Chapter 8 Spinal Cord and Nerve Roots
The spinal cord provides innervation for the trunk and limbs through the paired spinal nerves and their peripheral ramifications. Through them it receives primary afferent fibres from peripheral receptors located in widespread somatic and visceral structures. It also sends motor axons to skeletal muscle and provides autonomic innervation of cardiac and smooth muscle and secretory glands. Many functions are regulated by intraspinal reflex connections. Profuse ascending and descending pathways link the spinal cord with the brain. They allow higher centres to monitor and perceive external and internal stimuli and modulate and control spinal efferent activity.
The spinal cord is essentially a segmental structure. It gives rise to 31 pairs of segmentally arranged spinal nerves, which are attached to the cord by a linear series of dorsal and ventral rootlets. Dorsal rootlets contain afferent nerve fibres, and ventral rootlets contain efferent fibres (see Fig. 1.5). Groups of adjacent rootlets coalesce to form dorsal or ventral nerve roots. These cross the subarachnoid space and unite to form functionally mixed spinal nerves as they pass through the intervertebral foramina. The dorsal roots bear dorsal root ganglia, which contain the cell bodies of primary afferent neurones.
Topographical Anatomy of the Spinal Cord
Macroscopic Anatomy of the Spinal Cord and Spinal Nerves
The gross anatomy of the structures that lie within the vertebral canal and its extensions through the intervertebral foramina, the spinal nerve or radicular (‘root’) canals is the subject of this chapter. The spinal cord, its blood vessels and nerve roots lie within a meningeal sheath, the theca, which occupies the central zone of the vertebral canal and extends from the foramen magnum, where it is in continuity with the meningeal coverings of the brain, to the level of the second sacral vertebra in the adult. Distal to this level the dura extends as a fine cord, the filum terminale externum, which fuses with the posterior periosteum of the first coccygeal segment. Tubular prolongations of the dural sheath extend around the spinal roots and nerves into the lateral zones of the vertebral canal and out into the ‘root’ canals, eventually fusing with the epineurium of the spinal nerves. Between the theca and the walls of the vertebral canal is the epidural (spinal extradural) space (Ch. 4), which is loosely filled with fat, connective tissue containing small arteries and lymphatics and an important venous plexus. Three-dimensional appreciation of the anatomy of the spinal theca and its surroundings is essential for the efficient management of spinal pain, spinal injuries, tumours and infections. Equally significant clinically is the anatomy of the often precarious blood supply of the spinal cord and its associated structures. The increasing application and refinement of diagnostic imaging and endoscopic procedures lend new importance to topographical detail here.
Spinal Cord (Medulla)
The spinal cord is an elongated, approximately cylindrical part of the central nervous system, occupying the superior two-thirds of the vertebral canal (Figs 8.1–8.6). Its average length in European males is 45 cm; its weight is approximately 30 g (for dimensional data, consult Barson and Sands 1977). It extends from the upper border of the atlas to the junction between the first and second lumbar vertebrae; this lower level varies, and there is some correlation with the length of the trunk, especially in females. The termination may be as high as the caudal third of the twelfth thoracic vertebra or as low as the disc between the second and third lumbar vertebrae, and its position rises slightly in vertebral flexion. The spinal cord is enclosed in the dura, arachnoid and pia mater, separated from each other by the subdural and subarachnoid spaces, respectively. The former is a potential space, whereas the latter contains cerebrospinal fluid (CSF). The cord is continuous cranially with the medulla oblongata and narrows caudally to the conus medullaris, from whose apex a connective tissue filament, the filum terminale, descends to the dorsum of the first coccygeal vertebral segment. The spinal cord varies in transverse width, gradually tapering craniocaudally, except at the levels of the cervical and lumbosacral enlargements. It is not cylindrical, being wider transversely at all levels, especially in the cervical segments.
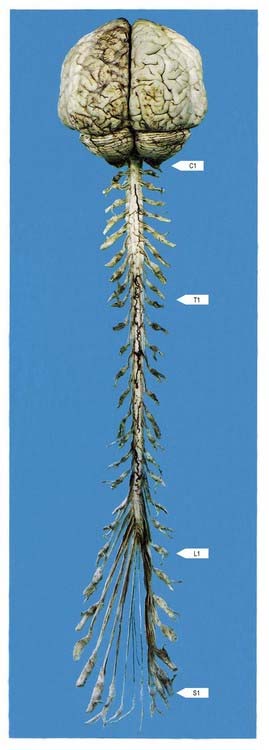
Fig. 8.3 Brain and spinal cord with attached spinal nerve roots and dorsal root ganglia, photographed from the dorsal aspect. Note the fusiform cervical and lumbar enlargements of the cord and the changing obliquity of the spinal nerve roots as the cord is descended. The cauda equina is undisturbed on the right but has been spread out on the left to show its individual components.
