7 Special Topics
Running injuries: etiology and recovery-based treatment
Allan Besselink, PT, Dip MDT, and Bridget Clark, PT, MSPT, DPT
However, running also displays a trend toward a significant rate of injury. The current literature indicates various injury rates, depending on the study. Koplan et al. (1982) reported that 60% of all runners will sustain an injury within any given year that is severe enough to force them to alter their training. It has also been reported that the yearly incidence injury rate for runners training for a marathon is as high as 90%. Given that the average runner will have 800 to 2000 footstrikes per mile, the opportunity for injury to occur is significant. Running injuries are not limited to any one joint or anatomic region (Table 7-1), although a large percentage of injuries tend to occur at the knee.
Anatomic Region | Percentage of Injuries |
---|---|
Knee | 7.2–50.0 |
Shin, Achilles tendon, calf, heel | 9–32.0 |
Foot and toes | 5.7–39.0 |
Hamstring, quadriceps | 3.4–38.0 |
Data from van Gent RN, Siem D, van Middelkoop M, van Os AG, Bierma-Zeinstra SM, Koes BW. Incidence and determinants of lower extremity running injuries in long distance runners: A systematic review. Br J Sports Med 2007;41:469–480.
Gait: Walking and Running
The gait cycle has been defined by Thordarson (1997) as the period from initial contact of one foot until the initial contact of that same foot. A brief review of the gait cycle will provide some background on the nature of mechanical loading and the neuromuscular requirements of both walking and running.
Running Mechanics
The running gait cycle (Fig. 7-1) is also divided into a stance phase and a swing phase. The stance phase may involve an initial foot contact which takes place as a heel strike, midfoot strike, or forefoot strike. Initial foot contact exists on a continuum with increasing gait speed, progressing from heel strike in walking to forefoot strike in sprinting. The percentage of the gait cycle spent in the stance phase varies depending on gait speed—60% with walking, 40% with running, and just 22% with world class sprinters. The walking gait cycle is distinct in that it involves a period of double limb support in which both of the feet are on the ground. The running gait cycle is distinct in that it involves a period of double float in which both of the feet are off the ground. The progression from walking gait to running gait is a continuum—from double limb support in walking to double float period in running.
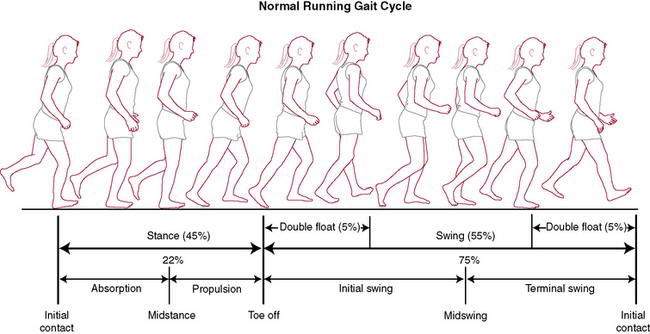
Figure 7-1 Normal running gait cycle.
(Redrawn from Mann RA, Coughlin MJ. Surgery of the Foot and Ankle, 6th ed. St. Louis: Mosby, 1993.)
At a certain walking speed, there is a transition from walking to running gait which occurs in order to maintain biomechanical, metabolic, and aerobic efficiency (Fig. 7-2). The speed at which this transition occurs varies between individuals, although it tends to be at or near a velocity of 12:00 per mile (5.0 mph) for most. This becomes an important issue when 70% of the running population runs at a pace of 10:00 per mile or slower. Though fast walking and slow jogging have a similar cardiovascular response, slow jogging creates ground reaction forces and loading rates as much as 65% greater than fast walking (Table 7-2). The progression from walking to running involves certain requirements from the body including the ability to tolerate increased mechanical loads (i.e., ground reaction forces) and the strength not only to progress the body forward concentrically, but also to eccentrically control the stance leg. Running and sprinting require more power and range of motion at the hip, knee, and ankle as speed is increased.
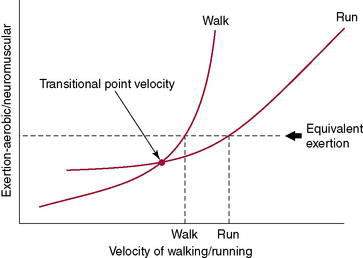
Figure 7-2 Transition from walking to running.
(Redrawn from Besselink A. RunSmart: A Comprehensive Approach to Injury-Free Running, Morrisville, 2008, Lulu Press.)
