Chapter 10 Spinal Cord Stimulation for Peripheral Vascular Disease





Establishing Diagnosis
Peripheral vascular disease (PVD) results from progressive atherosclerosis of the arteries of the lower extremities. The presence of PVD signals an increased likelihood of disease in other regions of the body, resulting in cardiovascular and cerebrovascular morbidity and mortality. PVD affects 10% to15% of the U.S. population; the prevalence increases with advancing age.1 Risk factors for PVD include smoking, diabetes, hypertension, dyslipidemia, age greater than 40 years, being of African origin, and previous cardiac and cerebrovascular disease.

The severity of the symptoms in vascular claudication is governed by the amount of stenosis, presence of collateral circulation, and vigor of exercise. The classification systems used to stage severity of disease are the Fontaine and the Rutherford systems, with the Fontaine system used more widely (Table 10-1). Stenoses in the arterial tree secondary to atherothrombosis are responsible for the underlying pathophysiology of this disease. As the disease advances, the resistance in the vascular system increases, and the system is unable to provide oxygenation to the muscles, especially during exercise. This results in claudication, ischemia, ulceration, and eventual loss of limb if left untreated.2 Skeletal muscle ischemia affects muscle metabolism with the accumulation of lactate and intermediates of oxidative metabolism (acylcarnitines). This in turn causes muscle deterioration, denervation, and atrophy.3 Chronic ischemia leads to ulceration and gangrene. Pain resulting from ulcers and gangrene is caused by ischemic neuropathy and necrosis of the sensory nerves at the site of the lesion. Severe necrosis of the sensory nerves can paradoxically make gangrenous lesions insensate and anesthetic.
Stage | Clinical |
---|---|
I | Asymptomatic |
IIa | Mild claudication |
IIb | Moderate-severe claudication |
III | Ischemic rest pain |
IV | Ulceration or gangrene |
Investigations
In the initial stages of PVD clinical history and physical examination are generally unreliable, with the diagnosis being missed more than 90% of the time based on these two factors alone. To make an early diagnosis of PVD, measurement of the ankle-brachial index (ABI) is helpful. The ABI is a ratio of the systolic blood pressure in the ipsilateral dorsalis pedis and posterior tibial arteries to that in the brachial artery (higher of bilateral brachial pressures), measured with a handheld continuous wave Doppler in the supine position. Normal ABI ranges between 1.0 and 1.3. An ABI <0.9 is 95% sensitive and 100% specific for PVD. Angiograms completed in these patients show more than 50% stenosis in one or more major blood vessels.4 The lower the ABI score, the more severe the PVD, with ABI <0.4 representing advanced ischemia. Segmental limb pressure, segmental volume plethysmography, duplex ultrasonography, computed tomography (CT) angiography, and magnetic resonance (MR) angiography are additional modalities for evaluation of the level and extent of disease (Fig. 10-1).
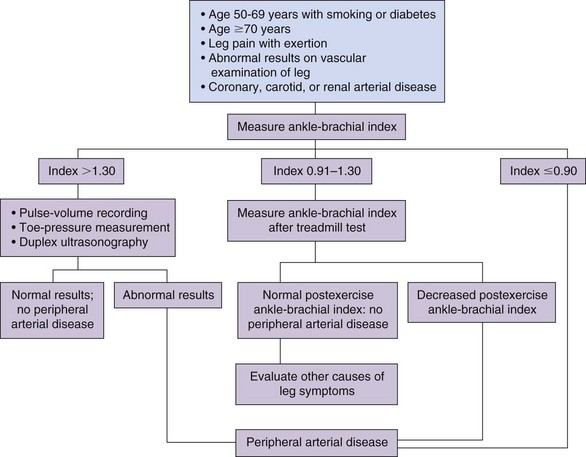
Fig. 10-1 Evaluation of patients with suspected peripheral vascular disease.
Adapted from Hiatt WR et al.: Medical treatment of peripheral arterial disease and claudication, N Engl J Med 344:1608, 2001.
The goals of treatment for patients with PVD are to relieve claudication, improve walking capacity, and improve quality of life. Initial management of PVD includes modification of risk factors such as smoking cessation, glycemic control, blood pressure normalization, and dyslipidemia management. Exercise rehabilitation has also been shown to be beneficial in reducing the symptoms of intermittent claudication by improving collateral circulation and thus improving functional status. Medical treatment of PVD includes antiplatelet and vasodilatory drugs. Revascularization is indicated when ischemic pain is severe, indicated by disabling claudication that prevents the patient from performing daily activities of living, rest pain, ischemic ulcers, or gangrene. Amputation is indicated in approximately 5% of patients with nonreconstructible critical limb ischemia and extensive tissue necrosis or life-threatening infection.5
Spinal cord stimulation (SCS) was first shown to be effective in relieving claudication symptoms and increasing blood flow to lower extremities by Cook and associates6 in 1976, who were using SCS to treat limb pain in patients with multiple sclerosis. During the late 1980s to early 1990s, the use of SCS as an alternative treatment measure for PVD rapidly advanced, especially in Europe. Currently SCS is the most promising neuromodulatory treatment for ischemic pain, and the overall beneficial effects last for at least 1 year in 80% of patients and for up to 5 years in 60% of patients.7 The beneficial effects of SCS in the treatment of ischemic pain include pain relief, ulcer healing, decreased oxygen requirement, and increased claudication distance.
Basic Science
There are no established animal models with PVD that give rise to ischemic pain. Therefore normal Sprague-Dawley rats are used for research into the mechanisms of action of SCS for PVD. These rats are used to study acute changes in peripheral blood flow during SCS. SCS intensity in the rat is determined by the motor threshold (MT), which is the stimulation required for muscle contraction to be observed. Experimental SCS is performed at 30%, 60%, 90%, and 300% of MT. Stimulation at 30% MT is the minimum stimulus that produces vasodilation, with 60% MT being the level that approximates the stimulation parameters in clinical applications in humans. SCS at the upper lumbar spinal segments such as L2-L3 produces the largest increase in cutaneous blood flow in the lower limbs in the rat.8 There are several proposed theories as to the mechanism of action of SCS in the treatment of PVD:


