Chapter 2
Spirometry
1. Determine whether spirometry is acceptable and repeatable.
2. Identify airway obstruction using vital capacity (VC) and forced expiratory volume in one second (FEV1).
3. Differentiate between obstruction and restriction as causes of reduced VC.
4. Determine whether there is a significant response to bronchodilators.
1. Select the appropriate VC and FEV1 for reporting from a series of spirometry maneuvers.
2. Quantify the degree of obstruction according to ATS-ERS criteria.
3. Describe the characteristics of an intrathoracic or extrathoracic obstruction pattern.
Vital capacity
Description
The VC is the volume of gas measured from a slow, complete expiration after a maximal inspiration, without forced or rapid effort (Figure 2-1). Alternately, VC may be recorded as a maximal inspiration following a complete expiration. VC is normally recorded in either liters (L) or milliliters (mL), and reported at body temperature, pressure, and saturation (BTPS). VC is sometimes referred to as the slow vital capacity (SVC), distinguishing it from FVC. Inspiratory capacity (IC) and expiratory reserve volume (ERV) are subdivisions of the VC. IC is the largest volume of gas that can be inspired from the resting expiratory level (see Figure 2-1). IC is sometimes further divided into the tidal volume (VT) and inspiratory reserve volume (IRV). ERV is the largest volume of gas that can be expired from the resting end-expiratory level (see Figure 2-1). Both the IC and ERV are recorded in liters or milliliters, corrected to BTPS.
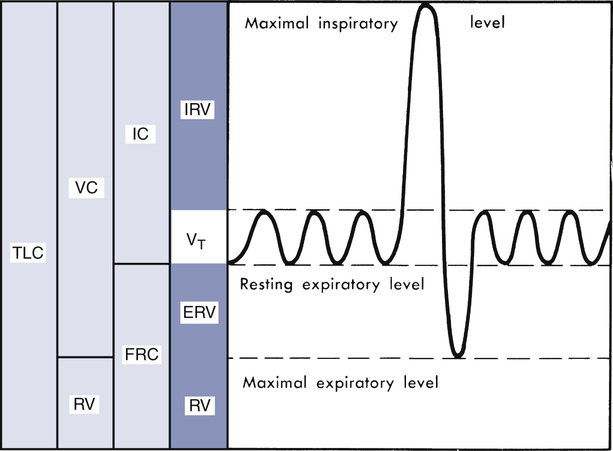
Diagrammatic representation of lung volumes and capacities based on a simple spirogram. TLC, total lung capacity; VC, vital capacity; RV, residual volume; FRC, functional residual capacity; IC, inspiratory capacity; Vt, tidal volume; IRV, inspiratory reserve volume; ERV, expiratory reserve volume. Relationships between the subdivisions and relative sizes as compared with TLC are shown (shaded areas). Resting expiratory level is used as a starting point for FRC determinations because it remains more stable than other identifiable points during repeated measurements. (Modified from Comroe JH Jr, Forster RE, Dubois AB, et al. The lung: Clinical physiology and pulmonary function tests. 2nd ed. St. Louis: 1962; Mosby.)
Technique
VC is measured by having the patient inspire maximally and then exhale completely into a spirometer. (See Chapter 11 for a complete discussion of spirometers.) The patient is instructed to perform the maneuver slowly and completely. VC can also be measured from maximal expiration to maximal inspiration. The spirometer does not need to produce a graphic display if only VC is to be measured. However, if IC and ERV are to be determined (see Figure 2-1), some means of recording volume change is required. The graphic display may be a computer screen or a recording device (see Chapter 11). A graphic display allows the technologist to determine that the test is performed correctly (Criteria for Acceptability 2-1). A graphic display is also usually required for reimbursement purposes.
Obtaining a valid slow VC is important. The subdivisions of the VC (IC and ERV) are used in the calculation of residual volume (RV) and total lung capacity (TLC). An excessively large tidal volume or an irregular breathing pattern during the VC maneuver may alter ERV or IC. If either ERV or IC is erroneously recorded, other lung volumes may be incorrectly estimated (see Chapter 4).