Table 7-2 Ground Reaction Forces Associated with Walking and Running at Various Speeds
Running Speed | Pace (Per Mile) | Vertical Ground Reaction Force (Body Weight) |
---|---|---|
1.5 m/s–1 (3.4 mph) (walk) | 17:53/mile | 1.1–1.5 |
2.5 to 3.0 m/s–1 (5.6–6.7 mph) (slow jog) | 8:56–10:44/mile | 2.5 |
5.0 to 8 m/s–1 (11.2–17.9 mph) (run) | 3:21–5:22/mile, or 0:50–1:20/quarter | 2.5–2.88 |
Data adapted from Keller TS, Weisberger AM, Ray JL, Hasan SS, Shiavi RG, Spengler DM. Relationship between vertical ground reaction force and speed during walking, slow jogging, and running. Clin Biomech 1996;11: 253–259 and Munro CF, Miller DI, Fuglevand AJ. Ground reaction forces in running: A reexamination. J Biomech 1987;20:147–155.
Ground reaction forces appear to increase linearly up to a gait speed of 60% of maximum speed (average of 4.0m/s−1), but at higher speeds, ground reaction forces appear to stay at approximately 2.5–2.8 times body weight (Table 7-2). It is also noteworthy that during running, athletes that heel strike upon initial contact have a higher initial peak in vertical ground reaction force than midfoot strikers. There is a strong relationship between impact peak and loading rate. The loading rate associated with running has been found to be positively correlated with running velocity, finding an average rate of 77 BW/s−1 (body weight) at slower speeds of 3.0m/s−1, increasing to 113 BW/s−1 at faster speeds of 5.0 m/s−1.
The anterior and posterior calf muscles, quadriceps, hip extensors, and hamstrings all work eccentrically during the stance phase. Of note is the function of the quadriceps, which is the primary shock absorber, absorbing 3.5 times as much energy as it produces. After the initial ground reaction forces are attenuated, the foot then supinates during the propulsion phase to provide a more rigid lever for push off. Winter (1983) noted that the gastrocneminus generates the primary propulsive force during the propulsion phase of running and produces forces between 800–1500 W, compared to 150 W for slow walking and 500 W for fast walking.
Causes of Running Injuries
Review of the current scientific research does in fact yield a definitive answer. One primary factor has been directly associated with the onset of running injury—training or errors in training. James et al. (1978) noted that the primary etiology in two thirds of all causes of injury can be directly related to “training error.” Lysholm and Wiklander (1987) reported that training errors alone, or in combination with other factors, were implicated in injuries in 72% of runners. Simply stated, training error is most often an issue of “too much, too soon,” the importance of which is explained later.
Contrary to the commonly held beliefs of the medical and running communities, there is not any specific correlation between anatomic malalignment or variations in the lower extremity and any specific pathologic entities or predisposition to any “overuse” syndromes. In fact, Reid (1992) noted that “normal variations in the human body abound, and only a few percent of the population are actually good examples of ‘normal.’… Furthermore, all of these variations are found in world class athletes and seem to produce little adverse effect on their ability to perform their sports.… [T]he corollary of this enormous variation of body build among enthusiastic amateur and the professional athletes is that there is a poor correlation of specific malalignments with specific conditions.” Table 7-3 summarizes the sport sciences literature regarding the factors that have been noted to have a direct association with running injury and those that either have no direct association or do not presently have scientific evidence to support an association with running injuries.
Table 7-3 Evidence-Based Factors Associated and Not Associated with Running Injuries
Factors Having a Direct Association with Injury | Factors That Do Not Have Evidence for Association with Injury | Factors Known to Not Have a Direct Association with Injury |
---|---|---|
“Training error” (most often too much, too soon) Running distance History of prior injury Previous competition in running events |
Warmup and stretching exercises Body height Malalignment Muscular imbalance Decreased range of motion Running frequency Level of performance (current skill level) Stability of running shoes Running on one side of the road Orthotics |
Gender Age Body mass index Running on hard surfaces Running hills Participation in other sports Time of year Time of day |
Data from van Mechelen W. Running injuries. A review of the epidemiological literature. Sports Med 1992;14:320–335.
Training error is the only factor that consistently displays a cause–effect relationship with running injuries. Reid (1992) has gone so far as to state that “every running injury should be viewed as a failure of training technique, even if other contributing factors are subsequently identified.” In addition, running distance of more than 25 to 40 miles per week, previous competition in running events, and a history of prior injury have been found to be strongly associated with running injuries.
Under-Recovery Not Overuse
For years, the health care community has pointed to the “overuse” running injury, but if “overuse” were the problem, then there would be a preset threshold at which point all runners would get injured—and this simply is not the case. Physiologic causes of running injuries can be explained by Wolfe’s law. The body aims to attain homeostasis at the cellular level. As a stimulus is applied to tissues (including bone, tendon, muscle, ligament, and collagen-based tissues), a cellular response is triggered and, over time and with sufficient recovery, an adaptation occurs. This adaptation could be greater tissue integrity, strength, or similar mechanical response. Tissues adapt to mechanical loading if given an environment in which to do so and sufficient metabolic capacity to allow this to occur (Fig. 7-3). This has been shown repeatedly with studies on astronauts and deep sea divers, two populations that face altered repeated and/or sustained mechanical loads. There is a precise balance between stimulus and response—or, for the athlete, the application of a training stimulus and the recovery and adaptation to this stimulus. With this in mind, “overuse” injuries should be more accurately described as “under–recovery” injuries because, given appropriate time for recovery, adaptation to the stimulus will take place successfully.
