated with acute subarachnoid hemorrhage. Neurocrit Care. 2007;7:10-17.
40 Baldwin ME, Macdonald RL, Huo D, Novakovic RL, Goldenberg FD, Frank JI, et al. Early vasospasm on admission angiography in patients with aneurysmal subarachnoid hemorrhage is a predictor for in-hospital complications and poor outcome. Stroke. 2004;35:2506-2511.
41 Sloan MA, Alexandrov AV, Tegeler CH, Spencer MP, Caplan LR, Feldmann E, et al. Assessment: transcranial Doppler ultrasonography: report of the Therapeutics and Technology Assessment Subcommittee of the American Academy of Neurology. Neurology. 2004;62:1468-1481.
42 Lysakowski C, Walder B, Costanza MC, Tramer MR. Transcranial Doppler versus angiography in patients with vasospasm due to a ruptured cerebral aneurysm: A systematic review. Stroke. 2001;32:2292-2298.
43 Lysakowski C, Walder B, Costanza MC, Tramer MR. Transcranial Doppler versus angiography in patients with vasospasm due to a ruptured cerebral aneurysm: A systematic review. Stroke. 2001;32:2292-2298.
44 Sviri GE, Lewis DH, Correa R, Britz GW, Douville CM, Newell DW. Basilar artery vasospasm and delayed posterior circulation ischemia after aneurysmal subarachnoid hemorrhage. Stroke. 2004;35:1867-1872.
45 Rabinstein AA, Friedman JA, Weigand SD, McClelland RL, Fulgham JR, Manno EM, et al. Predictors of cerebral infarction in aneurysmal subarachnoid hemorrhage. Stroke. 2004;35:1862-1866.
46 Suarez JI, Qureshi AI, Yahia AB, Parekh PD, Tamargo RJ, Williams MA, et al. Symptomatic vasospasm diagnosis after subarachnoid hemorrhage: evaluation of transcranial Doppler ultrasound and cerebral angiography as related to compromised vascular distribution. Crit Care Med. 2002;30:1348-1355.
47 Rabinstein AA, Weigand S, Atkinson JL, Wijdicks EF. Patterns of cerebral infarction in aneurysmal subarachnoid hemorrhage. Stroke. 2005;36:992-997.
48 Frontera JA, Rundek T, Schmidt JM, Claassen J, Parra A, Wartenberg KE, et al. Cerebrovascular reactivity and vasospasm after subarachnoid hemorrhage: a pilot study. Neurology. 2006;66:727-729.
49 Romano JG, Forteza AM, Concha M, Koch S, Heros RC, Morcos JJ, et al. Detection of microemboli by transcranial Doppler ultrasonography in aneurysmal subarachnoid hemorrhage. Neurosurgery. 2002;50:1026-1030.
50 Hertel F, Walter C, Bettag M, Morsdorf M. Perfusion-weighted magnetic resonance imaging in patients with vasospasm: a useful new tool in the management of patients with subarachnoid hemorrhage. Neurosurgery. 2005;56:28-35.
51 Pham M, Johnson A, Bartsch AJ, Lindner C, Mullges W, Roosen K, et al. CT perfusion predicts secondary cerebral infarction after aneurysmal subarachnoid hemorrhage. Neurology. 2007;69:762-765.
52 van der Schaaf I, Wermer MJ, van der Graaf Y, Hoff RG, Rinkel GJ, Velthuis BK. CT after subarachnoid hemorrhage: relation of cerebral perfusion to delayed cerebral ischemia. Neurology. 2006;66:1533-1538.
53 Rosenwasser RH, Armonda RA, Thomas JE, Benitez RP, Gannon PM, Harrop J. Therapeutic modalities for the management of cerebral vasospasm: timing of endovascular options. Neurosurgery. 1999;44:975-979.
54 Bejjani GK, Bank WO, Olan WJ, Sekhar LN. The efficacy and safety of angioplasty for cerebral vasospasm after subarachnoid hemorrhage. Neurosurgery. 1998;42:979-986.
55 Srinivasan J, Moore A, Eskridge J, Winn HR, Newell DW. Long-term follow up of angioplasty for cerebral vasospasm. Acta Neurochir Suppl. 2001;77:195-197.
56 Eskridge JM, McAuliffe W, Song JK, Deliganis AV, Newell DW, Lewis DH, et al. Balloon angioplasty for the treatment of vasospasm: results of first 50 cases. Neurosurgery. 1998;42:510-516.
57 Linskey ME, Horton JA, Rao GR, Yonas H. Fatal rupture of the intracranial carotid artery during transluminal angioplasty for vasospasm induced by subarachnoid hemorrhage. Case report. J Neurosurg. 1991;74:985-990.
58 Liu JK, Couldwell WT. Intra-arterial papaverine infusions for the treatment of cerebral vasospasm induced by aneurysmal subarachnoid hemorrhage. Neurocrit Care. 2005;2:124-132.
59 Smith WS, Dowd CF, Johnston SC, Ko NU, DeArmond SJ, Dillon WP, et al. Neurotoxicity of intra-arterial papaverine preserved with chlorobutanol used for the treatment of cerebral vasospasm after aneurysmal subarachnoid hemorrhage. Stroke. 2004;35:2518-2522.
60 Feng L, Fitzsimmons BF, Young WL, Berman MF, Lin E, Aagaard BD, et al. Intraarterially administered verapamil as adjunct therapy for cerebral vasospasm: safety and 2-year experience. AJNR Am J Neuroradiol. 2002;23:1284-1290.
