Chapter 4 Spinal cord injury
Incidence and aetiology
Data detailed are taken from the summary in De Vivo (2007). The ratio of male to female cases is approximately 3:1, with greater male preponderance in young age groups. Spinal cord damage can be traumatic or non-traumatic. The main causes of traumatic injury are shown in Figure 4.1. Gunshots and stabbings also make small but increasing contributions (Harrison, 2000; Whalley Hammell, 1995). A significant number of patients with mental health problems will sustain injury from jumping from a height. The level of injury at the time of discharge from hospital is illustrated in Figure 4.2.
The American Spinal Injury Association (ASIA) has produced a classification system for SCI, which is explained below and the classification of injury at discharge is also detailed below (see ‘Diagnosis’).
Non-traumatic aetiology is more common than traumatic. Reported incidence of nontraumatic SCI varies depending on which conditions are included in the study population. Non-traumatic most commonly result from degenerative disc disease and spinal canal stenosis, developmental anomalies (e.g. spina bifida) and congenital anomalies (e.g. angiomatous malformations); inflammation (e.g. multiple sclerosis); ischaemia (e.g. cord stroke); pressure on the cord due to expanding lesions (e.g. abcess or tumour extrinsic or intrinsic to the spinal cord) (New & Sundrajan, 2008). Each condition has distinct management needs and features. Their management will benefit from the knowledge and skills derived from an understanding of traumatic SCI, which is the focus of this chapter.
Terminology
Terms used to describe these patients indicate the general level of the spinal injury listing body functions and structure and a list of domains of activity and participation (International Classification of Functioning, Disability and Health; World Health Organization, 2001).
Types of spinal cord injury
SCI damages a complex neural network involved in transmitting, modifying and coordinating motor, sensory and autonomic control of organ systems. This dysfunction of the spinal cord causes variable loss of homeostatic and adaptive mechanisms which keep people naturally healthy. Any damage to the spinal cord results in deficits that can only be partly predicted, as described below. The pathology of the cord will influence the presenting impairment and the resulting prognosis. It can be precisely correlated with the neurological picture because of the segmental nature of the spinal cord (Kakulas, 2004). Complete transection of the cord is uncommon. It is useful to note that whilst the incidence of SCI in the population as a whole has largely remained the same, the overall prevelence is increasing. There are an increasing number of older people with SCI, reflecting the increasing ageing of the general population, in addition there are more people surviving with SCI into old age (Box 4.1 & Box 4.2).
Over the years there has been a significant reduction in mortality and preservation of neurology in new lesions (Grundy & Swain, 2002; Whalley Hammell, 1995). There are many reasons accounting for this, including:
A previous trend towards increasing incomplete lesions has now lessened. Recent incidence at time of injury has moved closer to equal numbers of incomplete and complete lesions. It is suggested that people with the more serious injuries are surviving, including a higher number of long-term ventilator-dependant patients. It is thought that the accumulative benefits of improved advanced life support and early interventions have now become fully established. It appears that there are no further influences currently increasing the trend towards an incomplete presentation, although the outcomes of new early restorative interventions will be eagerly awaited. There is a significant trend in the reduction in length of stay in the American Model Managed Care Systems, which also reflects a lower Functional Independence Measure (FIM) score at time of discharge and an increase in complications during the first year post discharge (De Vivo, 2007).
Pathogenesis
A brief outline of the pathological changes that occur with SCI is now given; further details can be found in other texts such as Tator (1998).
Later problems
After some weeks, there is evidence of astroglial scarring with cyst formation producing distorted neural architecture. In some cases, months or years later, a syrinx, an expanding cavity within the spinal cord probably associated with disordered cerebrospinal fluid (CSF) flow, may extend rostrally to produce further spinal cord damage. This posttraumatic syringomyelia may require drainage by shunt to prevent further extension. In view of this possibility, the neurological status should be reassessed periodically (Illis, 1988) and appropriate magnetic resonance imaging (MRI) scanning performed at intervals to minimize further neurological loss.
Spinal cord plasticity
When peripheral nerve is damaged, repair can lead to significant return of function (Battiston et al., 2009; Dahlin, 2008). It has been demonstrated that the central nervous system (CNS) has the capacity to regenerate and recover. It has similarly been hypothesized that there is capacity within the spinal cord to regenerate through a number of mechanisms. Research is ongoing to identify axonal budding, unmasking and interspinal spinal circuits (central pattern generators). For further reading on neuroplasticity see Chapter 11, Adkins et al. (2006), Kleim (2009), and Schwartz and Begley (2003). A summary of research aiming to establish new treatments in the management of spinal cord damage is discussed briefly below.