The accuracy of the IC and ERV measurements depends on the stability of the end-expiratory level, which should vary by less than 100 mL. Three or more tidal breaths should be recorded before the VC maneuver is performed. If the end-expiratory volume is not consistent, IC and/or ERV may be measured incorrectly (i.e., too large or too small). Even if the end-expiratory level is constant, the VT usually increases when the patient breathes through a mouthpiece with a noseclip in place. This increase in VT may change the IC or ERV, depending on the patient’s breathing pattern. Erroneous estimates of ERV may affect the calculation of RV, as described in Chapter 4.
Significance and Pathophysiology
Spirometry is indicated in a variety of situations (see Chapter 1). According to the 2005 ATS-ERS recommendations, there is only one contraindication to spirometry but several conditions that could yield a suboptimal test (Box 2-1). However, testing personnel should be aware of other conditions, which should be evaluated before testing, such as recent stroke, eye surgery, thoracic/abdominal surgery or pneumothorax, uncontrolled hypertension or hypotension,and known thoracic, aortic, or cerebral aneurysm.
Normal VC may vary as much as 20% above or below the predicted value in healthy individuals, but it must be above the bottom 5th percentile (lower limit of normal; see Chapter 13) to be considered normal. The ATS-ERS guidelines recommend the use of the National Health and Nutrition Examination Survey III (NHANES III) reference equations (see Evolve website http://evolve.elsevier.com/Mottram/Ruppel/) for patients in the United States ages 8-80 years. For children under 8 years old, the guidelines recommend the equations of Wang and others. However, in 2007, Stanojevic and others extended the NHANES III dataset to age 4 years.
In adults, VC varies directly with height and inversely with age; tall patients have larger VCs than short patients. VC increases up to approximately age 20 and then decreases each year thereafter, with an average decrease of about 25-30 mL/yr. It is usually smaller in women than in men because of differences in body size. Recent evidence indicates that lung volumes may differ significantly according to ethnic origin. VC also varies in individuals, depending on body position or time of day. Interpretation of lung function should consider the key factors of age, sex, height, and race (see Chapter 13).
As Hutchinson originally noted (see Chapter 1), a patient’s vital capacity is directly related to survival, and numerous studies have since supported his conclusion. In a study published in 2011 using data on spirometry and survival from the Atherosclerosis Risk in Communities (ARIC) dataset, 7489 participants with usable spirometry data were compared against measures of ventilatory function after controlling for many other factors likely to be associated with survival. The study concluded that survival was strongly associated with the forced vital capacity over all other spirometry parameters.
There are numerous causes of a decreased VC (Box 2-2). In general, these fall into the categories of loss of distensible lung tissue (i.e., fibrosis), obstructive lung disease, and reduced chest wall expansion (i.e., neuromuscular, kyphosis).
When the VC is reduced, additional pulmonary function measurements may be indicated. Forced expiratory maneuvers (see section, Forced Vital Capacity) can reveal whether the reduced VC is caused by obstruction. Reduced VC without slowing of expiratory flow is a nonspecific finding. Measurement of other lung volumes (see Chapter 4) may be indicated to determine whether a restrictive defect is present. Measurement of muscle pressures may help determine whether there is a problem with neuromuscular weakness (see Chapter 10).
In adults, VC less than the lower limit of normal (95% confidence limits, see Chapter 13) may be considered abnormal. Interpretation of the measured VC in relation to the reference value should consider the clinical question to be answered (Interpretive Strategies 2-1). The clinical question is often revealed in the history and physical findings of the patient (see Chapter 1). The terms mild, moderate, and severe may be used to qualify the extent of reduction of the VC in a manner similar to that described for FEV1 (see following section, FEV1).