(Dissection by M. C. E. Hutchinson, GKT School of Medicine; photograph by Kevin Fitzpatrick on behalf of GKT School of Medicine, London.)
A posterolateral sulcus exists from 1.5 to 2.5 mm lateral to each side of the posterior median sulcus. Dorsal roots (strictly rootlets) of spinal nerves enter the cord along this sulcus. The white substance between the posterior median and posterolateral sulcus on each side is the posterior funiculus. In cervical and upper thoracic segments, a longitudinal posterointermediate sulcus marks a septum dividing each posterior funiculus into two large tracts: the fasciculus gracilis (medial) and the fasciculus cuneatus (lateral). Between the posterolateral sulcus and anterior median fissure is the anterolateral funiculus. This is subdivided into anterior and lateral funiculi by ventral spinal roots that pass through its substance to issue from the surface of the cord. The anterior funiculus is medial to, and includes, the emerging ventral roots; the lateral funiculus lies between the roots and the posterolateral sulcus. In upper cervical segments, nerve rootlets emerge through each lateral funiculus to form the spinal accessory nerve (cranial nerve XI), which ascends in the vertebral canal lateral to the spinal cord and enters the posterior cranial fossa via the foramen magnum (Fig. 8.7).
Dorsal and Ventral Roots
The paired dorsal and ventral roots of the spinal nerves are continuous with the spinal cord (see Figs 8.6 and 8.10). They cross the subarachnoid space and traverse the dura mater separately, uniting in or close to their intervertebral foramina to form the (mixed) spinal nerves. Because the spinal cord is shorter than the vertebral column, the more caudal spinal roots descend for varying distances around and beyond the cord to reach their corresponding foramina. In so doing they form, mostly distal to the apex of the cord, a divergent sheaf of spinal nerve roots, the cauda equina, which is gathered around the filum terminale in the spinal theca.
Meninges
Dura Mater
The single layer of dura that lines the cranial cavity divides into two layers as it passes downward through the foramen magnum, although it is still a single layer as it forms the anterior and posterior atlanto-occipital membranes. Within the vertebral column, it has been suggested that the outer endosteal layer becomes the periosteum of the vertebral canal, which is separated from the spinal dura mater by an extradural (epidural) space (see later). This interpretation, which would make the epidural space ‘intradural,’ is not generally agreed on (Newell 1999). The spinal dura mater forms a tube whose upper end is attached to the edge of the foramen magnum and to the posterior surfaces of the second and third cervical vertebral bodies, as well as to the posterior longitudinal ligament by fibrous bands, especially toward the caudal end of the vertebral canal. The dural tube narrows at the lower border of the second sacral vertebra. It invests the thin spinal filum terminale, descends to the back of the coccyx, and blends with the periosteum. The meningeal coverings of the spinal roots and nerves are described later in this chapter.
Epidural Space
The epidural space lies between the spinal dura mater and the tissues that line the vertebral canal (Fig. 8.8). It is closed above by fusion of the spinal dura with the edge of the foramen magnum, and below by the posterior sacrococcygeal ligament, which closes the sacral hiatus. It contains loosely packed connective tissue, fat, a venous plexus, small arterial branches, lymphatics and fine fibrous bands that connect the theca with the lining tissue of the vertebral canal. These bands, the meningovertebral ligaments, are best developed anteriorly and laterally. Similar bands tether the nerve root sheaths or ‘sleeves’ within their canals. There is also a midline attachment from the posterior spinal dura to the ligamentum nuchae at the atlanto-occipital and atlanto-axial levels (Dean and Mitchell 2002). The venous plexus consists of longitudinally arranged chains of vessels connected by circumdural venous ‘rings.’ The vertebral venous plexus is commonly known as Batson’s plexus. The anteriorly placed vessels receive the basivertebral veins.