Treatment approaches in immediate post-injury management
There are four main approaches currently being considered to develop treatments for SCI (Ronsyn et al., 2008):
Understanding axonal guidance systems that will be required for directed outgrowth and functional reconnection will be essential if useful functional activity is to be regained. Although much progress has been made in the laboratory setting, no techniques applied to humans, despite having been through well researched trials (Fawcett et al., 2007; Lammertse et al., 2007; Steeves et al., 2007; Tuszynski et al., 2007) have yet to emerge showing any consistent results (Johnston, 2001; Ramer et al., 2000; Ronsyn et al., 2008; Tator, 1998).Claims for successful regeneration of the chronically injured spinal cord through late repair, remain contentious. Most studies lack robust pre- and post-intervention measures with many appearing to rely on associated intensive rehabilitation to demonstrate small functional improvements.
Diagnosis
Incomplete versus complete injury classification
ASIA Impairment Scale
In terms of diagnosis and prognosis, the classification of SCI has important ramifications. The ASIA Impairment Scale (ASIA, 2008) is the latest updated criteria for assessing and classifying functional levels of SCI, including the definitions of complete and incomplete lesions. The assessment is completed with the patient in supine, to enable testing in the acutely unstable injured person. The assessment comprises of 10 key myotomes and 28 dermatomes (Figure 4.3). Each dermatome is tested for light touch and pin prick sensation. The full description for these classifications will not be detailed here and it is available at www.asia-spinalinjury.org, with the impairment scale and classification outlined in Figure 4.4.
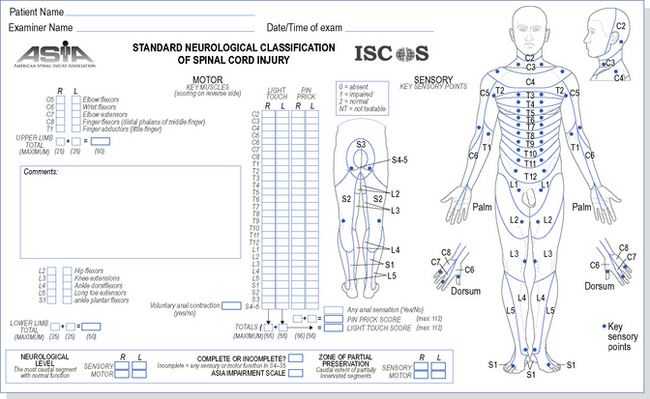
Figure 4.3 The American Spinal Injury Association (ASIA) Dermatome Chart and Impairment Scale.
American Spinal Injury Association: International Standards for Neurological Classification of Spinal Cord Injury, reprint 2008; Chicage, Il. Reprinted with permission.
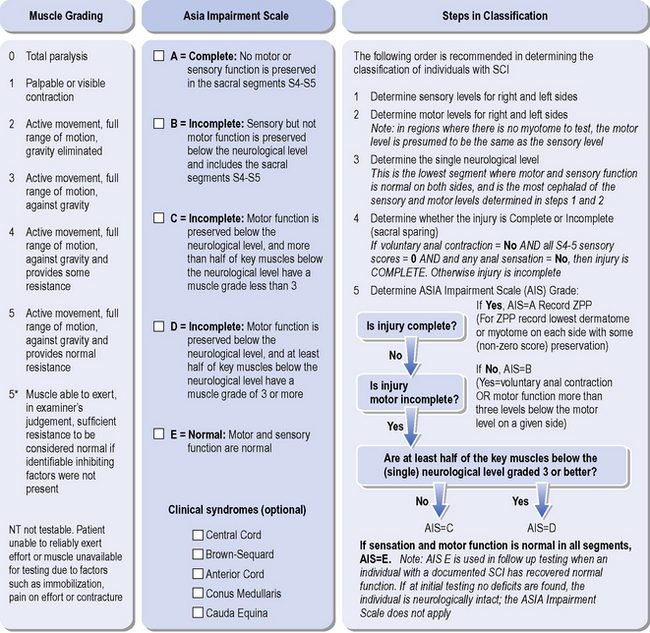
Figure 4.4 ASIA classification guide.