Artificially low estimates of the VC may result from poor patient effort. Similarly, inadequate patient instruction may affect the performance of the test maneuver. These errors may be eliminated by applying appropriate criteria (Criteria for Acceptability 2-1). Values for at least two maneuvers should be reproducible within 150 mL (see Chapter 12).
IC and ERV are approximately 75% and 25% of the VC, respectively. Changes in IC or ERV usually parallel increases or decreases in the VC. Increased VT caused by exertion or acid-base disorders (e.g., metabolic acidosis, respiratory alkalosis) may reduce IRV or ERV. This occurs because end-inspiratory and end-expiratory levels (see Figure 2-1) are altered. A similar pattern is commonly seen when patients breathe into a spirometer through a mouthpiece with noseclips in place. Changes in IC or ERV are of minimal diagnostic significance when considered alone. Reduction of IC or ERV is consistent with restrictive defects. Obese patients typically show a decrease in ERV, usually resulting in a low VC. A reduced ERV is one of the earliest lung function changes in obese patients.
Forced Vital Capacity, Forced Expiratory Volume, and Forced Expiratory Flow
Description
Technique
FVC is measured by having the patient, after inspiring maximally, expire as forcefully and rapidly as possible into a spirometer (see Chapter 1). The patient should inspire completely. The inhalation should be rapid but not forced. There should be little, if any, pause (less than 1-2 seconds) at maximal inspiration; a prolonged pause (4-6 seconds) may decrease flow during the subsequent expiration.
The volume expired may be read directly from a volume-time recording (Figure 2-2). This method is used by some small portable spirometers. More commonly, the maneuver is displayed on a computer monitor or liquid crystal display screen. The computer analyzes the signal from the spirometer, then calculates and displays the FVC. A spirometer that produces a graphical tracing (either volume-time or flow-volume) is essential for clinical
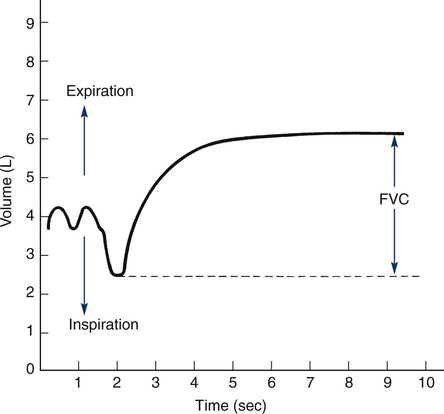
Typical spirogram plotting volume against time as patient exhales forcefully. In this tracing, expiration causes an upward deflection; in some systems, the tracing is inverted. The patient inspires to the maximal inspiratory level (dashed line), at which point lung volume is close to TLC. The patient then expires as forcefully and rapidly as possible to the maximal expiratory level, at which point the lungs contain the RV only (see text).
laboratory purposes to allow a visual inspection of the maneuver (Figure 2-3) and is also necessary for reimbursement purposes. Devices providing only numerical data may be helpful for simple screening. Whether used for diagnosis or monitoring, all spirometers should meet the criteria proposed by the ATS-ERS (see Chapter 12). The FVC maneuver depends on patient effort. Not all patients may be able to perform it acceptably (Criteria for Acceptability 2-2).
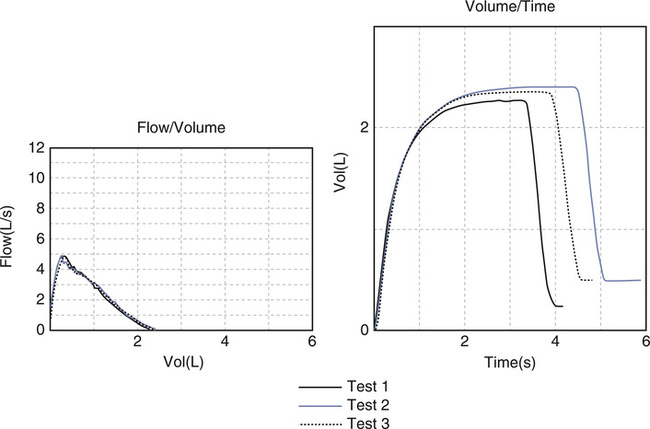
Acceptability based on a fast start (no hesitation), a sharp peak flow, no cough or glottic closure in the first second, and meeting the end of test criteria.
