8 Spinal Disorders
Whiplash Injury: Treatment and Rehabilitation
Adriaan Louw, PT, MAppSc (Physio), CSMT
The Whiplash Epidemic
In 1928 Harold Crowe introduced the term “whiplash” to describe an injury mechanism of sudden hyperextension followed by hyperflexion of the neck. Although several other terms (e.g., necklash, hyperextension injury, and acceleration injury) have been suggested, the term whiplash has stood the test of time. Unfortunately, the term “whiplash,” which was used originally to graphically describe the manner in which the head was suddenly moved, has become a commonly used diagnostic label (Bogduk 2003). The biggest criticism associated with the labeling is the lack of information regarding the diagnosis, injury, prognosis, or treatment. Whiplash can best be described as a sudden acceleration and deceleration of the head in space (Fig. 8-1) (Bogduk 2003 and Spitzer et al. 1995). This describes the process of the sudden movement and slowing down of the head in space that can occur during a motor vehicle collision (MVC), sport, or activity of daily living (ADL) (Spitzer et al. 1995). Patients presenting for the treatment of signs and symptoms associated with the whiplash injury are said to have a whiplash-associated disorder (WAD) (Spitzer et al. 1995).
Whiplash injuries have been called the “disease of the century” with ever-increasing numbers of people diagnosed with WAD and seeking treatment (Bogduk 2003). Motor vehicle collisions are the leading cause of death among Americans 1 to 34 years old, and according to the U.S. Department of Transportation, the total societal cost of crashes exceeds $200 billion annually. With the increasing number of patients with WAD; little information available regarding the epidemiology; and nothing written on diagnosis, prognosis, and various interpretations of the treatments, therapists often describe whiplash as one of the most challenging and frustrating conditions to treat (Holm et al. 2007 and Spitzer et al. 1995). Adding to the frustration, several studies have shown that WAD leads to high rates of chronic pain and disability (Holm et al. 2007 and Sterling et al. 2006). It is now accepted that approximately one in four or even as many as one in three patients may develop pain lasting more than 2 years after an MVC (Bogduk 2003 and Spitzer et al. 1995). It is also interesting to note that no management approach treating acute whiplash has substantially reduced the incidence of transition to chronicity of this disorder (Spitzer et al. 1995).
Diagnosis
Before any attempt is made to treat WAD, consideration must be given to diagnosing the patient. Several authors have described this as a pivotal part in the development of persistent pain in patients with whiplash (Bogduk 2003 and Spitzer et al. 1995). Numerous studies have shown the high incidence of missed injuries, including fractures on standard imaging studies such as x-ray, magnetic resonance imaging (MRI), and computerized tomography (CT) (Bogduk 2003 and Spitzer et al. 1995). Because of the poor ability of these studies to truly identify tissue pathology, current Emergency Department (ED) guidelines do not routinely prescribe the use of imaging techniques such as x-ray, MRI, or CT scan. This creates a problem: A patient has significant pain and dysfunction, yet imaging tests are unable to “find a cause” of the pain. This has, unfortunately, also caused patients with WAD to be viewed as malingerers, dishonest, or even neurotic (Spitzer et al. 1995 and Sterling et al. 2006). Several studies have shown that a valid way to determine disability following whiplash is the taking of a thorough history and evaluation of the patient’s disability (Carroll et al. 2009 and Spitzer 1995). In fact, the neck disability index (NDI), which was originally designed for mechanical neck pain, was validated for use in patients with WAD. A high NDI indicates significant disability as a result of WAD, regardless of the results of the imaging tests. Therapists should realize that a thorough history and questions regarding function and/or the use of the NDI are currently the preferred tests to evaluate the effect of the WAD on the patient.
A second common fault in dealing with whiplash injuries is that patients with mechanical neck pain and traumatic neck pain (whiplash) often are grouped together (Jull et al. 2007). It is clear from the research that this is not only wrong, but also poses significant problems for patients and health care providers. The current research into whiplash injuries clearly shows that whiplash trauma is not biomechanically compatible with ADLs. Additionally, whiplash is not a homogeneous entity, with evidence from research indicating that WAD presents as a heterogeneous complaint in terms of the levels of pain and disability and changes in the sensory, motor, and psychological systems (Jull et al. 2007); however, such classification does little to direct physical therapy management. It is recommended therapists utilize two models: idiopathic neck pain and neck pain following trauma. Current research will most likely over time identify subclassifications of the WAD group (Fig. 8-2).
Treatment: Review of the Literature
In 1928 the term “whiplash” was introduced by Harold Crowe at a scientific meeting; for treatment of whiplash he suggested, “the less the treatment, the better it is.” In 1954 Gray and Abott advised bed rest during the first 24 to 72 hours and sedation as part of the medical approach and concluded that “constant use of a cervical collar resulted in atrophy and worsening of symptoms.” The first studies on exercise emerged in the 1960s, in which authors such as Barufaldi 1961) recommended traction and isometric exercises, followed by relaxation exercises. The first major study was published by Janes and Hooshmand (1965), who investigated 10,000 patients with whiplash between 1956 and 1963. They showed the following:
In the mid 1970s the controversies surrounding mobilization and manipulation started when authors began describing the benefits and precautions in applying mobilization and/or manipulation. When reviewing the literature on the use of cervical spine soft collars, it is interesting to note a variance between minimum periods of 2 weeks up to 1 year after MVC. Numerous early studies recommended the immediate start of therapeutic traction, with or without the application of heat. Clinical trials soon followed (1989). In 1986 Mealy et al. compared soft collars to Maitland mobilization techniques and showed greater improvement in the mobilized group in terms of range of motion (ROM) and pain ratings. In 1989 McKinney et al. compared rest, active physiotherapy, and self-care, showing no significant difference, and in 1990 Pennie and Agambar compared cervical traction, self-care, and neck and shoulder exercises, again showing no significant difference.
In 1995 a landmark study on whiplash was published—“The Quebec Task Force Study. (Spitzer et al. 1995).” The Task Force reviewed 10,382 articles published on whiplash over a 10-year period and found 62 studies relevant and meritorious. The Task Force summarized the results of the most commonly used treatments for whiplash (Table 8-1). This review, although dated, is still seen by many as the gold standard and is used as a template for treatment. The Task Force concluded that:
Table 8-1 Summary of the Quebec Task Force Findings on the Treatment of Whiplash-Associated Disorders (WAD)
Treatment | Summary and Recommendation by the Task Force |
---|---|
Collars | Commonly prescribed; may delay recovery, causing increased pain, and decrease range of motion (ROM). Soft collars do not adequately immobilize the spine. |
Rest | Commonly prescribed for the first few days. Should be limited to less than 4 days. Detrimental to recovery from WAD. |
Cervical pillows | No studies |
Manipulation | Single manipulation reduced asymmetry but showed the results lasted less than 48 hours. Comparing mobilization to manipulation, no clear benefit of one over the other in decreasing pain and increasing ROM. Long-term manipulation is not justified in the treatment of WAD. |
Mobilization | Several studies. Maitland and McKenzie mobilization versus rest showed significantly greater improvement in pain and ROM for the mobilization groups. One study showed that patients given active exercises and advice recovered just as well as the mobilized group. Another study showed that mobilization was more effective than a combination of analgesics and education in the decrease of pain and increase of ROM. Mobilization appears to be beneficial in the short term, but the long-term benefits need to be established. Physical therapy should emphasize early return to usual activity and promote mobility. |
Exercise | No independent effect of exercise has been evaluated. The evidence suggests exercise as part of a multimodal intervention may be beneficial (short- and long-term). |
Traction | No independent effects of traction were found. One study tested different types of traction (static, intermittent, manual), but no significant differences were found on the different traction types. |
Posture | No studies |
Spray and stretch | No studies |
Transcutaneous electrical stimulation (TENS) | No accepted studies |
Electrical stimulation | No studies |
Ultrasound | No studies |
Laser, diathermy, heat, ice, massage | No independent studies. The modalities were part of the combination of passive modalities in different studies. |
Surgery | No studies on surgery or nerve blocks |
Injections Epidural Intrathecal Intra-articular |
No studies. Not justified in the management of WAD patients. |
Pharmacology Analgesics Anti-inflammatories |
Shown to be effective with the use of physical modalities. |
Muscle relaxants | No studies |
Psychosocial | No studies |
Acupuncture | No studies |
Treatment of Acute Whiplash (0–3 Weeks)
The first 3 weeks are chosen as the acute phase based on tissue healing time, clinical observation regarding referral to physical therapy, and important research related to timelines associated with whiplash (Sterling et al. 2003). This phase includes the immediate postwhiplash examination and treatment in the ED. After the ED visit patients typically are referred to their primary care physician for followup. Based on clinical observation, it is typical that injury, ED visit, physician followup, and subsequent referral to physical therapy occur around the third week. Based on the current best evidence into WAD treatment, some therapists may view this as inadequate and believe that therapy should start sooner. From a pain science perspective, it may be worthwhile to have a patient “rest” for a few days and psychologically “cope with the injury.” However, significant evidence suggests that early education and encouragement to move soon after the injury are of great value. This is different from aggressive early mobilization/manipulation. Several authors have questioned this approach because of the significant injuries associated with whiplash and the high incidence of missed fractures (Bogduk 2003). Two recent RCTs showed that patients who received educational videos in the ED regarding the pathology, self-help ideas, prognosis, plan of care, and goals performed much better than patients receiving “usual care”—ED waiting room, tests, medicine, and referral to their primary care physicians (Oliveira et al. 2006). Therefore, in the acute phase (0–3 weeks) therapists may consider the following:
Treatment of Subacute Whiplash (3 weeks–3 months)
Most patients seen in physical therapy after a whiplash injury are seen in the subacute phase. These patients follow the traditional model of injury: ED visit, referral to their primary care physician, and then referral to physical therapy approximately 3 weeks after the injury. These patients usually present with neck pain, scapular pain, headaches, decreased ROM, decreased function, and possible neurologic deficit (Bogduk 2003 and Spitzer et al. 1995). Treatment of the patient with subacute whiplash includes all of the acute whiplash principles and current best-evidence strategies.
Spinal stabilization exercises (Sterling et al. 2003). There is a large body of research regarding segmental spinal stabilization in the lumbar spine. Similar research is now emerging about the cervical spine (Jull et al. 2007 and Sterling et al. 2003). The cervical spine helps support and orient the head in relation to the thoracic spine and thus in essence provides two key elements: stability and mobility. Similar to the lumbar spine, the deeper muscles closer to the spine contribute to stability of the cervical spine, whereas the larger, superficial muscles that span multiple joints contribute more to movement. The deeper muscles have control strategies and proper morphology to stabilize the neck. In healthy individuals, the deep neck flexors provide a low-level, tonic contraction prior to movement of the extremities to protect the cervical spine. However, following injury, several changes occur to the deep cervical flexors:
All of these changes indicate that patients with neck pain (i.e., WAD) will demonstrate limited endurance, greater fatigability, less strength, altered proprioception, and reorganization of motor control. The cranio-cervical flexion test (CCFT) is used to evaluate the ability of the deep cervical flexors to produce low-load tonic submaximal contractions (Fig. 8-3) (Jull et al. 2008). Therapists should concentrate part of the rehabilitation of the patient with whiplash to retraining the deep neck flexors of the cervical spine. These exercises should aim to provide protection to the cervical spine during ADLs. Once local stabilizers have been activated and retrained, therapists should have patients perform various forms of exercises incorporating weights and resistive bands while engaging the deep neck flexors. Stabilization exercises should aim to focus on low-load, tonic contractions, which should be progressed by increasing the time the patient contracts the deep neck flexors—endurance.