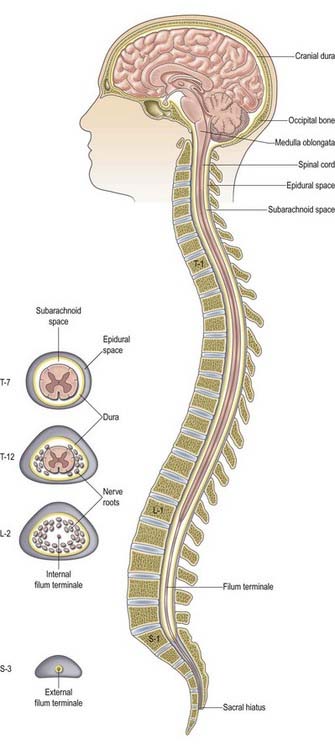
Fig. 8.8 Epidural space.
(Adapted with permission from Rosse, C., Gaddum-Rosse, P., 1997. Hollinshead’s Textbook of Anatomy, 5th ed. Lippincott-Raven, Philadelphia, Fig. 13-3.)
The shape of the space within each spinal segment is not uniform, although the segmental pattern is metamerically repeated. It is difficult to define the true shape of the ‘space’ because it changes with the introduction of fluid or as a result of preservation techniques. In the lumbar region, the dura mater is apposed to the walls of the vertebral canal anteriorly and attached by connective tissue in a manner that permits displacement of the dural sac during movement and venous engorgement. Adipose tissue is present posteriorly in recesses between the ligamentum flavum and the dura. The connective tissue extends for a short distance through the intervertebral foramina along the sheaths of the spinal nerves. Like the main thecal sac, the root sheaths are partially tethered to the walls of the foramina by fine meningovertebral ligaments. Contrast media and other fluids injected into the epidural space at the sacral level can spread up to the cranial base. Local anaesthetics injected near the spinal nerves, just outside the intervertebral foramina, may spread up or down the epidural space to affect the adjacent spinal nerves or may pass to the opposite side. The paravertebral spaces of each side communicate via the epidural space, particularly at the lumbar levels.
Subdural Space
The subdural space is a potential space in the normal spine because the arachnoid and dura are closely apposed (Haines, Harkey and Al-Mefty 1993). It does not connect with the subarachnoid space but continues for a short distance along the cranial and spinal nerves. Accidental subdural catheterization may occur during extradural injections. Injection of fluid into the subdural space may damage the cord either by direct toxic effects or by compression of the vasculature.
Arachnoid Mater
The arachnoid mater that surrounds the spinal cord is continuous with the cranial arachnoid mater (Figs 8.9, 8.10). It is closely applied to the deep aspect of the dura mater. At sites where vessels and nerves enter or leave the subarachnoid space, the arachnoid mater is reflected on to the surface of these structures and forms a thin coating of leptomeningeal cells over the surface of both vessels and nerves. Thus, a subarachnoid angle is formed as nerves pass through the dura into the intervertebral foramina. At this point, the layers of leptomeninges fuse and become continuous with the perineurium. The epineurium is in continuity with the dura. Such an arrangement seals the subarachnoid space so that particulate matter does not pass directly from the subarachnoid space into nerves. The existence of a pathway of lymphatic drainage from the CSF is controversial.
Pia Mater
The spinal pia mater closely invests the surface of the spinal cord and passes into the anterior median fissure (see Figs 8.9, 8.10). As in the cranial region, there is a subpial ‘space’; however, over the surface of the spinal cord the subpial collagenous layer is thicker than in the cerebral region, and it is continuous with the collagenous core of the ligamentum denticulatum.
The ligamentum denticulatum is a flat, fibrous sheet that lies on each side of the spinal cord between the ventral and dorsal spinal roots. Its medial border is continuous with the subpial connective tissue of the cord, and its lateral border forms a series of triangular processes, the apices of which are fixed at intervals to the dura mater. There are usually 21 processes on each side. The first crosses behind the vertebral artery, where it is attached to the dura mater, and is separated by the artery from the first cervical ventral root. Its site of attachment to the dura mater is above the rim of the foramen magnum, just behind the hypoglossal nerve; the spinal accessory nerve ascends on its posterior aspect (see Fig. 8.7). The last of the dentate ligaments lies between the exiting twelfth thoracic and first lumbar spinal nerves and is a narrow, oblique band that descends laterally from the conus medullaris. Changes in the form and position of the dentate ligaments during spinal movements have been demonstrated by cineradiography.
Beyond the conus medullaris, the pia mater continues as a coating of the filum terminale.