61 Badjatia N, Topcuoglu MA, Pryor JC, Rabinov JD, Ogilvy CS, Carter BS, et al. Preliminary experience with intra-arterial nicardipine as a treatment for cerebral vasospasm. AJNR Am J Neuroradiol. 2004;25:819-826.
62 Biondi A, Ricciardi GK, Puybasset L, Abdennour L, Longo M, Chiras J, et al. Intra-arterial nimodipine for the treatment of symptomatic cerebral vasospasm after aneurysmal subarachnoid hemorrhage: preliminary results. AJNR Am J Neuroradiol. 2004;25:1067-1076.
63 Rabinstein AA, Friedman JA, Nichols DA, Pichelmann MA, McClelland RL, Manno EM, et al. Predictors of outcome after endovascular treatment of cerebral vasospasm. AJNR Am J Neuroradiol. 2004;25:1778-1782.
64 Weidauer S, Lanfermann H, Raabe A, Zanella F, Seifert V, Beck J. Impairment of cerebral perfusion and infarct patterns attributable to vasospasm after aneurysmal subarachnoid hemorrhage: a prospective MRI and DSA study. Stroke. 2007;38:1831-1836.
65 Weidauer S, Vatter H, Beck J, Raabe A, Lanfermann H, Seifert V, et al. Focal laminar cortical infarcts following aneurysmal subarachnoid haemorrhage. Neuroradiology. 2008;50:1-8.
66 Topcuoglu MA, Ogilvy CS, Carter BS, Buonanno FS, Koroshetz WJ, Singhal AB. Subarachnoid hemorrhage without evident cause on initial angiography studies: diagnostic yield of subsequent angiography and other neuroimaging tests. J Neurosurg. 2003;98:1235-1240.
67 Duong H, Melancon D, Tampieri D, Ethier R. The negative angiogram in subarachnoid haemorrhage. Neuroradiology. 1996;38:15-19.
68 Alen JF, Lagares A, Lobato RD, Gomez PA, Rivas JJ, Ramos A. Comparison between perimesencephalic nonaneurysmal subarachnoid hemorrhage and subarachnoid hemorrhage caused by posterior circulation aneurysms. J Neurosurg. 2003;98:529-535.
69 Schievink WI, Wijdicks EF. Origin of pretruncal nonaneurysmal subarachnoid hemorrhage: ruptured vein, perforating artery, or intramural hematoma? Mayo Clin Proc. 2000;75:1169-1173.
70 Rinkel GJ, Wijdicks EF, Hasan D, Kienstra GE, Franke CL, Hageman LM, et al. Outcome in patients with subarachnoid haemorrhage and negative angiography according to pattern of haemorrhage on computed tomography. Lancet. 1991;19(8773):964-968. 338
71 Schievink WI, Wijdicks EF, Spetzler RF. Diffuse vasospasm after pretruncal nonaneurysmal subarachnoid hemorrhage. AJNR Am J Neuroradiol. 2000;21:521-523.
72 Ishihara H, Kato S, Akimura T, Suehiro E, Oku T, Suzuki M. Angiogram-negative subarachnoid hemorrhage in the era of three dimensional rotational angiography. J Clin Neurosci. 2007;14:252-255.
73 Hashimoto H, Iida J, Hironaka Y, Okada M, Sakaki T. Use of spiral computerized tomography angiography in patients with subarachnoid hemorrhage in whom subtraction angiography did not reveal cerebral aneurysms. J Neurosurg. 2000;92:278-283.
74 Khan N, Schuknecht B, Yonekawa Y. Presentation and management of patients with initial negative 4-vessel cerebral angiography in subarachnoid hemorrhage. Acta Neurochir Suppl. 2002;82:71-81.
75 Little AS, Garrett M, Germain R, Farhataziz N, Albuquerque FC, McDougall CG, et al. Evaluation of patients with spontaneous subarachnoid hemorrhage and negative angiography. Neurosurgery. 2007;61:1139-1150.
76 Tatter SB, Crowell RM, Ogilvy CS. Aneurysmal and microaneurysmal “angiogram-negative” subarachnoid hemorrhage. Neurosurgery. 1995;37:48-55.
77 Given CA, Burdette JH, Elster AD, Williams DWIII. Pseudo-subarachnoid hemorrhage: a potential imaging pitfall associated with diffuse cerebral edema. AJNR Am J Neuroradiol. 2003;24:254-256.
78 Phan TG, Wijdicks EF, Worrell GA, Fulgham JR. False subarachnoid hemorrhage in anoxic encephalopathy with brain swelling. J Neuroimaging. 2000;10:236-238.
79 Schievink WI, Maya MM, Tourje J, Moser FG. Pseudo-subarachnoid hemorrhage: a CT-finding in spontaneous intracranial hypotension. Neurology. 2005;65:135-137.
80 Mattioli C, Beretta L, Gerevini S, Veglia F, Citerio G, Cormio M, et al. Traumatic subarachnoid hemorrhage on the computerized tomography scan obtained at admission: a multicenter assessment of the accuracy of diagnosis and the potential impact on patient outcome. J Neurosurg. 2003;98:37-42.
81 Salvarani C, Brown RDJr., Calamia KT, Christianson TJ, Weigand SD, Miller DV, et al. Primary central nervous system vasculitis: analysis of 101 patients. Ann Neurol. 2007;62:442-451.
82 Ducros A, Boukobza M, Porcher R, Sarov M, Valade D, Bousser MG. The clinical and radiological spectrum of reversible cerebral vasoconstriction syndrome. A prospective series of 67 patients. Brain. 2007;130:3091-3101.