FEV1 (and other FEVT values) may be measured by timing the FVC maneuver over the described intervals. Historically, this was done by recording the FVC spirogram on graph paper moving at a fixed speed. The FEV for any interval could then be read from the graph, as shown in Figure 2-4. Most modern spirometers time the FVC maneuver using a computer. The computer then calculates and displays the FEV1 or other FEVT intervals. The spirometer should provide a volume-time display of each maneuver (Figure 2-5). A graphic representation allows the monitoring of patient effort at the beginning of the test. Accurate measurement of FEV1 (and other FEVT intervals) depends on the determination of the start-of-test (Figure 2-6). Computerized spirometers detect the start-of-test as a change in flow or volume above a certain threshold. The computer then stores volume and flow data points in memory and calculates the FEV1.
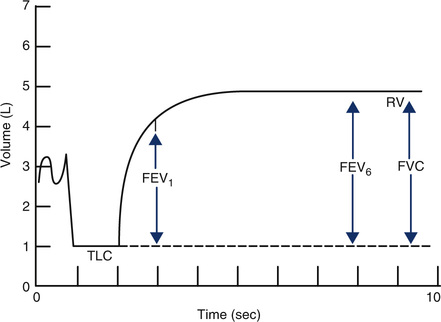
Various FEVT values can be measured from the volume-time display of an FVC effort. FEV at intervals of 1 and 6 seconds, along with the FVC, are shown (arrows). FEV1 is the most commonly used index of airflow. FEV6 is sometimes used as a surrogate for FVC in patients with airway obstruction. Precise timing and acceptable start-of-test are required to determine FEVT values accurately (Criteria for Acceptability 2-2).
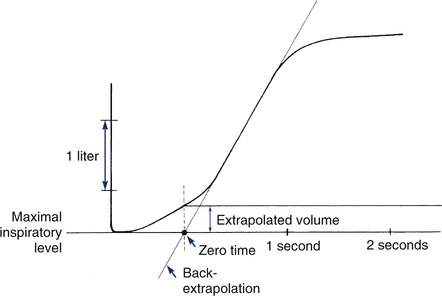
Back-extrapolation is a method for correcting measurements made from a spirogram that does not show a sharp deflection from the maximal inspiratory level. This occurs when a patient does not begin forced exhalation rapidly enough or hesitates at the start-of-test. A straight line drawn through the steepest part of a volume-time tracing is extended to cross the volume baseline (maximum inspiration). The point of intersection is the back-extrapolated time zero. To accurately determine the new time zero, the display should include at least 0.25 seconds and preferably at least 1 second before the start of the exhalation. Timed volumes, such as FEV1, are measured from this point rather than from the initial deflection from the baseline or from the point of maximal flow. The perpendicular distance from maximal inspiration to the volume-time tracing at time zero defines the back-extrapolated volume. To accurately determine FEV1, the back-extrapolated volume should be less than 5% of the FVC or less than 150 mL, whichever is greater. FVC efforts with larger extrapolated volumes should be considered unacceptable.
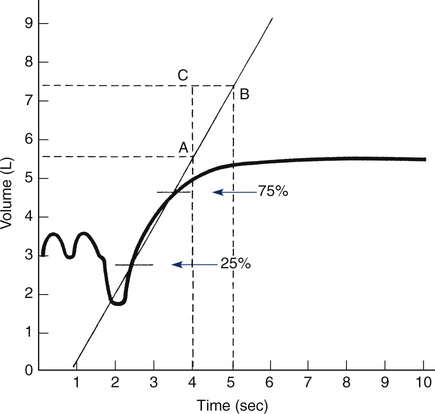
The slope of the line connecting 25% and 75% points is the average flow over the middle half of the FVC maneuver. Alternatively, a line connecting these points may be drawn to intersect two timelines 1 second apart (points A and B). The flow in liters per second can be read as the vertical distance between the points of intersection (AC)—in this case, approximately 2 L/sec.