Intermediate Layer
In addition to the well-defined coats of arachnoid and pia mater, the cord is surrounded by an extensive intermediate layer of leptomeninges (see Fig. 8.10). This layer is concentrated in the dorsal and ventral regions and forms a highly perforated, almost lace-like structure that is focally compacted to form the dorsal, dorsolateral and ventral ligaments of the spinal cord. Dorsally, the intermediate layer is adherent to the deep aspect of the arachnoid mater and forms a discontinuous series of dorsal ligaments that attach the spinal cord to the arachnoid. The dorsolateral ligaments are more delicate and fenestrated, and they extend from the dorsal roots to the parietal arachnoid. As the intermediate layer spreads laterally over the dorsal surface of the dorsal roots, it becomes increasingly perforated and eventually disappears. A similar arrangement is seen over the ventral aspect of the spinal cord, but the intermediate layer is less substantial.
Spinal Nerves
In those body segments that largely retain a metameric (segmental) structure (e.g. the thoracic region), spinal nerves exhibit a common plan (Fig. 8.11). The dorsal, epaxial ramus passes back, lateral to the articular processes, and divides into medial and lateral branches that penetrate the deeper muscles of the back; both branches innervate the adjacent muscles and supply a band of skin from the posterior median line to the scapular line. The ventral, hypaxial ramus is connected to a corresponding sympathetic ganglion by white and grey rami communicantes. It innervates the prevertebral muscles and curves around in the body wall to supply the lateral muscles of the trunk. Near the mid-axillary line it gives off a lateral branch that pierces the muscles and divides into anterior and posterior cutaneous branches. The main nerve advances in the body wall, where it supplies the ventral muscles, and terminates in branches to the skin.
Spinal Roots and Ganglia
Dorsal (Posterior) Roots
Little is known about the regions of entry and emergence of afferent and efferent rootlets in humans, but these zones of transition between the central and peripheral nervous systems have been extensively described in rodents (Fraher 2000).
Appearance and Orientation of Roots at Each Spinal Level
The size and direction of spinal nerve roots vary. The upper four cervical roots are small; the lower four are large. The thickness ratio of cervical dorsal roots to ventral roots is 3 : 1, which is greater than in the other regions. The first dorsal root is an exception, being smaller than the ventral root, and it is occasionally absent. The conventional view is that the first and second cervical spinal roots are short, running almost horizontally to their exits from the vertebral canal, and from the third to eighth cervical levels, the roots slope obliquely down. Obliquity and length increase successively, although the distance between spinal attachment and vertebral exit never exceeds the height of one vertebra. An alternative view is that upper cervical roots descend, the fifth is horizontal, the sixth to eighth ascend, the first two thoracic roots are horizontal, the next three ascend, the sixth is horizontal and the rest descend (Kubik and Müntener 1969). This view is based on the observation that the cervicothoracic part of the spinal cord grows more in length than the other parts do.
Lower lumbar and upper sacral roots are the largest, and their rootlets are most numerous. Coccygeal roots are the smallest. Kubik and Müntener (1969) confirm that lumbar, sacral and coccygeal roots descend with increasing obliquity to their exits. The spinal cord ends near the lower border of the first lumbar vertebra, and the lengths of successive roots rapidly increase; the consequent collection of roots is the cauda equina (see Fig. 8.3). The largest roots, and hence the largest spinal nerves, are continuous with the spinal cervical and lumbar swellings and innervate the upper and lower limbs.
Spinal Ganglia (Dorsal Root Ganglia)
Spinal ganglia are large groups of neurones on the dorsal spinal roots. Each is oval and reddish; its size is related to that of its root. A ganglion is bifid medially where the two fascicles of the dorsal root emerge to enter the cord. Ganglia are usually located in the intervertebral foramina, immediately lateral to the perforation of the dura mater by the roots (see Fig. 8.4). However, the first and second cervical ganglia lie on the vertebral arches of the atlas and axis, the sacral lies inside the vertebral canal, and the coccygeal ganglion usually lies within the dura mater. The first cervical ganglion may be absent. Small aberrant ganglia sometimes occur on the upper cervical dorsal roots between the spinal ganglia and the cord.