Manual Therapy (Spitzer et al. 1995). The Quebec Task Force study and subsequent systematic reviews and RCTs have shown that manual therapy techniques such as spinal mobilization and spinal manipulation may be of benefit to patients with subacute whiplash. Following a thorough examination, therapists are encouraged to carefully progress through the grades of movement/resistance to alleviate pain and dysfunction associated with specific physical dysfunction of specific joints/spinal levels. Manual therapy can be applied to both the cervical spine and thoracic spine. Evidence suggests that patients with neck pain respond favorably to manipulative therapy applied to the thoracic spine. Considering the concerns about missed fractures and significant tissue injury with whiplash, it is recommended that therapists perform skilled evaluations, continually reassess the patient, and adhere to a principle taught by manual therapy pioneer Geoffrey Maitland (2005) of “using the least amount of force to gain the desired outcome.” Qualitative studies have shown that patients want to receive hands-on treatment, and studies that compared hands-on to exercise-only interventions have found that hands-on treatment leads to better outcomes in the short term.
Consideration also should be given to strategies aimed at reducing the chance of a patient with subacute whiplash developing chronic WAD. Therapists who treat patients in the acute and subacute phases have a unique opportunity to help a patient progress through these phases and not move on to the chronic phase. Several factors have been identified in the development of chronicity, including factors associated with the accident (head position, signs and symptoms, the accident site, male/female, stationary car, etc.) and patient attributes (poor coping skills, extended rest, etc.) (Bogduk 2003, Jull et al. 2008, and Spitzer et al. 1995). Some factors can be positively affected by a therapist in the acute and subacute phases.
Treating Chronic Whiplash (3 Months and More)
Although patients with subacute whiplash may be the group most frequently presenting to physical therapy, patients with chronic whiplash are the most challenging. Three months is chosen specifically for the chronic phase because several studies have shown that patients who do not have significant decrease in their symptoms by 3 months postinjury have a very high likelihood of developing chronic pain associated with the whiplash injury (Spitzer et al. 1995, Sterling et al. 2003, and Sterling et al. 2006). This population also includes patients with whiplash who show up months or even years after injury seeking help for the pain and disability.
Education has been discussed in detail. Recent research has evaluated the use of neuroscience education in decreasing pain and disability among patients with chronic pain. It is recommended that therapists educate patients more regarding their pain as opposed to only using anatomy models. Additionally, neuroscience education has been shown to decrease fear and change a patient’s perception of his or her pain (Oliveira et al. 2006).
Therapeutic Exercise for the Cervical Spine
Christopher J. Durall, PT, DPT, MS, SCS, LAT, CSCS
Neck pain affects most adults at some point in their lives, and nearly 20% of the population suffers from persistent or recurrent symptoms (Croft et al. 2001, Binder 2006). Individuals with neck pain may have deficits in coordination (Falla et al. 2004a, Chui et al. 2005), strength, endurance (O’Leary et al. 2007c), repositioning acuity (Kristjansson et al. 2003, Sjolander et al. 2008), postural stability (Michaelson et al. 2003), or oculomotor control (Treleaven et al. 2005a). Patients with neck pain may also have mobility deficits in the cervical and/or upper thoracic regions (Childs et al. 2008). Therapeutic exercise has shown considerable promise as an intervention for individuals with neck pain (Kay et al. 2005, Gross et al. 2007), despite a lack of consensus among clinicians and researchers on optimal exercises or guidelines. In this section, exercises intended to correct deficits are discussed, with the objectives of reducing symptoms, improving function, and preventing recurrence.
Exercises to Improve Muscular Coordination, Endurance, or Strength
Deficits in cervical muscle performance may occur rapidly following the onset of neck pain and may persist despite symptom reduction or resolution (Sterling et al. 2003). Research has shown that exercises to improve coordination, endurance, or strength can aid neck symptom resolution (Sarig-Bahat 2003). This is logical given that the neck musculature provides nearly 80% of the mechanical stability of the cervical spine (Panjabi et al. 1998).
The deep cervical flexor (DCF) muscles (longus capitus and colli, rectus capitus anterior and lateralis, hyoid muscles) and deep cervical extensor (DCE) muscles (semispinalis cervicis, multifidus, rectus capitus posterior major and minor), in particular, appear prone to impairment in patients with neck pain (Sterling et al. 2003). These muscles have a high density of type I fibers and muscle spindles and are vulnerable to pain inhibition (Boyd-Clark et al. 2002). Reduced control and capacity of the deeper neck muscles can result in unwanted segmental motion or buckling during contraction of the multisegmental superficial muscles (Winters & Peles 1990). Thus the initial rehabilitation emphasis should be toward improving performance or coordination of the deeper cervical muscles.
Exercises to Improve Muscular Coordination
Patients with neck pain tend to have impaired DCF activity and elevated superficial cervical flexor (SCF; sternocleidomastoid [SCM], anterior scalene) activity during craniocervical flexion (Falla et al. 2004a, Chui et al. 2005). One exercise reported to help reverse this aberrant neck flexor synergy uses a pressure device positioned inferior to the occiput to provide feedback (Fig. 8-4). For this exercise the patient attempts to flatten the cervical lordosis, which requires DCF contraction (Mayoux-Benhamou et al. 1994), while minimizing SCF activation. The contractile effort with this exercise should be low and the patient should focus on precise control of the movement. Low-load exercises (∼20% maximal voluntary contraction) have been shown to facilitate more selective activation of the deeper cervical flexor and extensor muscles, while minimizing activity in their more superficial synergists (O’Leary et al. 2007b). Gentle, low-load exercise has also been shown to produce a superior, immediate hypoalgesic effect relative to higher-load exercise and is more appropriate when pain is a primary concern. Exercising above the pain threshold can impair neuromuscular control (Falla et al. 2007).
The pressure device–assisted craniocervical flexion exercise was reported to be as effective at increasing cervical flexion strength as an endurance exercise program in patients with chronic neck pain (Falla et al. 2006). Moreover, the perception that the exercise program was beneficial was roughly 10% greater in the group that performed craniocervical flexion with a pressure device. Of interest, this exercise was shown to improve repositioning acuity in people with neck pain to nearly the same extent as a proprioceptive training regimen (Jull et al. 2007b).
Controlled craniocervical flexion also can be done without a pressure device (Fig. 8-5). This exercise can be done sitting or standing initially to minimize gravity resistance and then reclined as tolerated to increase gravity resistance. Once the patient can nod while supine with minimal SCF activation, he or she can practice flexing the lower cervical spine while sustaining upper cervical flexion (Fig. 8-6). The SCMs are required to flex the lower cervical segments, so the patient does not need to palpate the SCMs during the combined movement. Inability to sustain upper cervical flexion during this exercise results in head protrusion (Fig. 8-7), which indicates that the exercise is too challenging and should be regressed. Krout and Anderson (1966) reported that 12 of 15 patients with nonspecific neck pain who performed controlled head/neck flexion while supine experienced good to complete recovery. This exercise, and the craniocervical flexion exercise described previously involving the pressure device, were shown to produce equivalent neck flexor strength gains following 6 weeks of twice-weekly training in a group of women with mild neck pain and disability (O’Leary et al. 2007b). Exercises for the DCFs can be particularly important for patients with cervicogenic headaches, who are prone to have poor DCF strength and endurance (Watson & Trott 1993, Jull et al. 1999) and weak cervical extensors (Placzek et al. 1999).
Compared to the DCF, evidence-based recommendations for facilitating selective activation of the DCE muscles are lacking. O’Leary and colleagues (2009) proposed that flexing and extending the lower cervical spine while maintaining a neutral craniocervical spine challenges the deep lower cervical extensors while minimizing activity of the more superficial extensors (Fig. 8-8). My preferred method for training the cervical extensors is shown in Figure 8-9. This exercise provides patient-controlled, progressive resistance to the cervical extensors. Whether this exercise selectively activates the DCE is unknown. Low-intensity isometric exercises for the cervical rotators also have been suggested to facilitate co-contraction of the neck flexors and extensors (Jull et al. 2007b).
Exercises to Improve Muscular Endurance or Strength
When an acceptable foundation of muscular coordination has been established, endurance and strength conditioning may be introduced. Previous studies have shown that endurance training and/or strength training can reduce pain and disability in patients with cervical strain, degenerative or herniated discs, and chronic or recurrent neck disorders. An endurance training approach utilizing low loads should be considered initially to avoid symptom aggravation. Of note, several investigators have found endurance training and strength training to be equally efficacious in reducing chronic neck pain, at least in women (Waling et al. 2000, Ylinen et al. 2006). Exercises to increase fatigue-resistance of cervical and upper thoracic muscles may be particularly useful for patients with neck pain associated with sustained postures. Patients with neck pain have been found to adopt a more forward-head posture and have difficulty maintaining an upright posture when seated (Szeto et al. 2002). Corrected posture in sitting significantly reduces cervical, upper thoracic, shoulder, and facial muscle activity compared to forward-head posture (McLean 2005).
Individuals with neck pain may also have impaired performance of the axioscapular muscles (levator scapulae, trapezius) (Falla et al. 2004). This phenomenon may be explained by the dual influence of the axioscapular muscles on the cervical spine and the shoulder girdle (Behrsin & Maquire 1986). Weakness of the trapezius muscles in particular has been reported to coincide with neck disorders (Andersen et al. 2008). Exercises known to elicit high levels of activation in the trapezius muscles are listed in Table 8-2 (Moseley et al. 1992, Ballantyne et al. 1993, Cools et al. 2007). Performing shoulder abduction while standing with the back against a wall (Fig. 8-10) may help correct deficits in trapezius performance and structural alignment simultaneously (Sahrmann 2002). Additional exercises for the axioscapular muscles have been used in various neck rehabilitation protocols (e.g., shoulder abduction, flexion, extension, scapular retraction, wall or floor push-ups, latissimus pull-downs, arm cycling), and associated pain reduction benefits have been reported (Randlov et al. 1998, Waling et al. 2000).
Table 8-2 Exercises with High Levels of Trapezius Electromyographic Activity
Exercises with High Levels of Upper Trapezius EMG Activity |
EMG = electromyography; “T” = prone horizontal abduction, starting @ 90 degrees of abduction; ER = external rotation; empty-can = scaption w/ glenohumeral internal rotation; “Y” = prone horizontal abduction, starting ∼120 degrees of abduction.