Open circuit technique describes an FVC maneuver where the subject inhales maximally, places the mouthpiece or filter into their mouth, and then forcefully exhales until empty. Using this technique, the patient has to coordinate taking the deep breath, holding the breath at TLC, and placing the mouthpiece correctly, which can be a challenge for some subjects. Closed-circuit technique describes a maneuver where the subject breathes tidally on the spirometer, inhales maximally, forcefully exhales, and then may be instructed to inhale forcefully to measure the inspiratory flow and volume. Most computerized spirometers correct for a slow start-of-test by back-extrapolation (see Figure 2-5). Visualization of the volume-time spirogram is the best means of identifying poor initial effort. Some portable spirometers report FEV1 without a spirogram. Such measurements should be used with caution because it may be difficult to determine whether the maneuver was performed acceptably.
The ratio of the FEV1 to FVC is expressed as follows:

The FEF25%-75% is measured from an FVC maneuver. The FEF25%-75% is the average flow during the middle half (from 25% to 75%) of the VC. A computerized measurement of the FEF25%-75% requires storage of flow and volume data points for the entire maneuver. Calculation of the average flow over the middle portion of the exhalation is simply 50% of the volume expired divided by the time required to get from the 25% point to the 75% point. To manually calculate the FEF25%-75%, a volume-time spirogram is used. The points at which 25% and 75% of the vital capacity have been expired are marked on the curve (see Figure 2-6). A straight line connecting these points can be extended to intersect two timelines 1 second apart. The flow (in liters per second) can then be read directly as the vertical distance between the points of intersection. Instantaneous flows, such as the FEF50% or FEF75%, cannot be read directly from a volume-time display but can be measured using a flow-volume curve (see later section, Flow-Volume Curve).
The FEF25%-75% depends on the FVC. Large FEF25%-75% values may be derived from maneuvers that produce small FVC measurements because the “middle half” of the volume is actually gas expired at the beginning of expiration. This effect may be particularly evident if the patient terminates the FVC maneuver before exhaling completely. When the FEF25%-75% is used for assessing the response to bronchodilator or bronchial challenge, the effect of changes in the absolute lung volumes must be considered. Measuring the FEF25%-75% at the same lung volumes in the comparison tests is called the isovolume technique. Isovolume corrections are usually applied when the FVC changes by more than 10% (indicating a change in TLC or RV). This technique requires that lung volumes (see Chapter 4) be measured in conjunction with flows. The isovolume technique may also be used with other flow measurements that are dependent on FVC.
Acceptability and Repeatability for Spirometry Results
1. The volume-time tracing should show maximal effort with a smooth curve. There should be no coughing or hesitation during the first second. The tracing should show at least 6 seconds of forced effort. An obvious plateau with no volume change (25 mL or less) for at least 1 second should be achieved. Children, adolescents, and some restricted patients may plateau in less than 6 seconds (children less than 10 years of age must exhale at least 3 seconds). Patients with severe obstruction may continue exhalation well past 15 seconds; therefore, 6 seconds is simply a minimum. In severe obstruction, very low flows may be observed at the end of expiration. Continuation of the maneuver in these patients will not appreciably change the test results. The FVC maneuver may be stopped if the patient cannot continue for clinical reasons such as excessive coughing or dizziness. Multiple prolonged (longer than 6-15 seconds) exhalations are seldom necessary.