Spinal Nerves Proper
Immediately distal to the spinal ganglia, ventral and dorsal roots unite to form spinal nerves (Figs 8.11, 8.12). These soon divide into dorsal and ventral rami, both of which receive fibres from both roots. At all levels above the sacral, this division occurs within the intervertebral foramen. Division of the sacral spinal nerves occurs within the sacral vertebral canal, and the dorsal and ventral rami exit separately through posterior and anterior sacral foramina at each level. Spinal nerves trifurcate at some cervical and thoracic levels, and the third branch is called a ramus intermedius. At or distal to its origin, each ventral ramus gives off recurrent meningeal (sinuvertebral) branches and receives a grey ramus communicans from the corresponding sympathetic ganglion. The thoracic and first and second lumbar ventral rami each contributes a white ramus communicans to the corresponding sympathetic ganglia. The second, third and fourth sacral nerves also supply visceral branches, unconnected with sympathetic ganglia, that carry a parasympathetic outflow directly to the pelvic plexuses.
Coverings and Relations of the Spinal Roots and Nerves in the Radicular Canal
Tubular prolongations of the spinal dura mater, closely lined by the arachnoid, extend around the spinal roots and nerves as they pass through the lateral zone of the vertebral canal and through the intervertebral foramina (Figs 8.9, 8.10, 8.13). These prolongations, the spinal nerve sheaths (root sheaths), gradually lengthen as the spinal roots become increasingly oblique. Each individual dorsal and ventral root runs in the subarachnoid space with its own covering of pia mater. Each root pierces the dura separately, taking a sleeve of arachnoid with it, before joining within the dural prolongation just distal to the spinal ganglion. The dural sheaths of the spinal nerves fuse with the epineurium, within or slightly beyond the intervertebral foramina. The arachnoid prolongations within the sheaths do not extend as far distally as their dural coverings, but the subarachnoid space and its contained CSF extend sufficiently distally to form a radiologically demonstrable ‘root sleeve’ for each nerve. Shortening or obstruction of this sleeve seen on myelography indicates compression of the spinal nerve. At the cervical level, where the nerves are short and the vertebral movement is greatest, the dural sheaths are tethered to the periosteum of the adjacent transverse processes. In the lumbosacral region there is less tethering of the dura to the periosteum, although there may be an attachment posteriorly to the facet joint capsule.
Functional Components of Spinal Nerves
Each typical spinal nerve contains somatic and visceral (autonomic) fibres.
Variations of Spinal Roots and Nerves
The courses of spinal roots and nerves in relation to the thecal sac and vertebral and radicular canals may be aberrant. An individual intervertebral foramen may contain a duplicated sheath, nerve and roots, which are then absent at an adjacent level. Abnormal communications between roots may occur within the vertebral canal. These anomalies have been described and classified for the lumbosacral spine by Neidre and Macnab (1983).
Rami of the Spinal Nerves
Dorsal (posterior primary) rami of spinal nerves are usually smaller than the ventral rami and are directed posteriorly. Retaining a segmental distribution, they all (except for the first cervical, fourth and fifth sacral and coccygeal) divide into medial and lateral branches that supply the muscles and skin of the posterior regions of the neck and trunk (Fig. 8.14).
Cervical dorsal spinal rami
The first cervical dorsal ramus, the suboccipital nerve (see Fig. 8.7), is larger than the ventral. It emerges superior to the posterior arch of the atlas and inferior to the vertebral artery and enters the suboccipital triangle to supply the rectus capitis posterior major and minor, obliquus capitis superior and inferior and semispinalis capitis. A filament from the branch to the inferior oblique joins the second dorsal ramus. The suboccipital nerve occasionally has a cutaneous branch that accompanies the occipital artery to the scalp and connects with the greater and lesser occipital nerves. It may also communicate with the accessory nerve.
The second cervical dorsal ramus is slightly larger than the ventral and all the other cervical dorsal rami (see Figs 8.7, 11.10B). It emerges between the posterior arch of the atlas and the lamina of the axis, below the inferior oblique, which it supplies. It receives a connection from the first cervical dorsal ramus and divides into a large medial and smaller lateral branch. The medial branch, termed the ‘greater occipital nerve’, ascends between the inferior oblique and semispinalis capitis, pierces the latter and trapezius near their occipital attachments and is joined by a filament from the medial branch of the third dorsal ramus. It ascends with the occipital artery, divides into branches that connect with the lesser occipital nerve and supplies the skin of the scalp as far forward as the vertex. It supplies the semispinalis capitis and, occasionally, the back of the auricle. The lateral branch supplies the splenius capitis, longissimus capitis and semispinalis capitis and is often joined by the corresponding third cervical branch.