It is worth noting that the cervical spine and head must be fixated during upper trapezius or levator scapulae activation for meaningful force transmission to the scapulae. During arm elevation, for instance, the head and cervical spine attachments of the upper trapezius must be fixed to enable the muscle to upwardly rotate the scapula. Inadequate fixation will result in craniocervical extension. Thus, in this example, the DCF muscles must be activated to stabilize the head and cervical spine by neutralizing the extension moment of the upper trapezius (Fig. 8-11) (Porterfield & DeRosa 1995). This reinforces the importance of creating a foundation of motor control/coordination in the deeper cervical muscles before higher-resistance training exercises are introduced.
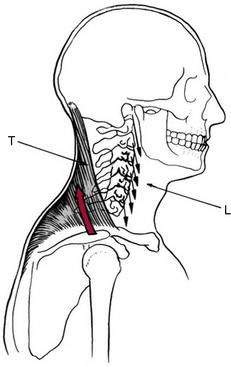
(From Porterfield JA, DeRosa C. Mechanical Neck Pain: Perspectives in Functional Anatomy. Philadelphia: WB Saunders Co. 1995. Fig. 3-6, p. 54)
Higher-resistance training of the cervical musculature may be necessary to significantly reduce pain and disability in individuals with chronic or recurrent neck disorders or to provide adequate muscular stabilization and force dissipation in select patients (e.g., wrestlers, football players). Ylinen and colleagues (2006) reported that the greatest strength gains and symptom reduction in women with chronic neck pain occurred during the first 2 months with strength training or endurance training. This suggests that a concerted effort may be required for at least 8 weeks to reap the benefits of endurance or strength training on neck pain. In another study, Ylinen et al. (2007a) reported that the gains in neck strength and motion achieved during a 12-month exercise program were largely maintained 3 years later. This suggests that patients should be encouraged to continue endurance and/or strength training, presumably with an independent “maintenance” program, for up to 1 year to prevent symptom recurrence.
Endurance and/or strength training can be particularly effective for women (Ylinen 2003, 2006, 2007). Women have a greater incidence of neck pain and higher prevalence of chronic neck pain than men (Hagen et al. 2000), which may be attributable to lower muscle strength (Vasavada et al. 2008). Maximal moments of the neck muscles are roughly 1.5 to 2.5 times lower in women than men, even when adjusted for body size (Jordan et al. 1999). Consequently the neck flexors and extensors are roughly 30% and 20% weaker, respectively, in healthy females than in males (Vasavada et al. 2008). This suggests that, in women, the mechanical demands on the neck muscles may be closer to their maximal moment-generating capacity. As a result, neck muscles may fatigue sooner in women, diminishing the muscles’ capacity to stabilize the cervical spine.
The intensity, volume (repetitions and sets), and frequency of endurance and strengthening exercises should be “titrated” to stimulate the desired adaptive changes without undesirable side effects such as symptom aggravation or poor adherence (Haskell 1994). Patients with high irritability may tolerate only brief bouts of very-low-intensity exercise through a limited arc, whereas patients with moderate or low irritability may be tolerant of longer and more intense exercise sessions.
Evidence suggests that the majority of strength gains occur in response to the first exercise set stimulus (Pollock et al. 1993, Durall et al. 2006). Accordingly, the American College of Sports Medicine (2002) recommends one set per exercise, with each set performed to volitional exhaustion. Pollock and colleagues (1993) reported that strength gains in the cervical extensors were not statistically different between healthy subjects who performed one set of 8 to 12 repetitions or two sets of 8 to 12 repetitions twice each week for 12 weeks. Randløv et al. (1998) found no difference in pain, ADLs, strength, or endurance outcomes between groups of patients who performed one set or five sets of cervical and shoulder exercises over 3 months.
Exercises to Improve Repositioning Acuity, Oculomotor Control, or Postural Stability
Research has shown that people with chronic or recurrent neck disorders or neck pain secondary to cervical spine trauma are prone to deficits in head/neck repositioning acuity (Kristjansson et al. 2003, Sjolander et al. 2008), postural stability (Michaelson et al. 2003, Treleaven et al. 2005b), and oculomotor control (Treleaven et al. 2005b)—apparently as a result of impaired afferentiation from cervical mechanoreceptors (Dejong et al. 1977). A growing body of evidence supports the use of exercises to ameliorate these deficits (Sarig-Bahat 2003).
Repositioning acuity can be fostered by using a light source (e.g., focused-beam headlamp or laser pointer affixed to a headband) and a target (e.g., dart board, archery target) (Fig. 8-12). Relocation exercises, like the one demonstrated in Figure 8-12, are commonly performed sitting but also can be done standing. Labile surfaces (e.g., ball, dome, wobble board) can be used to increase the challenge.
Oculomotor exercises, designed to improve eye/head coupling and gaze stability, can be progressed from eye movements with the head stationary to trunk and/or head movements with visual fixation on a target. These exercises can be made more challenging by increasing the speed and range of eye, head, or trunk movements or by altering backgrounds and visual targets. Exercises to improve oculomotor control (Table 8-3) have been shown to reduce dizziness and pain, and to improve postural control, cervical ROM, and function (Revel et al. 1994, Taimela et al. 2000).
Activities intended to improve postural stability are listed in Table 8-4. Postural stability exercises are often progressed from stable to labile surfaces and from bilateral to unilateral stances. These exercises are not unique to cervical spine treatment, and other techniques for challenging postural stability can be incorporated. Taimela et al. (2000) reported that patients with chronic neck pain who received eye fixation exercises, seated wobble board training, exercises to improve cervical muscle endurance and coordination, along with relaxation training and behavioral support had greater reductions in neck symptoms, improvements in general health, and improvements in their ability to work than patients who were educated on neck care or instructed in a traditional cervical spine home exercise program.
Table 8-4 Exercises to Improve Postural Stability
Exercises to Improve Mobility
Childs and colleagues (2008) suggested that flexibility exercises should be considered for the anterior/medial/posterior scalenes, upper trapezius, levator scapulae, pectoralis minor, and pectoralis major. I emphasize stretching of the SCF muscles, especially the anterior scalene and SCM (Fig. 8-13), which promote a forward-head posture when shortened. Addressing length impairments in other muscles may be beneficial for certain patients. For instance, patients with neck pain associated with an increased thoracic kyphosis may benefit from pectoralis minor stretching and/or thoracic extension self-mobilization using a chair back or foam roller to create a fulcrum (Fig. 8-14).
Patients lacking cervical rotation may benefit from active or active-assisted rotation on a partially inflated beach ball (Fig. 8-15). To facilitate rotation, a nylon or cotton strap can be used to impart an anteriorly directed force on the contralateral articular process of the hypomobile cervical segment as the patient actively rotates. This facilitated rotation exercise (performed sitting without a beach ball) was reported to be effective in reducing cervicogenic headache symptoms by 50% within 4 weeks in patients who had a loss of rotation in full flexion of 10 degrees or more (Hall et al. 2007).
A strap, pillow case, or towel can also be used to create a fulcrum for extension below a hypomobile cervical segment (Fig. 8-16A). Alternatively, patients can also be educated to use their index and/or middle fingers to create a dynamic, accommodating fulcrum, thereby “biasing” the extension movement to the restricted motion segment (Fig. 8-16B).
Nerve mobilization techniques may be beneficial for patients with neck and arm pain to facilitate improved nervous tissue gliding (Murphy et al. 2006). Coppieters et al. (2009) reported that nerve excursion was greater with a gliding technique (alternate ends of nerve are concurrently tensed and slacked) than with a tensioning technique. Readers are encouraged to consult additional sources for nerve mobilization techniques.
Patients with radicular or referred symptoms may also benefit from directionally specific exercises. McKenzie (2009) advocated performing repeated movements (with concurrent manual procedures as needed) in directions that promote distal-to-proximal symptom migration (“centralization”). At the time of this writing there were no published clinical trials using specific exercise movements to promote symptom centralization exclusively in patients with cervical radiculopathy, so the efficacy of centralization procedures for this particular subgroup of patients is unknown. Kjellman and Oberg (2002) reported that the McKenzie method was no more effective than general exercise or low-intensity ultrasound in combination with education in reducing disability in patients with nonspecific neck pain.
A popular exercise in the McKenzie approach, cervical retraction (Figure 8-17), can be used to increase flexion ROM in the upper cervical segments (Ordway et al. 1999), reduce anterior shearing of the lower cervical segments, and train the DCFs and cervical extensors in synchrony (Mayoux-Benhamou et al. 1994). This may be particularly important for patients with a more forward-head posture. As previously described, a strap or the index and middle fingers can be used to focalize the lower cervical extension that occurs during retraction.
The heterogeneous, multifactorial nature of neck pain makes it difficult to develop “one-size-fits-all” exercise programs. Clinicians should select exercises according to identified deficits, functional limitations, and the patient’s irritability level. Comprehensive exercise programs for patients with neck pain should include exercises to improve aerobic conditioning and performance of the trunk/torso muscles (See Rehabilitation Protocol 8-1).
REHABILITATION PROTOCOL 8-1 Sample Therapeutic Exercise Program for Patient with Nonspecific Neck Pain
Phase 1
Phase 2
Treatment-Based Classification of Low Back Pain
Michael P. Reiman, PT, DPT, OCS, SCS, ATC, FAAOMPT, CSCS
Low back pain (LBP) is the most prevalent of all musculoskeletal conditions and one of the primary reasons an individual visits a primary care physician (Woolf and Pfleger 2003). LBP affects nearly everyone some time in their lifetime and about 4% to 33% of the population at any given point (Woolf and Pfleger 2003).
Background
Despite extensive research into the assessment and treatment of LBP, it remains a twentieth-century health care enigma (Waddell 1996). In the 1990s much of the evidence for efficacious treatment remained elusive (van Tulder et al. 1997). The reason for some treatments failing to demonstrate efficacy in randomized controlled trials may be a false assumption that sufferers of LBP are a homogeneous group (Delitto et al. 1995). The importance of identifying homogeneous subgroups in randomized, controlled trials has been emphasized to avoid problems with sample heterogeneity (Binkley et al. 1993, Spratt et al. 1993, Delitto et al. 1995, Fritz and George 2000, Bendebba et al. 2000). The process of developing criteria for the identification of homogeneous subgroups within the LBP population is classification. Different potential types of classification schemes might include the following:
History
TBC is based on the premise that subgroups of patients with LBP can be identified from key history and clinical examination findings (Delitto et al. 1995). Delitto et al. (1995) also hypothesized that each subgroup would respond favorably to a specific intervention but only when applied to a matched subgroup’s clinical presentation. Seven different classification groups were originally described in TBC; however, recent investigations have collapsed the seven classification groups to four: manipulation, specific exercise (flexion, extension, and lateral shift patterns) and the newly added stabilization and traction (Table 8-5).
