Chapter 7 Spinal Cord Stimulation
Parameter Selection and Equipment Choices
Parameter Selection
Although spinal cord stimulation at its simplest requires only that a cathode be placed in position over cord with a closely spaced or distant anode to complete the circuit, many other factors may enter into play to change the stimulation experience for the patient. The intrinsic anatomy and physiology of a given patient is something that must be understood and considered.1 The selection of a device and its parameters for stimulation is impactful in patients. Most obvious among decisions of device selection is that of the lead or leads comprising the functional array. Array geometry and lead design have been demonstrated to be powerful in fiber selection for stimulation.2–4 In particular, much of the recent lead development has been in designing arrays and leads to exclude stimulation of certain fibers, specifically the segmental fibers, in an effort to be better able to stimulate those desired within the cord.5 The primary example of this is the ability to stimulate the back area rather than the dorsal roots representing the lower quadrants of the abdomen. Other topographic areas of desired stimulation have necessitated the use of alternate targets, such as the sacral nerve roots in patients with pelvic pain disorders.6,7
Much has been said and written about arrays. However, the parameters of stimulation have had relatively little attention.8 Parameters of stimulation include the frequency or number of pulses per second expressed in Hertz (Hz); pulse width (PW), the duration of each pulse expressed in microseconds; and the amplitude or voltage, representing the power output from the generator. Another factor with potential impact on the experience of the patient is the waveform of each pulse. Although stimulation is done through ramped currents, they are essentially square waves; but the recovery phase of each pulse varies. However, the impact of this variance is less quantifiable because waveforms vary from company to company and may also vary within a given company’s family of devices. Other factors such as constant current vs. constant voltage variances make objective comparisons between one waveform and the next difficult.
To produce paresthesia, Aβ fibers should be stimulated. These lie in the dorsal columns of the spinal cord and have a somatotopic arrangement with the most caudal segments arranged medially, whereas the more cephalad fibers present laterally (Fig. 7-1). Activation of nerve fibers depends on current density, pulse frequency, and specific fiber sensitivity to stimulation. Current density at a target is a function of power output, frequency, and distance to the target. Fiber characteristics that are most impactful to depolarization are size and resting membrane potential and curvature to or from the field.9 All other things being equal, larger fibers depolarize more readily than smaller fibers. Notably within the spinal cord, different tracts have varying sensitivities to current than others.
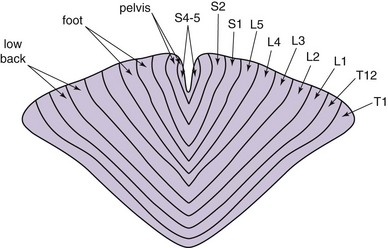
Fig. 7-1 Topographic arrangement of nerve fibers in the dorsal column.
(Adapted from Feirabend HKP et al: Morphometry of human superficial dorsal and dorsolateral column fibres: significance to spinal cord stimulation, Brain 125(5):1137-1149, 2002.)
Because the descending sympathetic tracts (intermediolateral fasciculus) are relatively sensitive to stimulation, it is reasonable to consider that pain relief secondary to stimulation may be related to direct inhibition of these pathways rather than large-fiber afferent activation. It is known that efficacious stimulation in patients treated for angina pectoris may be delivered below sensory perceptual threshold.10