Chapter 12 Special Senses
The special senses of olfaction, vision, taste, hearing and balance are conveyed to the brain in cranial nerves. In each case, highly specialized peripheral receptors respond to stimuli in the external environment or our relationship to it. The olfactory system has an ancient lineage, reflected by the fact that afferent olfactory pathways proceed directly to the cerebral cortex and bypass the thalamus. Its terminal fields are, likewise, primitive cortical areas in a phylogenetic sense and are considered to be parts of the limbic system. All other special senses have a thalamic representation that projects to specialized regions of the neocortex. The integrative functions related to the various special senses (e.g. control of ocular gaze) are also included here. Of particular importance is a detailed discussion of retinal functional anatomy.
Olfaction
Olfactory pathways subserving the sense of smell are described in this section. Details of the relationship between the olfactory pathways and the limbic system are shown in Figure 16.7.
There is a clear laminar structure in the olfactory bulb (Fig. 12.1). From the surface inward, the laminae are the olfactory nerve layer, glomerular layer, external plexiform layer, mitral cell layer, internal plexiform layer and granule cell layer.
The olfactory tract leaves the posterior pole of the olfactory bulb to run along the olfactory sulcus on the orbital surface of the frontal lobe (see Fig. 16.7). The granule cell layer of the bulb is extended into the olfactory tract as scattered medium-sized multipolar neurones that constitute the anterior olfactory nucleus. They continue into the olfactory striae and trigone to the grey matter of the prepiriform cortex, anterior perforated substance and precommissural septal areas. Many centripetal axons from mitral and tufted cells relay in, or give collaterals to, the anterior olfactory nucleus; the axons from the nucleus continue with the remaining direct fibres from the bulb into the olfactory striae.
As the olfactory tract approaches the anterior perforated substance, it flattens and splays as the olfactory trigone. Fibres of the tract continue from the caudal angles of the trigone as diverging medial and lateral olfactory striae, which border the anterior perforated substance. An intermediate stria sometimes passes from the centre of the trigone to end in a small olfactory tubercle. The lateral olfactory stria follows the anterolateral margin of the anterior perforated substance to the limen insulae, where it bends posteromedially to merge with an elevated region, the gyrus semilunaris, at the rostral margin of the uncus in the temporal lobe (see Fig. 16.7). The lateral olfactory gyrus forms a tenuous grey layer covering the lateral olfactory stria; it merges laterally with the gyrus ambiens, part of the limen insulae. The lateral olfactory gyrus and gyrus ambiens form the prepiriform region of the cortex, passing caudally into the entorhinal area of the parahippocampal gyrus. The prepiriform and periamygdaloid regions and the entorhinal area (area 28) together make up the piriform cortex. The medial olfactory stria, covered thinly by the grey matter of the medial olfactory gyrus, passes medially along the rostral boundary of the anterior perforated substance toward the medial continuation of the diagonal band of Broca. Together, they curve up on the medial aspect of the hemisphere, anterior to the attachment of the lamina terminalis. The diagonal band enters the paraterminal gyrus. The medial stria becomes indistinct as it approaches the boundary zone, which includes the paraterminal gyrus, parolfactory gyrus and, between them, prehippocampal rudiment (see Fig. 16.7).
Vision
Eye
The eyeball, the peripheral organ of vision, is situated in the orbit, a skeletal cavity whose walls help protect the eye from injury (Fig. 12.2). The orbit also has a more fundamental role in the visual process itself: it provides rigid support and direction to the eye and forms the sites of attachment for its external muscles. This setting permits the accurate positioning of the visual axis under neuromuscular control and determines the spatial relationship between the two eyes—essential for binocular vision and conjugate eye movements.
Visual Pathway
The visual pathway is illustrated in Figure 12.3. The first-order neurone of the visual system is a bipolar cell that is contained entirely within the retina. The second-order neurone is a ganglion cell whose axon enters the optic nerve.
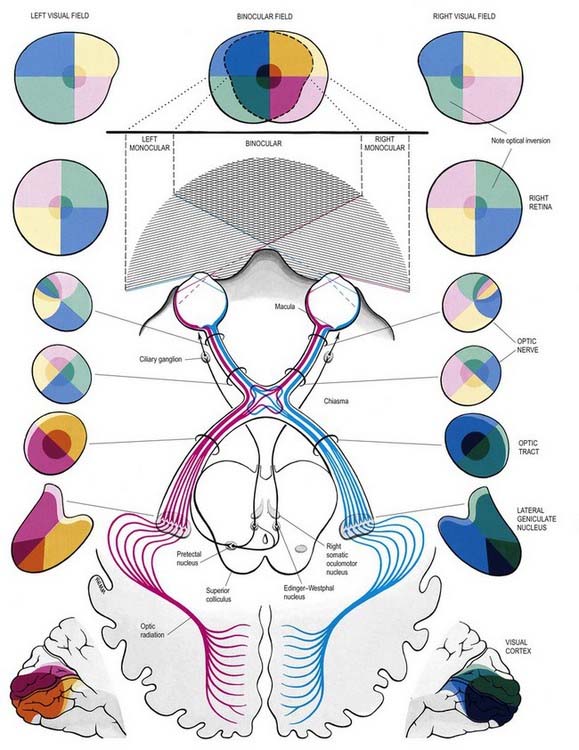
Fig. 12.3 The visual pathway, showing the spatial arrangement of neurones and their fibres in relation to the quadrants of the retinae and visual fields. The proportions at various levels are not exactly to scale; in particular, the macula is exaggerated in size in the visual fields and retinae. In each quadrant of the visual field and the parts of the visual pathway subserving it, two shades of the respective colour are used—paler for the peripheral fields, and darker for the macular part of the quadrant. From the optic tract onward, these two shades are both more saturated to denote the intermixture of neurones from both retinae, with the palest shade reserved for parts of the visual pathway concerned with monocular vision. The pathway subserving the pupillary light reflex is also indicated.