Table 8-5 Treatment-Based Classification and Matched Treatment in Patients with Acute Low Back Pain
Classification | Key History and Clinical Findings | Matched Intervention |
---|---|---|
Manipulation | Clinical prediction rule variables (Flynn et al. 2002) Variables: |
Variables (in order of importance):
Postpartum patients:
Promotion of local stabilizing muscle groups (transverse abdominis, multifidus, etc.)
Strength training of larger, global muscle groups (erector spinae, oblique abdominals, etc.)
Progression of local and global muscle group training in functional positions
The clusters of examination findings and matched interventions used in the TBC approach were principally derived from expert opinion, with little evidence support. Proper classification of patients into the appropriate category has proved reliable (Fritz and George 2000, Heiss et al. 2004, Fritz et al. 2006). The manipulation clinical prediction rule (see Table 8-5) has the best evidence support because it has been validated (Childs et al. 2004). Perhaps most important to consider regarding TBC is whether overall outcomes are improved when it is used in comparison to an alternative approach. Fritz et al. (2003) and Brennan et al. (2006) both provided support for the use of a TBC approach of classification and matched intervention for patients with LBP.
Core Stabilization Training
Barbara J. Hoogenboom, EdD, PT, SCS, ATC, and Kyle Kiesel, PT, PhD, ATC, CSCS
The common prerequisite for participation and success in dynamic function is a strong and stable core of the human body. Control of the spinal segments during upright posture is required not only for activities of daily living, but also for balance, stability, and coordination during occupational tasks and complex and high-level sports activities (Ebenbichler et al. 2001). This stability enables an individual to transmit forces from the earth through the kinetic chain of the body, resist externally applied loads and forces, and ultimately propel the body or an object using the limbs. The concept of core stabilization of the trunk and pelvis as a prerequisite for movements of the extremities was described biomechanically in 1991 (Bouisset 1991). Subsequently, core training for stabilization has become a major trend in treatment of spinal injuries and pathologies, after spinal surgery, and in training regimens for enhancement of athletic/work performance and injury prevention.
Many descriptive terms and rehabilitation programs are associated with the concept of core stability, including: abdominal bracing, lumbar stabilization, dynamic stabilization, motor control (neuromuscular) training, neutral spine control, muscular fusion, and trunk stabilization (Akuthota and Nadler 2004). The core has been conceptually described as either a box or a cylinder (Richardson et al. 1999) because of its anatomic and structural composition. The abdominals create the anterior and lateral walls, the paraspinals and gluteals form the posterior wall, and the diaphragm and pelvic floor create the top and bottom of the cylinder, respectively (Fig. 8-20).
Additionally, muscles of the hip girdle reinforce and support the bottom and sides of the cylinder. Envisioning this cylindrical system helps to understand core function as that of a dynamic muscular support system, described by some authors as the powerhouse, engine, or a “muscular corset that works as a unit to stabilize the body and spine, with and without limb movement” (Richardson et al. 1999).
Proximal stability as a requisite for distal mobility is a commonly understood principle of human movement originally described by Knott and Voss (1968) and applied in the concepts of proprioceptive neuromuscular facilitation. Nowhere is the concept of dynamic proximal stability more important than in sport. Without proximal control of the core, athletes could not effectively use the lower extremities to propel the body during running and jumping or eccentrically control the pelvis and limbs during the loading phases of running and landing. Furthermore, during activities that require use of the upper extremities to properly support or propel the body (e.g., gymnastics and swimming), manipulate an object (e.g., tennis racquet or golf club), throw objects (e.g., shot put or pitching), sufficient proximal core control is essential. Simply put, the core, as a result of its position in the middle of the human kinetic chain, serves as a link that allows for transfer of energy between the upper and lower extremities. According to Kibler et al. (1998):
When the core is functioning optimally, muscles throughout the kinetic chain also function optimally, allowing the individual to produce strong, functional movements of the extremities (Kibler et al. 1998, Andrews et al. 2004) (Table 8-6).
Even small alterations within the kinetic chain have serious repercussions on other portions of the kinetic chain and thus on skills that are based on efficient, coordinated utilization of segments (Kibler et al. 1998). Without proper stabilization and dynamic concentric and eccentric control of the trunk during functional tasks, the extremities or “transition zones” between the core and extremities can be overstressed (e.g., hip and shoulder joints), resulting in injury or tissue damage.
Anatomy
Stability of the core requires both passive (bony and ligamentous structures) and dynamic (coordinated muscular contractions) stiffness. A bony spine without the contributions of the muscular system is unable to bear essential compressive loads associated with normal upright activities and remain stable (McGill 2002). Anatomists have known for decades that a compressive load of as little as 2 kg causes buckling of the lumbar spine in the absence of muscular contractions (Morris et al. 1961). Additionally, significant microtrauma of structures within the lumbar spine can occur with as little as two degrees of segmental rotation, demonstrating the vital stabilizing function of the core muscles of the trunk (Gracovetsky et al. 1985, Gardner-Morse and Stokes 1998). Core stabilization is important not only for protection of the lumbar spine, but also to transmit the wide variety of forces that are placed on the spine and core muscles by moving limbs.
Many authors have described classifications of local and global (Richardson et al. 1999, Punjabi et al. 1989, McGill 2002) or superficial and deep (Hodges 2003b) muscles, which together contribute to stability of the core. The local or intersegmental muscles are hypothesized to function primarily as stabilizers, and the global or multisegmental muscles are hypothesized to function primarily as producers of movement (Kavcic et al. 2004) (Table 8-7). Panjabi et al. (1989) suggested that global muscles may play an important role in stabilization because of their ability to efficiently produce stiffness in the entire spinal column, as compared with local muscles acting on only a few levels. The global muscle system, although important for movement and total spinal stability, contributes primarily compressive forces to stability and is limited in its ability to control segmental shear forces (Richardson et al. 1999). Even if the global muscle system is performing adequately, the local system working insufficiently to control segmental motion may cause local instability. In fact, excessive use of global muscles in co-contraction during light functional tasks may indicate inappropriate trunk muscle control in patients with low back pain (O’Sullivan et al. 1997). Global muscles that link the trunk to the extremities (e.g. the iliacus in the lower extremity and the latissimus dorsi in the upper extremity) may actually challenge or adversely affect segmental stabilization. Spinal segment stability must be maintained in the presence of contractions of powerful global muscles during functional activities (Richardson et al. 1999). Local and global muscles both contribute to postural segmental control and general multisegmental stabilization during static and dynamic tasks (Akuthota and Nadler 2004, Richardson et al. 1999, McGill 2002); however, debate continues over which muscles are important stabilizers and how to better train the neuromuscular control system to prepare it to provide sufficient stability of the core to withstand the three dimensional torque demands imposed upon it. Cholewicki et al. (1996, 2003) reported on the basis of biomechanical analyses that no single local or global muscle owns a dominant responsibility for lumbar spine stability. Stability and movement likely depend on appropriate length and excursion, facilitated co-contractions, and coordinated muscular activity (both concentric and eccentric) in all muscles of the core. The emergent view by many prominent authors is that continual, low-level, local muscle contractions, in addition to neuromuscular coordination and motor control, are requisite for all functional activities (Richardson et al. 1999, McGill 2002). Additionally, the timing of activation and ability to demonstrate volitional control of local muscles are important precursors to higher level core strengthening. (Hodges 2003a, Macedo et al. 2009, Tsao and Hodges 2008, Richardson et al. 2004).
Local Muscles (Postural, Tonic, Segmental/Joint Stabilizers) | Global Muscles (Dynamic, Phasic, Torque Producing) |
---|---|
Intertransversarii and Interspinales (function primarily as proprioceptive organs) Multifidi Transversus abdominis Quadratus lumborum (medial portion) Diaphragm Internal oblique (posterior fibers) Iliocostalis and longissimus (lumbar portions) Psoas major (posterior portion, when working on the spine, not as a hip flexor) Hip rotators* Hip abductors* |
Rectus abdominis External oblique Internal oblique (anterior fibers) Longissimus (thoracic portion) Iliocostalis (thoracic portion) Quadratus lumborum (lateral portion) Latissimus dorsi Iliacus Psoas major (anterior portion, when working as a hip flexor) Hip adductors Hip extensors Quadriceps Hamstrings Hip rotators* Hip abductors* |
* Disagreement exists about whether these are local or global.
(Adapted from Richardson, Panjabi, McGill)
Although as a society we are fixated on the rectus abdominis and its classic “six-pack” appearance, understanding of the importance of the local muscles diminishes the functional importance of this global muscle. The rectus abdominis is a trunk flexor with a large movement capacity of the trunk that often substitutes for contractions of the important local muscles. Overuse of the rectus abdominis provides too great a flexion moment, and associated flexion of the spine, rather than stabilization. Many fitness programs incorrectly overemphasize the training of the rectus abdominis (Akuthota and Nadler 2004) and inappropriately induce shear forces by the flexion moments produced by its contraction. The shear forces induced by the contraction of the rectus abdominis are counterproductive to the goal of segmental and total core control, which is the primary goal of core strengthening or neuromuscular training. Furthermore, there are associated concerns of repeated segmental flexion of the lumbar spine and the potential for increased posterior-directed disc pressure.
Contemporary research has illuminated the roles of two important local muscle groups: the transversus abdominis (TA) (Cresswell et al. 1994, Hodges 1999) and the multifidus (Wilke et al. 1995, Hides et al. 1994). The TA, the deepest of the abdominal muscles, uses its horizontal fiber alignment and attachment to the thoracolumbar fascia to increase intraabdominal pressure (IAP), thereby making the core cylinder as a whole more stable. Although increased IAP had been associated with the control of spinal flexion forces and a decrease in load on the extensor muscles (Thomson 1988), it is probable that the TA is most important in its ability to assist in intersegmental control (Richardson et al. 1999). The TA offers “hooplike” cylindrical stresses to enhance segmental stiffness to limit both translational and rotational movement of the spine (Ebenbichler 2001, McGill and Brown 1987). Bilateral contraction of the TA performs the movement of “drawing in of the abdominal wall” (Richardson et al. 1999, p. 33), and does not produce spinal movement. The TA is active throughout the movements of both trunk flexion and extension, suggesting a unique stabilizing role during dynamic movement, different from the other abdominal muscles (Cresswell 1993, Cresswell et al. 1994). Finally, electromyographic (EMG) evidence suggests that the more internal muscles of the trunk (TA and internal obliques) behave in an anticipatory or feed-forward manner to provide proactive control of spinal stability during movements of the upper extremities (Hodges 1997, Hodges and Richardson 1997a), regardless of the direction of limb movements (Hodges and Richardson 1997a). The results of a recent study by Allison et al. (2008) suggest that the feed-forward mechanism that affects the TA originally described by Hodges and Richardson may exhibit asymmetry and directional selectivity based on task performance. As methods to examine the function of the core muscles improve, the functional specificity of training will likely become more differentiated.