American Spinal Injury Association: International Standards for Neurological Classification of Spinal Cord Injury, reprint 2008; Chicage, Il. Reprinted with permission.
The ASIA system defines that a patient can have neurological sparing below the injury level, but in the absence of the sacral sparing, this is classified as a complete lesion, ASIA A, with zones of partial preservation. Where there is sensory preservation of S4–S5, the patient is classified sensory incomplete, ASIA B. This implies the preservation of the long tracts through the lesion. The classification of incomplete versus complete lesions indicates the presence of sensation in the lower sacral segments S4-S5, which implies significant prognostic indication of potential for neurological improvement. To be classified ASIA C, the patient must be assessed to have preserved S4-S5 sensation and voluntary anal sphincter motor activity. If the voluntary anal activity is absent then there must be preservation of motor function in some muscles innervated more than three levels below the motor classification level. In addition, more than half the key muscles below the neurological level must be grade 1-2/5. Similarly if half the key muscles are grade 3-5/5 then the classification will be ASIA D. The classification of SCI on discharge from hospital is shown in Figure 4.5.
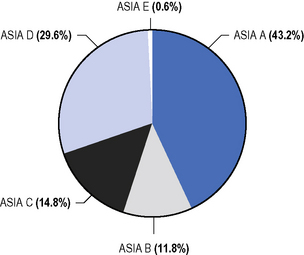
Figure 4.5 American Spinal Injury Association (ASIA) classification at discharge from hospital.
American Spinal Injury Association: International Standards for Neurological Classification of Spinal Cord Injury, reprint 2008; Chicage, Il. Reprinted with permission.
From a prognostic point of view, research suggests that 72 hours post injury (Brown, 1994; Maynard et al., 1979), and 1 month post injury are good time points for this classification (Waters et al., 1994a, 1994b). Further assessment is advised at 3 months post injury. All patients are assessed immediately on hospital admission, to gain baseline data. The wealth of prognostic statistics is based on data obtained using these time periods.
Incomplete lesions and prognostic indicators
There are recognized patterns of incomplete cord injury which tend to present clinically as combinations of syndromes rather than in isolation. The signs and symptoms are related to the anatomical areas of the cord affected (Figures 4.6 & 4.7). Clinically, patterns of incomplete lesions are referred to as a syndrome.
Recent statistics show the common patterns of incomplete lesions to be the following:
Anterior cord syndrome
Anterior cord syndrome describes the effects of ventral cord damage affecting spinothalamic and corticospinal tracts; there is complete motor loss caudal to the lesion, and loss of pain and temperature sensation as these sensory tracts are located anterolaterally in the spinal cord. Preservation of the posterior columns means that perception of vibration and proprioception on the ipsilateral side are intact. This syndrome can arise from anterior spinal artery embolization. Motor recovery is thought to be less in these patients in comparison with other incomplete lesions (Crozier et al, 1991; Foo, 1986).
Brown–Séquard syndrome
Originally described by Galen, this syndrome describes sagittal hemicord damage with ipsilateral (same side) paralysis and dorsal column interruption, leading to loss of proprioception, in addition to contralateral (opposite side) loss of temperature and pain sensation. The relatively normal pain and temperature sensation on the ipsilateral side is due to the spinothalamic tract crossing over to the opposite side of the cord, at the level they enter the cord. This hemisection injury of the cord is classically caused by stabbing. This syndrome has a favourable prognosis, with almost all patients ambulating successfully (Johnston, 2001). The theory for this is that, despite the loss of pinprick on the one side of the cord, axons in the contralateral cord may facilitate recovery (Little & Habur, 1985).
Central cord lesion
The upper-limbs are more profoundly affected than the lower limbs and the condition is typically seen in older patients with cervical spondylosis. Due to degenerative changes in the spinal column, there are osteophytes and possible disc bulges, combined with spondylitic joint changes in the anterior part of the vertebral column. Posteriorly, the ligamentum flavum is thickened. A hyperextension injury compresses the cord in the narrowed canal and leads to interference of the blood supply. This may be already compromised in an older person and so has less potential for recovery. The central cervical tracts are predominantly affected. There is often flaccid weakness of the arms, due to lower motor neurone (LMN) lesions, and spastic patterning in the arms and legs due to upper motor neurone (UMN) injury. Bowel and bladder dysfunctions are common, but only partial. Research findings vary, showing that 57–86% patients will ambulate, although 97% of younger patients less than 50 years ambulate compared with 41% over 50 years (Foo, 1986).