2. The start-of-test should be abrupt and unhesitating. Each maneuver should have the back-extrapolated volume calculated. FEV1 and all other flows must be measured after back-extrapolation (see Figure 2-5). The ATS-ERS guidelines recommend that the volume-time tracing must begin at least 0.25 seconds before the beginning of the exhalation in order to be able to measure the back-extrapolated volume. If the volume of back-extrapolation is greater than 5% of the FVC or 150 mL (whichever is greater), the maneuver is unacceptable and should be repeated. The patient should be shown the correct technique for performing the maneuver. Demonstration by the technologist is often helpful.
3. A minimum of three acceptable efforts should be obtained. The test may be repeated multiple times, but if repeatable values cannot be obtained after eight attempts, testing may be discontinued. The only criterion for eliminating a test session completely is failure to obtain two acceptable maneuvers after at least eight attempts.
4. The two largest values for both FVC and FEV1 should be within 150 mL (or within 100 mL if the FVC is 1 liter or less). The second largest value is simply subtracted from the largest value for both FVC and FEV1 to determine repeatability. Some clinicians prefer to use 5% as the repeatability criterion. This may be more appropriate than an absolute volume of 150 mL, particularly in children or those with large FVC values. However, the 150 mL of absolute volume criterion has less variance than the 5% criterion, making it less dependent on individual characteristics and therefore more widely applicable to patients of varying age, gender, and baseline lung function. If the two largest FVC or FEV1 values are not within 150 mL (or 5%, if that criterion is applied), the maneuver should be repeated up to a maximum of eight times or until the patient cannot or will not continue. The repeatability criteria should be applied only after the maneuver has been judged as acceptable. Individual spirometric maneuvers should not be rejected solely because they are not repeatable. Bronchospasm or fatigue often affects repeatability. Interpretation of the test should include comments regarding repeatability or lack of it. As a minimum, three acceptable satisfactory maneuvers should be saved for evaluation. (See Figure 2-6.)
Data from all acceptable maneuvers should be examined. The largest FVC and the largest FEV1 should be reported, even if the two values are from different test maneuvers. The reported FEV1/FVC ratio is taken from these values. Flows that depend on the FVC (e.g., the FEF25%-75%) should be taken from the single best test maneuver, which is the maneuver with the largest sum of FVC and FEV1 (Table 2-1). The reported flow-volume curve is also taken from the single best test maneuver.
Table 2-1
Comparison of Spirometry Efforts
Test | Trial 1 | Trial 2 | Trial 3 | Best Test Reported |
FVC (L) | 5.20 | 5.30 | 5.35* | 5.35 |
FEV1 (L) | 4.41* | 4.35 | 4.36* | 4.41 |
FEV1/FVC (%) | 85 | 82 | 82 | 82 |
FEF25%-75% (L/sec) | 3.87 | 3.92 | 3.94 | 3.94 |
FEF50% (L/sec) | 3.99 | 3.95 | 3.41 | 3.41 |
FEF25% (L/sec) | 1.97 | 1.95 | 1.89 | 1.89 |
PEF (L/sec) | 8.39 | 9.44 | 9.89 | 9.89 |
*These values are keys to selecting the best test results. The FEV1 is taken from trial 1 and the FVC from trial 3, even though the largest sum of FVC and FEV1 occurs in trial 3. All FVC-dependent flows (average and instantaneous) come from trial 3. The maximal expiratory flow-volume curve, if reported, would be the curve from trial 3 as well. It should be noted that the FEV1% (FEV1/FVC) is calculated from the FEV1 of trial 1 and the FVC of trial 3.
Significance and Pathophysiology
Forced Vital Capacity
See Interpretive Strategies 2-2. FVC usually equals VC in healthy individuals. In patients without obstruction, FVC and VC should be within 150 mL of each other. FVC and VC may differ if the patient’s effort is variable or if significant airway obstruction is present (i.e., FEV1/FVC less than 0.70). FVC is often lower than VC in patients with obstructive diseases if forced expiration causes airway collapse. This pattern is often seen in emphysema because of a loss of tethering support of the airways. Large pressure gradients across the walls of the airways during forced expiration collapse the terminal portions of the airways. Gas is trapped in the alveoli and cannot be expired. This causes the FVC to appear smaller than the VC. The FVC can appear larger than the VC if the patient exerts greater effort on the forced maneuver.