Among the posterior spinal muscles, the multifidus is important for its contribution to control of the neutral or stable position of the spine (Punjabi et al. 1989, Wilke et al. 1995). As a result of its unique anatomic structure and segmental innervation, the multifidus is important for providing segmental stiffness, proprioceptive input to the central nervous system, and motion control. The tonically active multifidus is reported to offer two thirds of the increase in segmental stiffness at the L4-L5 segment when contracted (Wilke et al. 1995). Dysfunction of the multifidus that occurs after injury to the spine (Hides et al. 1994) makes this muscle group an important focus for rehabilitation (Macdonald et al. 2006). Clinical and preliminary experimental evidence suggests that a biomechanically beneficial co-contraction of the TA and multifidi occurs during specific exercises (Richardson et al. 1999) (Fig. 8-21). This specific and specialized relationship provides increased stiffness within spinal structures, offering critical tonic cylindric stabilization for the core, and is the basis for rehabilitation.
The importance of the top and bottom of the core, the diaphragm, and the pelvic floor, respectively, must not be underestimated. The diaphragm, like the TA, functions in anticipatory postural control by firing prior to extremity musculature during the movement of shoulder flexion (Hodges, Butler, and McKenzie, 1997). Also, contraction of the diaphragm occurs concurrently with activation of the TA but independent of the phase of respiration (Hodges, Butler, and McKenzie, 1997). Although beyond the scope of this chapter discussion, restoring diaphragmatic breathing may be an important component of a core training to consider prior to the initiation of core strengthening (Akuthota and Nadler 2004). The pelvic floor has been shown to be active during lifting tasks and to be instrumental in increased activation of the TA when voluntarily contracted (Richardson et al. 1999). Conversely, EMG activity of the pubococcygeus increased during activation of the abdominal muscles. Thus, the bottom of the cylinder, the pelvic floor, cannot be ignored during core strengthening (Table 8-8).
Table 8-8 Muscle Group Categories
Specific Muscle Group | Primary Responsibility |
---|---|
Transversus abdominis | Maintaining neutral lumbopelvic position by abdominal drawing/cylindrical stabilization |
Ipsilateral internal and external obliques | Trunk side flexion |
Contralateral internal and external obliques | Trunk rotation |
Multifidus | Segmental lumbar stability |
Erector spinae | Trunk extensor |
Gluteus maximus | Hip extensor |
Gluteus medius | Hip abductor/lateral rotator and eccentric control of pelvic alignment |
Hip lateral rotators | Eccentric control of internal rotation of limb |
Diaphragm | Proximal “cylinder” stabilization and intraabdominal pressure |
Pelvic floor | Distal “cylinder” stabilization and intraabdominal pressure |
Mechanisms of Injury to the Core
The early portion of this chapter describes many mechanisms of injury and possible sources of pain and progressive dysfunction. Spinal injuries occur in deconditioned and well-conditioned individuals alike. Injury to the trunk, abdomen, and low back is not gender specific, and males and females are injured in similar mechanisms. Cholewicki et al. (2000) suggested that a common factor for injury to athletes may be the inability to generate sufficient core stability to resist external forces imposed on the body during high-speed events. Other authors suggest a deficient endurance of the trunk stabilizing musculature that predisposes individuals to the negative effects of repetitive forces over time (Richardson et al. 1999), motor control deficits, and imbalances of the local muscles (TA and multifidus) and the global musculature (rectus abdominis and erector spinae). A weak or inefficient core may result in altered functional movements, altered postures, and an increased potential for both macrotraumatic and microtraumatic injury in workers and athletes (Andrews et al. 2004).
Two examples of microtraumatic injuries that can occur in workers and athletes are spondylolysis and spondylolisthesis. The athletic population is more prone to these conditions and is more likely to be symptomatic from these injuries than nonathletes because of the extreme extension/flexion reversals in trunk posture demanded by many sports. Spondylolytic microfracture of the pars is believed to occur as a result of shear forces that occur during repetitive flexion and extension (Swedan 2001). Athletes with high rates of this type of microtraumatic injury include gymnasts (Hall and Thein Brody 1999), divers, figure skaters, swimmers who perform the butterfly stroke (Swedan 2001), and volleyball players (Hall and Thein Brody 1999). In fact, gymnasts younger than age 24 have a four times greater incidence of spondylolysis than the general female population (Swedan 2001). Spondylolytic microfractures can lead to a subsequent spondylolisthesis condition.
Microtraumatic injuries may also occur as a result of muscular imbalances, from uncontrolled shear forces acting on the spine (Swedan 2001, Hall and Thein Brody 1999), or because of a lack of synchronized muscular control and stabilization by the core musculature. Sports such as golf, diving, and softball provide potential mechanisms for microtraumatic injury to the core induced in a similar manner. Rather than being a result of straight plane flexion and extension, these injuries are related to extreme rotation, often in combination with extension. Careful assessment of motor strategies and subsequent corrective movement retraining by the rehabilitation professional may be a key to prevention of many microtraumatic injuries in a wide variety of athletes and workers.
Findings from diagnostic ultrasound measurements taken on patients with acute LBP indicate that rapid multifidus atrophy, as measured by cross-sectional area, occurs on the same side as the low back pain, even as quickly as within 24 hours after injury (Hides et al. 1994). This effect is likely a result of muscular inhibition. After a first episode of acute low back pain, recovery of multifidus function and cross-sectional area does not occur without specific, targeted intervention (Hides et al. 1996). In a study of male high-performance rowing athletes, multifidus muscle dysfunction was present despite their rigorous training. In this same study, the fatigue rates of the multifidus were used to successfully discriminate between controls and subjects with low back pain (Roy et al. 1990). Fortunately, regular training with specific, localized muscular contractions of the core can facilitate recovery of the multifidus muscle (Roy et al. 1990). Understanding the support function of the local muscles is relevant to treatment of a wide variety of conditions including generalized low back pain, disc derangement, facet joint irritation and dysfunctions, sacroiliac dysfunction, incontinence, and respiratory disorders (Richardson et al. 1999).
Rehabilitation: Assessment and Intervention
Examination
The functions of the deep, local muscles of the core are best objectively examined using fine-wire EMG (Gardner-Morse and Stokes 1998, Stokes et al. 2003) or real-time ultrasound (Hodges 2003a, Kiesel 2007a, Koppenhaver et al. 2009, Teyhen 2006) and magnetic resonance imaging (MRI) (Kiesel 2007a). The use of real-time ultrasound by the rehabilitation professional has been designated as rehabilitative ultrasound imaging (RUSI) (Teyhen 2006).
RUSI can be used for various aspects of spinal rehabilitation including assessment of muscle activation by the measurement of thickness change from rest to activation and muscle girth. Thickness-change deficits have been identified in both the TA and lumbar multifidus muscles in patients with LBP. Additionally, research has been conducted on the use of RUSI for biofeedback during motor control training (Hides et al. 2006, Kiesel 2007a, Henry and Westervelt 2005, Teyhen et al. 2005, Frantz Pressler et al. 2006).
Although real–time ultrasound is not widely used in the clinical setting, its use has been growing steadily and perhaps clinical practice of the future will allow for increased use of this tool for both examination and intervention (Teyhen 2007, Teyhen et al. 2007).
Simple, reliable, and objective clinical tests for dynamic motor control of the core are not readily available. Clinically, therapists can use manual muscle tests that examine isometric holding of muscles (e.g., Kendall tests for upper and lower abdominals or Sahrmann tests for lower abdominals), positional holding tests (plank or side plank) (Fig. 8-22) for endurance in isometric positions, and pressure biofeedback to assess the ability of a patient to hold the core stable during dynamic tasks. Sahrmann (2002) subscribes to the premise of activating the lower abdominal group (TA and external obliques) to provide proper lumbopelvic control while adding dynamic LE movements of progressive levels of difficulty. She originally described a functional grading system on a 0 to 5 scale, which was later expanded to include several descriptors of lower-level function (0.3–0.5). This system provides a construct to assist the therapist in determining the appropriate starting point for the lower abdominal exercise progression. The patient is given the command to “pull the belly button toward the spine,” which recruits the TA (Goldman 1987). The assessment grades indicate that the patient is able to successfully maintain appropriate lumbopelvic control with the specific LE perturbation. Sahrmann’s lower abdominal scale is shown in Table 8-8 (Sahrmann 2002), and it should be noted that it does not correlate with the Kendall and McCreary (1983) grading system of 1 to 5 as used in manual muscle testing.
A subjective clinical test for the multifidus involves the activation of the multifidus at various segments under the palpating fingers of a therapist (Richardson et al. 1999). The multifidus activation test is performed with the patient prone using the command, “Gently swell out your muscles under my fingers without using your spine or pelvis. Hold the contraction while breathing normally” (Richardson et al). This test includes both side-to-side and multiple-level comparisons to assess for segmental activation or inhibition of the lumbar multifidi (Fig. 8-23).
Richardson et al. (1999) described the abdominal drawing in test for function of the deep abdominal muscles and subsequently developed the air-filled pressure biofeedback unit in an attempt to quantify this task. This clinical test of deep muscle co-contraction is performed in prone, by performing the drawing in task while concurrently using the pressure biofeedback device (Richardson et al. 1999). The authors are unaware of any reliability studies related to the use of the pressure biofeedback device, and future reliability studies would enhance the use of this device (Fig. 8-24).
In addition to the basic performance of this task, the physical therapist must also monitor the task for the ability to hold a tonic, smooth contraction of the TA without resorting to the use of the global muscles. The prone position is useful because it minimizes the ability of the patient to use the rectus abdominis for the contraction. In a single-blind study of subjects with and without back pain, it was determined that only 10% of patients with a history of LBP could perform the TA test using The Stabillizer™ (Chatanooga Rehabilitation Products, Chatanooga,TN) as compared to 82% of the patients without back pain (Richardson et al. 1999, 2004).
Testing of control of lumbopelvic posture as a measure of core stability against a load is accomplished clinically by performing various leg-loading activities in supine using the pressure biofeedback device (Figs. 8-25 and 8-26). The test examines the ability of the core to hold the lumbopelvic region still or steady during various progressive leg-loading activities (Sahrmann 2002). The pressure biofeedback device provides information to the patient about loss of support or neutral position during functional tasks. Posterior pelvic tilt motion of the pelvis results in an increase in baseline pressure, whereas anterior pelvic tilt results in a decrease in pressure from baseline. Other tasks in prone and supine positions, such as movement of the extremities and loading activities, can be assessed. Effective contraction of the TA solicits a co-contraction of the multifidus and vice versa (Richardson et al. 1999).
Unfortunately, the use of the pressure biofeedback device is limited to activities that involve a surface to offer counterforce to read the pressure exerted onto the device. It is not yet clinically possible to evaluate dynamic core stability and measure activity of deep stabilizing musculature in the upright position, and clinicians must rely on motor performance taught in other positions to “carry over” to more demanding positions. Richardson et al. (1999, p. 110) advocated frequent repeat prone formal testing, using the biofeedback device, to check efficiency of the local core stabilizers because “assessment in functional tasks by means of observation and palpation only does not give a reliable indication of the improvement in deep muscle capacity.”