Prognosis
Recovery of the incomplete spinal cord injury
Ninety per cent of incomplete SCI patients have some recovery of a motor level in their upper-limbs, compared to 70–85% of the complete injuries (Ditunno et al., 2000). Pinprick sparing in a dermatome is an excellent indicator of increased recovery of motor strength (Poynton et al., 1997) and it has been found that pinprick preservation below the level of the injury to the sacral dermatomes is the best indicator of useful recovery, with 75% of patients regaining the ability to walk. Fifty per cent of patients who had no sacral sparing regained some motor recovery but not of functional use (Katoh & El Masry, 1995).
Studies have found that incomplete SCI patients showed ongoing improvement in their motor activity, although this tended to slow during the second year post injury, with the exception of incomplete tetraplegics who lacked sharp/blunt discrimination and failed to demonstrate any lower-limb motor recovery. In incomplete paraplegics, there was evidence of 85% of the muscles recovering from a flicker to an antigravity grade within the first year, but if there was no activity initially, only 26% gained an antigravity grade (Waters et al., 1994a, 1994b).
Ambulation recovery
Between 44 and 76% of people with incomplete SCI, with preserved sensation but no motor function, have been reported to achieve ambulation (Maynard et al., 1979; Waters et al., 1994a, 1994b).
Crozier et al. (1991) reported, using ASIA assessment at 72 hours, that 89% of ASIA B–E patients with pinprick preservation went on to ambulate, compared with 11% having preserved light touch but not pinprick.
Summary of changes in ASIA impairment scale from admission to discharge
Acute general management
Trauma management
Immediate management
Acute hospital management
Acute trauma management guidelines are well established (Moore et al., 1991). Management of the patient with an SCI has special features resulting from spinal cord shock. Full details are described by Grundy and Swain (2002).
Breathing
Paralysis of respiratory muscles may be a feature. Patients with acute cervical cord injuries can fatigue in their breathing. Pulse oximetry is a crude indicator of respiratory distress because it measures only haemoglobin saturation and not partial pressure of oxygen (Po2) (Hough, 2001). Any evidence of desaturation or of falling saturation should be proactively addressed by the critical care team to maintain oxygenation and prevent further cord damage. Monitoring patients’ breathing rate, pattern and colour, and noting agitation, drowsiness or distressed behaviour, is vital. Arterial blood-gas analysis may be the critical factor in deciding whether to provide ventilatory support. Respiratory failure remains one of the main causes of death in acute tetraplegia, whilst pneumonia is the leading cause of death in all persons with SCI (Jackson et al., 1994). The principles of respiratory management are covered in Chapter 15.
Spinal cord shock
This is the phenomenon of cessation of nervous system function below the level of damage to the cord and may be due to the loss of descending neural influences. It is usually expected that after several seconds to months, the flaccid paralysis and areflexia of spinal shock are replaced by hyperexcitability, seen clinically as hyperreflexia, spasticity and spasms. More recently this has been identified as a critical period when the timing of potential interventions can influence recovering neurological systems. Studies have shown that there is competetive synaptic growth into the synaptic spaces that have been vacated. These transient vacant sites become open to repopulation by spared axons. It is at this moment that interventions should ideally target synapse growth mediating voluntary movement rather than local segmental neurons mediating spasticity and hyperreflexia (Ditunno et al., 2004; McDonald et al., 2002).
Stauffer (1983) noted that it is rare to see patients in total spinal shock and totally areflexic. Strong spasticity almost immediately post injury is indicative of an incomplete SCI. In these patients assessment of voluntary movement requires careful differentiation. In the authors’ experience, development of increased muscle tone and involuntary movements may mislead patients to believe they have functional return of activity. It is important for the therapist to anticipate such reactions and to assess carefully in order to avoid confusion and disappointment.
Spinal stabilization versus conservative management
Spinal fractures may be classified as stable, unstable or quasistable (i.e. currently stable but likely to become unstable in the course of everyday activity). Disagreement continues between protagonists and antagonists of surgical stabilization of the spine, but surgery is increasingly used (Collins, 1995).