FVC can be reduced by mucus plugging and bronchiolar narrowing, as is common in chronic
bronchitis, chronic or acute asthma, bronchiectasis, and cystic fibrosis. Reduced FVC is also present in patients whose trachea or mainstem bronchi are obstructed. Tumors or diseases affecting the patency of the large airways can produce this result.
Some obstructed patients have a relatively normal FVC in relation to their predicted values. However, the time required to expire their FVC (forced expiratory time or FET) is usually prolonged. Healthy adults can expire their FVC within 4 to 6 seconds. Normal children and adolescents may exhale their FVC in less than 4 seconds. Patients with severe obstruction (e.g., those with emphysema) may require 15 seconds or more to exhale completely (Figure 2-7). Accurate measurement of FVC in such individuals may be limited by how long the spirometer can collect exhaled volume. Some spirometers allow only 15 seconds of volume recording. This is usually long enough to diagnose airway obstruction. However, the FVC and FEV1% may be inaccurate if the patient continues to exhale for a longer time. The ATS-ERS recommends that spirometers measure FVC for at least 15 seconds (see Chapter 12).
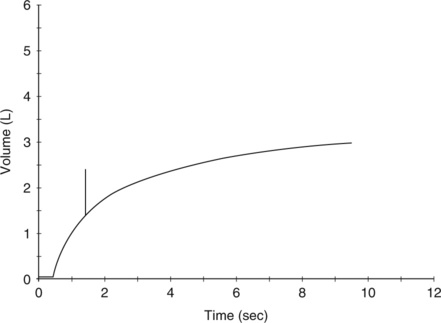
A tick mark notes the point at which the patient exhaled his FEV1. A significant volume is exhaled after the first second, and the tracing does not show an obvious plateau.
An alternative to measuring FVC in severely obstructed patients is to use FEV6. Because normal patients can exhale their FVC in 6 seconds, substituting FEV6 for FVC allows the FEV1/FEV6 to be used as an index of obstruction. Using FEV6 in place of FVC eliminates the necessity of having the patient try to exhale for a long interval. Predicted values for FEV6 and FEV1/FEV6 may be calculated using coefficients in Chapter 13. Because FEV6 may underestimate FVC, use of FEV1/FEV6 instead of FEV1/FVC may reduce the sensitivity of spirometry to detect airway obstruction, especially in older patients and in those with mild obstruction.
Reduced FVC (or VC) is a nonspecific finding. Values below the 5th percentile are considered abnormal (see Chapter 13). A low FVC may be caused by either obstruction or restriction (see Box 2-2). Interpretation of the FVC in obstructive diseases requires correlation with flows. An FVC that is significantly lower than VC suggests airway collapse and gas trapping. In restrictive patterns, low FVC may indicate the need to assess other lung volumes, particularly total lung capacity (TLC; see Chapter 4). Interpretation of FVC values close to the lower limit of normal depends on the clinical question to be answered. An FVC at the 5th percentile would be interpreted differently in a healthy patient with no symptoms than in a patient with a history of cough or wheezing. An FVC much lower than expected is often accompanied by the complaint of exertional dyspnea.
Forced Expiratory Volume (FEV1)
FEV1 measures the volume expired over the first second of an FVC maneuver. FEV1 is reported as a volume, although it measures flow over a specific interval. FEV1, like FVC, may be reduced in either obstructive or restrictive patterns (Figures 2-7 and 2-8). FEV1 values may also be reduced because of poor effort or cooperation by the patient.
ModerateFEV1 = 60%-69% predicted
Moderately severeFEV1 = 50%-59% predicted