Henry and Westervelt (2005) explored the use of real-time ultrasound for teaching the abdominal “drawing in” or abdominal hollowing maneuver to healthy subjects. They found that this feedback tool decreased the number of trials needed to consistently perform this maneuver. The authors stated that real-time ultrasound is a beneficial teaching tool for facilitating consistency of performance of the abdominal hollowing maneuver as compared to verbal and cutaneous feedback, which are the teaching methods used presently by most clinicians. Teyhen et al. (2005) demonstrated that real-time ultrasound imaging can be used reliably to measure the thickness of the TA in both contracted and noncontracted states. Studies to assess the value of using RUSI for feedback when learning to volitionally activate the multifidus have also shown favorable results. Van et al. (2006) also demonstrated that visual feedback improved the ability of subjects to perform the multifidus swelling exercise and that variable feedback was superior to constant feedback on long-term retention trials (Herbert et al. 2008).
Exercises/Training Techniques
Contemporary thinkers refer to the concept of neuromuscular retraining of the core (contributing to segmental stability and stiffness) rather than pure strengthening of muscles supporting the core (Ebenbichler et al. 2001, Akuthota and Nadler 2004, Richardson et al. 1999). To effectively provide core stabilization, the patient must use the neuromuscular system to coordinate contractions of many local and global muscles that are able to influence the position of the pelvis, hips, and spine. In vitro studies demonstrated that local muscles can effectively provide segmental stabilization of the lumbar spine (Wilke et al. 1995). Thus, recruitment and tonic activity of local musculature are the hallmark of contemporary rehabilitation and training activities, as compared with older programs that focused on contractions of the global musculature. Motor control programs for functional performance of skills used by patients (both healthy and injured) are widely varied, are complex, and may involve alterations in both feedback and feedforward mechanisms (Richardson et al. 1999). Multifidus muscle dysfunction, which may be present in highly skilled athletes despite their excellently trained condition, supports the use of an alternate (training of local stabilizers) exercise approach rather than the traditional exercise regimen often used for core strengthening with a focus on global musculature (Roy et al. 1990). Jemmet (2003) described such an alternate approach as a shift away from treating multifidus dysfunction using a strengthening model to a model based on motor re-education.
According to Richardson, Jull, Hodges, and Hides (1999), rehabilitation has three distinct phases: (1) formal motor skill training, (2) gradual incorporation into light functional tasks, and (3) progression to heavy-load functional tasks. The last phase of rehabilitation must be tailored to include high-level work and sport-specific demands performed by the patient in a wide variety of body positions, whether during a prevention or rehabilitation program. Therapeutic exercise in more advanced activities must have two different goals in mind:
Current evidence supports the concept that training efforts should not focus on any single muscle; rather, they should have components of local and global muscle training (Cholewicki and McGill 1996, Cholewicki and Van Vliet 2002, McGill 2002).
Where to Begin: Formal Motor Skill Training
The key to training the local stabilizers is teaching the abdominal drawing in or hollowing exercise, an action specific to the TA. Drawing in of the abdominal wall was originally described by Kendall and McCreary (1983) and later further described by DeTroyer et al (1990) as “drawing the belly in.” The patient must be cued to “narrow your waist” or “pull your belly button away from your waistband” without using the other abdominal muscles or holding his or her breath.
Sahrmann (2002) described using lower-level exercises initially to develop control versus higher-level exercises focused on more strength development. Her lower abdominal exercise progression, directed toward proper recruitment of both the obliques and TA, is initiated in a supine position with hips and knee flexed. The patient is instructed to “pull your belly button toward the spine” for all exercise levels described in Table 8-8. Based on the results of the evaluation described earlier in this section, the patient must maintain lumbopelvic control while adding the specific LE perturbations. The exercise progression is essentially the same as the assessment, only done in repetition and to the tolerance of the patient. It is important that if the patient is no longer able to maintain proper lumbopelvic control, then the exercise is stopped. The therapist needs to use good clinical judgment to determine if the patient is at the appropriate level or if the exercise needs to be regressed or progressed based on periodic reassessment.
Another important feature of teaching the skill of abdominal bracing is that the patient understands the corset-like circular function of the TA so he or she can envision it working to draw in the waist or hollow the abdomen. The difficulty of core exercises must be tailored to the level of achievement of the abdominal drawing in exercise (Andrews et al. 2004), and progressed from there. The patient must also understand that this action occurs without producing any movement of the spine and requires only low-level activation of the TA, <10% of the maximal voluntary isometric contraction (McGill 2002, Richardson et al. 1999, 2004). Movement of the spine or pelvis indicates that global muscles are being used to attempt the task, rather than effectively recruiting and using the local TA. The four-point or quadruped position can be used initially to teach this task and then other positional instruction can be added (e.g., supine, prone, sitting, standing, and half kneeling) (Fig. 8-27).
Exercises should be progressed in difficulty only when the patient can maintain spinal stability and contraction of the TA while breathing normally (Andrews et al. 2004). The drawing in maneuver should then be taught in the plank exercise, which helps to develop endurance of the TA and multifidus in a semifunctional position.
Maintaining the drawing in maneuver during the ideal lumbopelvic position is critical because the patient often has a tendency to be too flexed or too extended in the trunk. Use the cue “keep your back flat like a table top” while performing the plank exercise for a timed bout (Fig. 8-28). Patients often have difficulty assuming and holding the plank position because of poor abdominal performance or concurrent UE weightbearing difficulty. Athletes with excellent core control and endurance have been known to hold this position for more than 3 minutes. The average athlete begins with 30 to 60 seconds and works up in time duration. For patients who have trouble maintaining the position for 30 seconds, an alternate position with the knees flexed (modified position like a push-up for women) can be used, which decreases core demands by shortening the control lever arm. Many patients are challenged by 15 seconds or less.
Finally, appropriate recruitment of the multifidi for segmental stability is critical in managing low back pain in patients/athletes. After the patient learns how to recruit the multifidi in the prone position (see earlier prone multifidus test of Richardson, Hodges, and Hides), then he or she progresses to the quadruped position for training multifidus activation and neuromuscular training. In a multifidus neuromuscular control exercise, the patient in quadruped is cued to lift the knee straight toward the ceiling approximately 1 inch without lifting the toes off the ground or laterally shifting the hips or trunk. If the patient has difficulty performing this exercise properly, it may be necessary to stabilize the contralateral side against a stable surface (e.g., wall or couch). The progression for this exercise is placement of a towel roll under the contralateral knee to increase the range of segmental lumbar motion (Fig. 8-29).
Incorporation Into Light Functional Tasks
Once the patient is able to properly demonstrate recruitment and control of the local core exercises (e.g., drawing in for TA, plank, multifidi), the program must be advanced to include light dynamic functional tasks. The next stage is to progress to more general functional exercises that target the key muscle groups in isolation but move closer toward the specific movement and loading patterns necessary for the patient’s activities. The key muscle groups are listed in Table 8-9 with their primary responsibility as they relate to the neuromusculoskeletal system.
Test position: In supine, all tests begin in hook-lying position. Instruct the patient to maintain a flat, silent, stable lumbar spine while performing the following movement. | |
Levels of Difficulty | |
Level 0.3 | Single bent knee lift ∼2 inches off the table, while other foot remains on the table. Keep pelvis silent. |
Level 0.4 | Using hands, passively hold one knee firmly to the chest (hip >90 degrees), then perform single bent knee lift as in 0.3. |
Level 0.5 | Using hands, passively hold one knee lightly toward chest (hip at 90 degrees), then perform single bent knee lift as in 0.3. |
Level 1A | Single bent knee lift (as 0.3), with opposite hip held actively at greater than 90 degrees of flexion. |
Level 1B | Single bent knee lift (as 0.3), opposite hip held actively at 90 degrees of flexion. |
Level 2 | Bring both hips to 90 degrees of flexion, actively hold one there while sliding the other heel on table until knee is fully extended, return. |
Level 3 | Bring both hips to 90 degrees of flexion, actively hold one there while gliding the other heel to (glide = unsupported by table) until full knee extension, return. |
Level 4 | Double-leg knee extension (heels supported by table). |
Level 5 | Double-leg knee extension (heels unsupported by table). |
Note: Each movement is performed unilaterally, to assess for rotational function, until grades 4 and 5, which are performed bilaterally.
(Adapted from Sahrmann SA. Diagnosis and Treatment of Movement System Impairments. St. Louis: Mosby Inc., 2002.)
Functional progression of core stabilization activities is the most important part of the program when used either for prevention or rehabilitation. Functional progressions require therapist knowledge of work- or sport-specific demands along with a good sense of creativity. Without progression through relevant functional tasks, appropriate motor learning cannot be achieved because carryover from lower functional or developmental-level tasks cannot be assumed. The most basic functional task requiring tonic deep muscular support is the transition from sitting to standing. Maintenance of neutral core while rising to standing from a sitting posture is functional for almost every patient (Fig. 8-30). Instruction in monitoring of the core and repetition of this simple task is a low-level start. After sit to stand is mastered, the next functionally relevant task is walking. An early dynamic exercise is to teach the patient to activate the TA and multifidus while walking and breathing normally (Richardson et al. 1999). Difficulty can be added to exercises by changing body positions, using less stable positions (e.g., therapeutic balls, foam rollers, Dyna Discs, or other unstable surfaces), adding equipment for perturbations (e.g., Theraband, tubing, or cable pulleys), or increasing load during tasks. The plank can be made more difficult and somewhat dynamic in the side plank position by adding UE and LE challenges (Fig. 8-31). Examples of additional developmental postures to use during dynamic exercises include tall kneeling and half kneeling, shown in Figures 8-32 and 8-33.
Finally, the practice of isolated training of a specific group of muscles to reduce compressive loads on the spine must be examined (Kavcic et al. 2004). Strength training of the lumbar extensors has traditionally been a part of low back rehabilitation. The lumbar extensors are considered global muscles. They serve as prime movers into trunk extension and may need to be included in strengthening exercise programs for athletes after injury to the low back. Many exercises directed at strengthening the lumbar extensors as a group, however, fail to place the pelvis in a stable, neutral position and prevent substitution of the gluteals. In fact, many commercially available “low back strengthening” exercise machines do not attempt to stabilize the pelvis and place the spine in a lordotic or hyperextended position. Graves et al. (1994) studied the effect of pelvic stabilization on retraining of the lumbar extensors and found that strengthening should be performed with the pelvis stabilized. It should be noted, however, that in their research they used a machine that provided passive external stabilization to the lumbar spine (because of machine design), but we believe that a successfully maintained active pelvic stabilization may be more appropriate. Techniques of core stabilization using local musculature can be applied during various strengthening tasks involving global muscles of the trunk and extremities. Kavcic et al. (2004) concluded that it is “justifiable to train motor patterns that involve the contribution of many of the potentially important lumbar spine stabilizers” during rehabilitation and that focus on a single muscle or group appears to be misdirected if the goal is the development of a stable spine.
Table 8-10 gives several examples of exercise progression for core stabilization.