Definition of instability or stability of a spinal lesion has now achieved substantial agreement based on the three column principles (Dennis, 1983). There is general agreement that restoration of the anatomy of the canal is sensible in terms of giving the cord the best opportunity for recovery.
It is debated whether neurological recovery or degree of spinal stability in the long term differs with surgical or conservative management. Surgery aims to minimize neurological deterioration, restore alignment and stabilization, facilitate early mobilization, reduce pain, minimize hospital stay and prevent secondary complications (Johnston, 2001). Review of evidence over the last decade does not identify any specific timing or role for early surgical decompression. Surgical intervention within the first 72 hours after injury has been shown to be safe and a role for urgent decompression has been identified in certain circumstances and may improve neurological outcomes (Fehlings & Perrin, 2006).
Management of acute lesions at T4 and above
Surgical stabilization may be achieved by anterior or posterior fixation, or a combination of the two (e.g. Collins, 1995). Patients managed conservatively are immobilized with bed rest; depending on the degree of instability, they may have to be maintained in spinal alignment by skull traction. Traction is applied usually by halo traction, Gardner–Wells or cone calipers (Grundy & Swain, 2002).
Early mobilization may be indicated and can be achieved using a halo brace (Hossain et al., 2004). Care in handling and positioning during physiotherapy is discussed below (see ‘Acute physical management’).
Special problems in spinal cord injury
Osteoporosis
Osteoporosis is a loss in bone mass without any alteration of the ratio between mineral and the organic matrix. A text by (Jiang et al., 2006) provides a comprehensive overview of osteoporosis. It is thought that immobilization for long periods and a sedentary life lead to an increase in bone reabsorption, thus causing osteoporosis.
Rapid loss of bone minerals occurs during the first 4 months following SCI. A range of data available from studies include: at 1 year bone mass density reduces in the femoral neck by 27%, mid femoral shaft by 25% and distal femur by 43%. The reduction continues in the pelvis and lower limbs over the 10 years after injury and can reduce by 50%. Tetraplegics can lose up to 16% on their bone mass density in their upper limbs. The osteoporosis may cause fractures of long bones during relatively simple manoeuvres, such as transfer or passive movements (Belanger et al., 2000). The rate of incidence of fractures has been documented to be 1% in the first year post injury, 1.3% per year at 1–9 years, 3.4% per year at 10–19 years, 4.6% per year at 20–29 years (Jiang et al., 2006).
It has been shown that early mobilization with weight bearing might prevent or slow bone-mineral loss (De Bruin et al., 1999). Bone mineral density can be preserved in bones below the level of the lesion (Frey-Rindova et al., 2000). The question is frequently asked whether a patient who has not stood for several years should recommence standing. There is a variety of opinion on how to proceed, either returning straight to standing or commencing a weight-bearing programme using a tilt table, usually combined with bone-enhancing agents, and monitoring using bone densitometry, before returning to standing in a frame. Such advice is empirical and, as yet, despite many studies, no clear guidance has been produced.
Heterotopic ossification
Calcification in denervated or UMN-disordered muscle remains an ill-understood process and commonly occurs in patients with SCI (David et al., 1993). It may be confused in the early stages with deep venous thrombosis, when it presents as swelling, alteration in skin colour and increased heat, usually in relation to a joint. During the active process, analysis of plasma biochemistry shows a raised alkaline phosphatase. It can result in loss of range of movement (ROM) and difficulty in sitting. If ossification occurs around the hips it may lead to further skin pressure problems. Treatment of this condition is discussed by David et al. (1993). It must be emphasized that stretching should be gentle, as overstretching may be a predisposing factor for this condition.
The bladder
Urological complications of SCI are major mortal and morbid risks and the reader is referred to Fowler and Fowler (1993) for a review. Spinal cord damage disrupts the neural controls of bladder function. The objectives of bladder management are to provide a system ensuring safety, continence and least social disruption.
Fertility
Fertility is usually maintained in women, with the ovulatory cycle being normal within 9 months after injury. Fertility in men is, however, a problem (Brindley, 1984). Improvements in fertility rates for men after SCI have been made due to several important technical advances. These include improved methods in the retrieval and enhancement of sperm, such as electroejaculation, and improved means of achieving fertilization with limited sperm quality and numbers through in vitro techniques.