Dermatomes
The cutaneous area supplied by one spinal nerve, through both rami, is a dermatome (Fig. 8.15). Typically, dermatomes extend around the body from the posterior to the anterior median line. The upper half of each zone is supplemented by the nerve above, and the lower half by the nerve below. The area supplied by dorsal rami is limited laterally by the dorsolateral line, which descends laterally from the occiput to the medial end of the acromion, continues to the posterior aspect of the greater trochanter and curves medially to the coccyx. Cutaneous strips supplied by dorsal rami do not correspond exactly to those served by ventral rami and differ in both breadth and position. Dermatomes of adjacent spinal nerves overlap markedly, particularly in the segments least affected by development of the limbs (i.e. second thoracic to first lumbar). In some regions (e.g. upper anterior thoracic wall), cutaneous nerves supplying adjoining areas are not from consecutive spinal nerves, and the overlap is minimal. When the second thoracic spinal ramus is severed, anaesthesia is sharply demarcated, but some overlap for awareness of painful and thermal stimuli may exist. Likewise, after section of a peripheral nerve (e.g. ulnar nerve at the wrist), the area of tactile loss is always greater than that of lost pain and temperature sensation. Hence, the area of total anaesthesia and analgesia following section of peripheral nerves is always less than might be anticipated from their anatomical distribution.
Internal Organization
In transverse section, the spinal cord is incompletely divided into symmetric halves by a dorsal (posterior) median septum and a ventral (anterior) median sulcus (see Fig. 8.5). It consists of an outer layer of white matter and an inner core of grey matter. The dimensions and relative volumes of white matter and centrally aggregated neurone cell bodies vary according to the level (Fig. 8.16). The amount of grey matter at any level is a function of the amount of muscle, skin and other tissues innervated by neurones at that level. It is therefore largest by proportion in the cervical and lumbar enlargements, because neurones in these segments of the cord innervate the limbs; it is attenuated at thoracic levels. The absolute amount of white matter is greatest at cervical levels and decreases progressively at lower levels; this is the case because descending tracts shed fibres as they descend, and ascending tracts accumulate fibres as they ascend.
Spinal Grey Matter
In three dimensions, the spinal grey matter is shaped like a fluted column (see Fig. 8.6). In transverse section (see Fig. 8.16) the column is often described as being ‘butterfly shaped’ or resembling the letter ‘H.’ It consists of four cellular masses, referred to as the dorsal and ventral horns (or columns), which project dorsolaterally and ventrolaterally toward the surface. The grey matter that immediately surrounds the central canal and unites the two sides is termed the dorsal and ventral grey commissure. The dorsal horns are the site of termination of primary afferent fibres, which enter via the dorsal roots of spinal nerves. The tip of the dorsal horn is separated from the surface of the cord by a thin dorsolateral tract (tract of Lissauer) in which primary afferents ascend and descend for a short distance before terminating in the subjacent grey matter (Figs 8.17, 8.18). The dorsal horn may be described in terms of a head, neck and base, the individual constituents of which are described in more detail later. The ventral horns contain efferent neurones whose axons leave the spinal cord in ventral nerve roots. A small intermediate lateral horn is present at the thoracic and upper lumbar levels and contains the cell bodies of preganglionic sympathetic neurones.
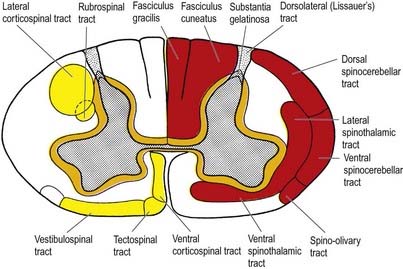
Fig. 8.17 Simplified diagram of the main tracts of the spinal cord. The ascending tracts are shown in red on the right side of the figure; the descending tracts are shown in yellow on the left side; the ‘intersegmental’ tracts are shown in orange on both sides. Many tracts are omitted (see Fig. 8.18).
Spinal grey matter is a complex mixture of neuronal cell bodies (somata), their processes (neurites) and synaptic connections, neuroglia and blood vessels (Fig. 8.19). Neurones in the grey matter are multipolar and vary in size and other features, particularly the length and arrangement of their axons and dendrites. Many are Golgi types I and II neurones. Axons of Golgi type I neurones pass out of the grey matter into ventral spinal roots or spinal tracts. Axons and dendrites of Golgi type II neurones are largely confined to the nearby grey matter. The distribution of neurones may be intrasegmental—deployed within a single segment—or intersegmental—spread through several segments.