Table 8-10 Progression of Core Stabilization Exercises
Beginning Exercises | Intermediate Exercises | Advanced Exercises |
---|---|---|
Isometric holding exercises: |
UE = upper extremity; LE = lower extremity.
Notes: It is important to provide the patient with clear explanations and use a variety of teaching “tools” such as verbal analogies/descriptors, visual aides, clinician demonstration, and tactile cues. The patient must be educated to the type of skill and motor retraining that needs to occur. This includes a discussion about precision and intensity of contractions (mild to moderate contractions of the involved musculature, rather than maximal contractions, are indicated). The patient must understand from the outset the subtlety and precise natures of the contractions involved and then apply to all activities. Be certain to monitor for signs of unwanted global muscle activity during activities. These signs include pelvic or spine movement, rib cage depression, no change in diameter of the abdominal wall (should draw in laterally and anteriorly), aberrant breathing patterns, inability to perform normal breathing during tasks, and coactivation of thoracic portions of erector spinae. Methods of observation include visual observation, palpation, and electromyographic assessment. Be creative in designing and progressing activities of core stabilization; your only limitation is your own imagination.
Transversus Abdominis with UE Perturbations
Ipsilateral IO/EO—Side Flexion
Contralateral IO/EO—Rotation
Diaphragm and Pelvic Floor
Dynamic, Progressive Functional Challenges
Lunge onto Unstable Surface Using Exercise Ball (Fig. 8-38)
Description: Standing on one leg,the athlete lunges forward with the other leg and places foot onto the BOSU™ ball (Fig. 8-38). The athlete is instructed to perform the lunge while maintaining a stable core and concurrently reaching with the core ball. Note: External perturbation may be provided by the therapist using a dowel to push on the patient’s pelvis during the lunge.
Squat with Overhead Sustained Lift
Description: With feet shoulder-width apart, knees slightly bent, spine in neutral, the athlete starts by lifting a piece of dowel overhead and maintaining while performing a form squat (Fig. 8-39). The emphasis is on having the patient maintain a neutral spine position throughout the entire squatting motion. This exercise is progressed by adding weights (e.g., dumbbells, barbell, or barbell with weights) in the overhead position.
Four-Way Tubing Pulls on Unstable Surface
Description: With tubing anchored low, the athlete places one end around one ankle while standing on an unstable surface (e.g., Airex pad, foam roller, or Dyna Disc™ [Dynadisk R. Exertools, Petaluma, CA]) (Fig. 8-40). With the stance leg in slight knee flexion, the athlete is instructed to pull against the tubing away from the anchor point. The exercise is repeated with changes in direction for hip extension, abduction, flexion, and adduction. The emphasis is on having the athlete maintain proper lumbopelvic alignment, especially in the frontal and sagittal planes.
Overhead Medicine Ball Toss Kneeling on BOSU™ Ball
Description: The patient kneels on a BOSU™ ball and performs an overhead toss and catch with a medicine ball either with another person or against a Plyoback Rebounder (Plyoback Elite Rebounder®, Exertools, Petaluma, CA) (Fig. 8-41). The emphasis is on having the patient maintain good trunk alignment, avoiding flexion or extension of the lumbar spine. This can be made more difficult by having the patient not allow the feet to touch the ground.
Single-Limb Stance on BOSU™ Ball with Repeated Rowing
Description: With the tubing anchored at chest height the patient assumes a single-limb stance in a partial-squat position on the BOSU™ and performs repeated rowing motions with the tubing (Fig. 8-42). The emphasis is on having the patient maintain neutral lumbopelvic alignment, especially in the frontal and sagittal planes.
Half-Kneeling Medicine Ball Side Toss
Description: While in a half-kneeling position (near leg up) and sideways to the Plyoback Rebounder, the patient performs a rotational toss and catch, allowing trunk rotation to occur in a controlled midrange of motion (Fig. 8-43). The emphasis is on having the obliques not only generate the rotational movement during the toss phase, but also control the eccentric movement during the catching phase. The exercise is performed from both sides.
Diagonal Tubing Pulls on Unstable Surfaces
Description: Diagonal tubing pulls can be done in both D1 and D2 patterns either with single (Fig. 8-44) or double arm pulls (Fig. 8-45). By placing the athlete on unstable surfaces (e.g., Airex pad, foam rollers, Dyna Disc™, or BOSU™) and in different stance positions (e.g., double limb, single limb, tandem stance, lunge position, squat position, or sport-specific stance), the exercise can be tailored to meet the needs of any athlete.
Core Ball Punch on Unstable Surface
Single-Limb Dead Lift
Description: The patient stands on one limb with the knee slightly flexed (Fig. 8-47). Maintaining proper spine and pelvis and upright trunk, the patient lowers one or both hands toward the floor while flexing at the hip, and then returns to the upright starting position. It is imperative that the slight lordosis (neutral spine) is not lost during the motion. The movement is accomplished by the gluteus maximus and, to a lesser extent, the hamstrings on the stance leg. This motion is also known as a flat back or golfer’s lift and may be functional for lifting light loads.
Conclusion
Core stability training is increasing in popularity as clinicians have become aware of the relationship that a poorly functioning core has to performance and injury. Experts agree that retraining of the deep local muscles of the core must be incorporated into rehabilitation of patients with injury to the low back to effectively accomplish functional rehabilitation (Richardson et al. 1999). Core training routines can be creatively designed and progressed by the rehabilitation professional to facilitate complete return to occupation or sport. It must be acknowledged that the concept of core stabilization is not intended to replace many other systems and philosophies of treatment; rather it is but one part of the big picture of spinal rehabilitation. Motor re-education of the deep core stabilizers may need to precede more general exercise and be incorporated in subsequent exercises to successfully educate or re-educate deep local muscles in their essential stabilizing function. Local muscular exercises must be carefully assessed, taught, and mastered using available clinical tools and techniques before training the global muscles of the core. Successful use of local muscles during exercises and function may prevent or correct motor control problems that contribute to recurrent injury and incorrect use of the muscles of the core. As more evidence emerges regarding the use of RUSI, and it becomes more available to clinicians, it likely will gain popularity as a tool for clinical muscular assessment and biofeedback during rehabilitation. No matter the exercise approach or applied theory, the clinical outcomes of core stability programs have not been well researched. The specific exercises and theories described in this chapter need further investigation both for rehabilitation of injuries of the low back, pelvis, and associated core muscles and for use in strength training and performance-enhancement programs. Incorporation of core stabilization techniques into rehabilitative, fitness, preventive, and wellness programs will continue to be important in the ever-evolving practice of spinal rehabilitation.
McKenzie Approach to Low Back Pain
Barbara J. Hoogenboom, EdD, PT, SCS, ATC, and Jolene Bennett, PT, MA, OCS, ATC, Cert MDT
The rehabilitation professional caring for patients with low back pain must use an evidence-based, efficient, and effective treatment approach. The objectives of this section are to present an introduction to the McKenzie classification system, describe evaluation techniques, and present treatment interventions common to the McKenzie system for the lumbar spine. A case study describing lumbar pain/pathology in a baseball player is used to illustrate the importance of a thorough evaluation process to determine the correct treatment approach. This section of the chapter is to be considered only as an introduction to the McKenzie system and is not to be used as a substitute for attendance at the courses offered by the McKenzie Institute or thorough reading of the textbooks related to the lumbar spine written by Robin McKenzie and Stephen May (McKenzie and May 2004).
Types of Pain
As noted in the previous section of this chapter, any anatomic structure that has a nerve supply is capable of causing pain or nociceptive impulses. In the lumbar spine these structures may include the capsules of the facet and sacroiliac joints, the outer part of the intervertebral discs, the interspinous and longitudinal ligaments, the vertebral bodies, the dura mater, nerve root sleeve, connective tissue of nerves, blood vessels of the spinal canal, or local muscles (Bogduk 1997, Butler 1991). The large number of nociceptors within the lumbar region makes it impossible, even for the most experienced clinician, to determine the exact tissue that is the source of the pain. Kuslich et al. (1991) determined that compressed nerve roots are the source of significant leg pain and the outer wall of the annulus fibrosus is the source of significant back pain. Other authors determined that most pain in the lumbar spine and leg is attributable to the intervertebral disc, whereas the facet and sacroiliac joints play a lesser role in the genesis of low back pain (Bogduk 1993 and 1994).
Somatic pain is described as deep and aching and is vague and hard to localize. Many clinicians believe that the deeper the affected structure, the more widespread the distribution of the pain (Bogduk 1994). Nociceptors present in the facet and sacroiliac joints are capable of referring pain down the leg (McKenzie and May 2004). The stronger the noxious stimulus, the more distal the pain travels down the leg. Neurogenic pain arises from pressure on a nerve root(s), which in turn causes further inflammation. Neurogenic pain is often severe and shooting in nature and felt in a narrow area of the leg, as opposed to somatic pain, which usually has a broad distribution of pain and achiness. All nerve root pain is felt in the leg, and often the leg pain is worse than the back pain. Motor and sensory abnormalities, if present, indicate significant inflammation and compression on the nerve root and should be observed closely by the clinician. Typically, inflammation of the L4 nerve root refers pain down the anterior aspect of the thigh, L5 refers pain down the lateral aspect of the leg, and S1 refers pain down the posterior aspect of the leg. This distribution can be variable among patients and should not be interpreted rigidly (McKenzie and May 2004). As noted earlier, the nociceptive source of leg pain can be from somatic and/or neurogenic sources; frequently, it is a combination of both.
Activation of Pain
Mechanical pain occurs when a mechanical force is applied to any tissue and that stress deforms the local nociceptors present within the tissue. Mechanical pain is intermittent and diminishes or totally resolves if the mechanical stress is removed. An example of this is placing a hyperextension force on a finger; by maintaining this position the nociceptive pain system is stimulated. When the force is released, the mechanical pain resolves. No chemical treatment will diminish pain arising from a mechanical deformation and no mechanical treatment will totally resolve pain arising from chemical stress. These simple principles are the basis for the McKenzie approach to treating any type of musculoskeletal pain with repeated movements (McKenzie and May 2004).
The degree of chemical and mechanical pain present with each individual injury must be determined during the subjective and objective examination and subsequent evaluation. Then, each component of pain must be treated accordingly. Table 8-11 gives some guidelines in determining the source of pain during examination and evaluation.
Table 8-11 Key Factors of Chemical and Mechanical Types of Pain (McKenzie)
Key Factors of Chemical Pain | Key Factors of Mechanical Pain |
---|---|
Constant pain | Intermittent pain |
Pain appears shortly after injury | Repeated movements cause lasting reduction in pain, abolish and centralize pain |
Cardinal signs of inflammation may be present (swelling, redness, heat, tenderness) Lasting aggravation of pain by all repeated movements |
Directional preference One direction of movement will decrease pain, whereas the opposite direction will increase pain |
No movement found that reduces, abolishes, or centralizes pain | — |
Stages of Tissue Healing
The final stage is remodeling, which occurs from the fifth week on. In this stage it is important to apply regular stress sufficient to provide tension without damage so the soft tissues elongate and strengthen. Return to full range of motion in all directions of movement is the goal and should be present to achieve return to full function (McKenzie and May 2004). The principles of treatment for each stage are summarized in Table 8-12.