Figure 7-3 illustrates the body’s ability to recover from and adapt to a single training stimulus. Figures 7-4 and 7-5 display the effect of several training stimuli: Figure 7-4 with appropriate and sufficient recovery and Figure 7-5 with insufficient recovery and poor training adaptation. Injuries occur when the rate of application of training stimulus exceeds the rate of recovery and adaptation.
Mechanical Assessment
Subjective
A thorough examination should begin with a review of the patient’s prior running program. We have compiled a list of characteristic traits of the run training program that typically contribute to factors related to overuse/under-recovery (Table 7-4). This assists the clinician’s understanding of the athlete’s current capacity to tolerate mechanical loading. The intent and rationale for each question has also been provided.
Running Experience | Intent/Rationale of Question |
1. Have you been involved in any other sport or fitness activities, and if so, for how long? | General level of conditioning and tissue “health” and current loading capacity. |
2. How long have you been a runner? | More experienced runners tend toward lower injury risk. |
3. Have you had any previous running injuries? If so, where and when? | Injury risk increases if history of a prior running injury. |
Current Training Program | Intent/Rationale of Question |
1. How many days per week do you run? | Number of recovery days per week. |
2. How many miles do you run per week? | Most programs emphasize “more is better”; injury risk tends to increase at 25–40 miles per week |
3. What is your average running pace (minutes/mile)? | Running mechanics change with running pace. |
4. What was your longest run in the month prior to injury? | The rate of progression of the total volume of training and loading capacity. |
5. Do you recall any change in your running program that occurred just prior to the onset of your injury? | Injured runners most typically have some type of sudden change in the volume of their training; the rate of application of training stimuli exceeds the rate of adaptation to training. |
6. Are you training with a group or individually? Are you using a published program or a coach? | Access to the program itself can be valuable for further analysis by the clinician (see #5). |
7. What is the longest run that you have done since you noted the injury? How long ago was this done? | Allows the clinician to better understand where to resume running when the athlete is ready (i.e., longer break = more gradual resumption of training). |
8. Do you compete in races? If so, what distance(s)? Are you currently training for a particular event? | Injury risk is higher in those who have competed in the past. If they are currently training for an event, it may affect their rate of progression and return to running, along with their overall goal setting. |
9. Do you do interval training (speed work) in your training program? If so, what and how often? | Is the athlete doing any run training activities that are building power and loading capacity? |
10. Do you do strength and/or plyometric training as part of your training program? If so, what exercises are you doing? Typical number of sets and repetitions? Light, moderate, or heavy resistance? Number of days per week? | Strength and plyometric training (high load, low repetitions) build greater loading capacity and power output. |
11. Is there anything else you would like to tell me about your running program? | It is common that the athlete will have an inherent “sense” of the factors that contributed to the injury. Ask them! |
Objective
The McKenzie method of Mechanical Diagnosis and Therapy, or MDT™ (The McKenzie Institute, Syracuse, NY), forms the basis of the mechanical assessment and is presented here because it is a comprehensive classification and treatment system that has scientific research to support not only its assessment process, but also its classification algorithm. Although MDT™ initially gained widespread international acceptance for the treatment of spinal pain, its principles also are readily applied to the extremities. Three primary aspects are unique to the McKenzie method™—mechanical assessment, self-treatment, and prevention (Table 7-5). Although a complete description of the McKenzie method™ is not within the scope of this chapter, further resources can be found in the reference list at the end of this chapter.
Table 7-5 Basic Concepts of Mechanical Diagnosis and Therapy™
Mechanical Assessment |
1. Establish a relationship between symptom response and mechanical loading (typically via repeated test movements).
. |
.
.
Education
Education of the patient is a critical element in the effective treatment of the injured runner.
As the patient recovers from injury and returns to running, the physical therapist thoroughly reviews the progression back to running to prevent reinjury (see Table 7-4). Runners, like most athletes, are eager to return to athletic training and competition. Because running injuries are generally training related, it is imperative that athletes understand how to modify their training to foster injury recovery and tissue repair, how to prepare their body to accept the increasing mechanical loads with running, and how to optimize their performance. Most runners are under the mindset that “more is better.” Because research clearly dictates otherwise for runners, it is imperative to educate the patient.