Week 1 | Weeks 2–4 | Weeks 5+ |
---|---|---|
Injury and Inflammation | Repair and Healing | Remodeling |
↓ | ↓ | ↓ |
Minimize further mechanical deformation | Gentle tension and loading without lasting pain | Prevent contractures by increasing tensile load to tissue |
Decrease inflammation | Work into edge of stiffness but no lasting pain cessation of exercise | Normal return to full range of motion |
Relative rest | Patient in control of forces of pressure at end range | Overpressure applied to end range of repeated movements either by patient or therapist |
Protected movements, with little to no stress | Progressive return to normal loads and tension | Return to full functional level with all activities |
Intervertebral Disc
The second, internal displacement of the disc material has also been determined to be a potential source of pain. The position of the discal material is influenced by spinal postures and prolonged postural positions of flexion or extension, as originally described by Nachemson (1992) (Fig. 8-48). Such conditions cause disc material displacement according to direction. In both of these cases the pain that is caused results from uneven loading of the intervertebral disc, which may cause neurogenic pain from pressure on the nerve root (Bogduk 1997) (Fig. 8-49). A large volume of literature exists regarding disc function and mechanics; however, more discussion is beyond the scope of this manuscript.
What is important to remember is that the disc is a mobile tissue, affected both by movement and sustained postures. This fundamental concept is the cornerstone of the McKenzie approach for treatment of spinal pain. The McKenzie system describes many types of mechanical back pain and hypothesizes that changing mechanical loads on the intervertebral disc will either increase or decrease pain, causing peripheralization or centralization of the neurogenic symptoms noted by the patient. Asymmetric loading of the disc will displace the nucleus pulposus (NP) to the area of least pressure. If the lumbar spine is flexed, the force is highest in the anterior aspect of the disc and thus the NP will be displaced in the opposite direction. In this case the NP would be displaced posteriorly within the disc. Many studies have supported this hypothesis and have shown that posterior displacement of the NP occurs with lumbar flexion and anterior displacement of the NP occurs with lumbar extension (Schnebel et al. 1988, Beattie et al. 1994, Fennell et al. 1996, Brault et al. 1997, Edmondstone et al. 2000). Authors consistently report that the aging NP becomes more fibrous over a lifetime and therefore demonstrates less predictable responses to repeated movements and may be displaced less easily in the older patient than in younger patients (Schnebel et al. 1988, Beattie et al. 1994).
In this section the term disc herniation is used as a nonspecific term to indicate disc material displacement and/or fissure or disruption. The McKenzie approach uses repeated movements in the sagittal plane to evaluate and treat these disruptions. The McKenzie classification term for this is a derangement, which is discussed in detail in the next section. A derangement can be labeled as reducible or irreducible based on the presence or absence of the hydrostatic mechanism within the disc wall. If the herniation is present in a disc where the outer wall is intact (hydrostatic mechanism intact), then it is reducible and repeated movements would corect the mechanical stresses on the disc. If the herniation is present in a disc where the outer wall is not intact (hydrostatic mechanism disrupted), then the derangement is irreducible and repeated movements will not improve the pain or symptoms (McKenzie and May 2004).
The direction of herniation is important because this directs the treatment approach. More than 50% of derangements appear to start centrally in the disc, whereas approximately 25% start posterolaterally within the disc. As the derangement extends into the dura and nerve root, more than 50% displace posterolaterally and 25% displace posterocentrally. This suggests that most derangements occur in the sagittal plane, so lumbar flexion and extension are part of the mechanism of injury and the avenue for repeated movement treatment. Fewer than 10% of derangements herniate directly laterally requiring torsional or lateral forces to be a component of the treatment. Most derangements occur at the L4-L5 and L5-S1 levels (McKenzie and May 2004).
McKenzie Classification of Syndromes
The McKenzie classification consists of three different syndromes. Webster’s dictionary defines a syndrome as a group of signs and symptoms that occur together and characterize a particular abnormality (Merriam-Webster 1999). The three different syndromes are the derangement syndrome, the dysfunction syndrome, and the postural syndrome. Each syndrome has unique characteristics that are portrayed differently during the performance of a thorough history and physical examination. The physical examination consists of a series of loading maneuvers that impart stresses to the tissues of the spine, and each syndrome has unique responses to the loading tests. Correct identification of the syndromes will lead the clinician directly to the proper mechanical treatment.
Derangement Syndrome
The derangement syndrome is defined by McKenzie and May as follows: “Internal derangement causes a disturbance in the normal resting position of the affected surfaces. Internal displacement of articular tissue of whatever origin will cause pain to remain constant until such time as the displacement is reduced. Internal displacement of articular tissue obstructs movements” (McKenzie and May 2004, p. 140). Derangement is the most common syndrome seen by the rehabilitation provider, and it relates to the presentation of internal intervertebral disc displacements. The clinical presentation of derangement syndrome may or may not include leg pain in addition to back pain. The patient’s pain changes with induced directional movements as the forces change within the intervertebral disc because of varying positions of the spine. The pain may be present during the movement and at the end range of movement. Sagittal plane range of motion frequently is limited; however, as the derangement is reduced in response to treatment, the range of motion should improve and return to normal. McKenzie further breaks down the derangement syndrome into central symmetric, unilateral asymmetric symptoms to the knee, and unilateral asymmetric symptoms below the knee. Each of the subdivisions of the derangement syndrome has varied and unique principles for intervention. The reader is directed to McKenzie and May’s textbook for further clarification of these sub-classifications (McKenzie and May 2004).
The term centralization is associated with the derangement syndrome and is referred to extensively in the literature on disc herniations (Fig. 8-50). Centralization is the response to therapeutic loading strategies; pain is progressively abolished in a distal to proximal direction with each progressive abolition being retained over time until all symptoms are abolished. If distal or radicular pain is present, successful treatment results in the phenomenon of the pain moving from a widespread to a more central location and eventually being abolished (McKenzie and May 2004).
Dysfunction Syndrome
Dysfunction syndrome is characterized by pain caused by mechanical deformation of structurally impaired tissue and a limited range of motion in the affected direction. The patient reports pain only at the end range of available motion, and when the mechanical load is released, the pain disappears. This syndrome is not common, and a few studies have reported it to be present in fewer than 20% of the patients with lumbar pain treated using the McKenzie approach (McKenzie and May 2004). The dysfunction syndrome may occur in the flexion, extension, or side gliding direction. The dysfunction is named for the direction that is limited, so if flexion is limited, then it would be labeled flexion dysfunction and vice versa for extension (McKenzie and May 2004).
Postural Syndrome
The postural syndrome is characterized by the presence of pain only when normal tissue is deformed over a prolonged period such as by sitting in a slouched posture. This syndrome is seldom seen in isolation, but if abnormal postural loading continues, this deformation of tissue may lead over time to derangement or dysfunction syndrome (McKenzie and May 2004).
Summary of Syndromes
In summary, the derangement syndrome is the most common syndrome and posterolateral derangement of the disc occurs most frequently. The treatment of a derangement is to mechanically load the compromised tissue in the opposite direction of the movement that increases the pain. In a posterolateral derangement the treatment direction is extension of the lumbar spine. This concept may be why many clinicians associate McKenzie treatment only with lumbar extension. Further investigation of the McKenzie approach reveals there is much more than just interventions involving lumbar extension. The derangement syndrome can be compared to a meniscal tear in the knee joint. The tear influences the joint as a mechanical block limiting full function and altering the imposed mechanical forces through the knee, thereby changing the perception of pain. The dysfunction syndrome can be compared to adhesive capsulitis of the glenohumeral joint. It is a soft tissue restriction that limits end-range motion and produces pain at end range. The treatment for a dysfunction is to repeatedly stretch into the direction of limitation. The postural syndrome is treated with postural correction exercises and patient education. See Table 8-13 for a review of McKenzie’s syndromes.
McKenzie Evaluation
The goal of the physical examination is to confirm or refute clinical conclusions drawn initially from the subjective portion of the examination. The movement tests are used to expose the mechanical or nonmechanical nature of the injury and determine a directional preference. The reader is referred to the McKenzie text for exact details regarding testing procedures, positions, and varied responses (McKenzie and May 2004). The movement testing portion of the examination determines three things: (1) the baseline pain, (2) if pain is evident during the movement, and (3) if pain is evident at end range of the movement. On returning to the starting position of the movement, the clinician needs to know how the movement affected the baseline pain. When the patient has baseline pain, potential responses include:
The following patient case illustrates the McKenzie examination and subsequent treatment process. The patient is an 18-year-old baseball catcher, currently unable to practice baseball (catching) for longer than 30 minutes. Batting is unaffected. He reports low-level aching into the lumbar region and buttocks with occasional tingling into the right posterior thigh and knee, which is intermittent but always increases after baseball practice. Playing baseball (catching), driving a car, sitting at school, and doing forward-bending activities make it worse, and walking/movement make it better. He reports that symptoms are getting worse. He reports a history of a lumbar contusion during football season, with pain resolution until attending a baseball catching camp 6 weeks earlier. This case presentation illustrates two possible presentations of low back–related signs and symptoms to demonstrate how a derangement and a dysfunction may initially appear similar but display differences during the mechanical movement testing (Table 8-14).
Additional notes about the case patient’s examination are as follows:
Based on the physical examination chart (see Table 8-14), the patient could respond two different ways. In the dysfunction columns, a flexion dysfunction is indicated by consistent end-range pain with repeated flexion demonstrated both in standing and in lying. The range of motion deficits associated with a dysfunction do not change quickly because repeated stress of the tight soft tissue over a few months time is needed to make gains in motion. Repeated extension will have no effect on the tissue because it is putting slack on the tightened tissue rather than stress on the tissue as occurs with repeated flexion testing. The dysfunction syndrome is analogous to adhesive capsulitis of the shoulder and is painful only at end range of motion, and increasing the extensibility of tissue is a slow process.
Intervention
The various stretches may include:
The patient depicted in this case could easily have an alternate presentation, as noted in the derangement column of Table 8-14. This alternate classification is unilateral asymmetric above the knee derangement. This syndrome would be revealed with repeated trunk flexion, which would have increased and peripheralized the pain, whereas lumbar extension in lying would have decreased and centralized the pain. In a derangement presentation, the direction of preference for treatment is lumbar extension. Some of the exercises appropriate for this patient are:
The dosing of all of these exercises is dependent on the acuity status of the patient and is not discussed here because it is variable. The McKenzie textbook has an excellent section on this topic using a traffic-light analogy to guide in decision making for determining the appropriate intensity of testing movements and of treatment choices (McKenzie and May 2004). Other exercises that are appropriate for this patient may exist, but those presented emphasize the importance of directional preference to abolish signs and symptoms and return to full function.