Axons from third-order visual neurones in the lateral geniculate nucleus run in the retrolenticular part of the internal capsule and form the optic radiation, which curves dorsomedially to the occipital cortex. Fibres representing the lower half of the visual field sweep superiorly to reach the visual cortex above the calcarine sulcus. Those representing the upper half of the visual field curve inferiorly into the temporal lobe (Meyer’s loop) before reaching the visual cortex below the calcarine sulcus.
Retina
The retina is the sensory neural layer of the eyeball (Figs 12.4–12.6). It is a very complex structure and should be considered a special area of the brain, from which it is derived by outgrowth from the diencephalon (Ch. 15). It is dedicated to the detection and early analysis of visual information and is an integrated part of the much larger apparatus of visual analysis present in the thalamus, cortex and other areas of the central nervous system.
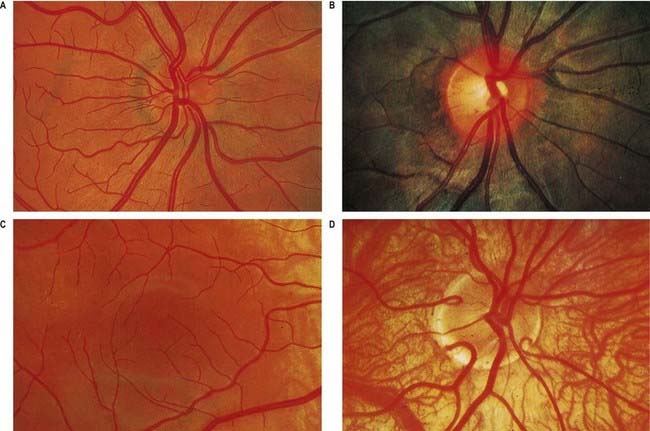
Fig. 12.4 Ophthalmoscopic photographs of the right human retina. A, Note the dichotomous branching of vessels. Arteries are brighter red and show a more pronounced ‘reflex’ to light, as a pale stria along their length. The veins are also larger in calibre; more of them cross arteries superficially than is usual. The optic disc, around the entry of the vessels, is a light pink, with a surrounding zone of heavier pigmentation. Compare with Figure 12.5A from the same Caucasian adult. B, Appearances in a heavily pigmented individual (an adult of African origin), with a paler optic disc than in A. Note accentuation of the edge of the disc by retinal and choroidal pigmentation. The arteries cross the veins superficially in this retina. C, Normal macula of a young Caucasian subject. The vessels radiate from the centrally placed fovea. The macular branches of the central retinal artery are approaching from the right. The macula is largely free of vessels of macroscopic size, but the capillaries here form a particularly close network, except at the fovea. D, The region of the optic disc in an eye with poorly developed pigmentation. Three cilioretinal arteries are curving around the edge of the disc (two on the left, one on the right). Between the two cilioretinal arteries, a single macular artery is apparent. Due to the depressed pigmentation, choroidal vessels are also visible, especially veins; on the left of the photograph, two large vorticose venous tributaries can be seen.
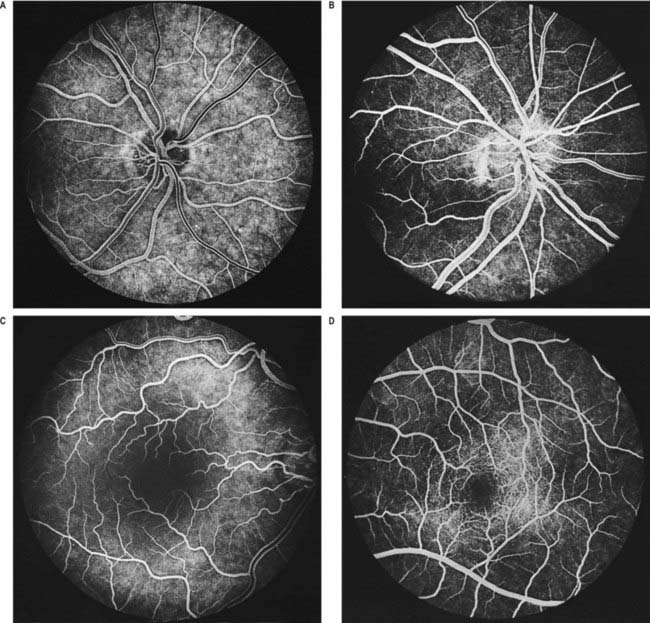
Fig. 12.5 Fluorescence angiograms of the retina. These are produced by photography with a fundus camera at known periods of time following the introduction of fluorescein into the circulation. A, Angiogram of the same retina shown in Figure 12.4A, taken in ‘mid-venous’ phase. The arteries display an even fluorescence, but the veins appear striped, owing to laminar flow. This appearance is the reverse of the arterial ‘reflex’ shown in Figure 12.4A and should not be compared with it. The background mottling is due to fluorescence from the choroidal vessels. B, Angiogram of the left optic disc, showing the major arteries and veins and also their smaller branches. Note particularly the radial pattern in the retinal capillaries. The laminar flow in the veins is less obvious than in Figure 12.4A. C, Angiogram showing the macular region of a right eye. The main macular vessels are approaching from the right. The subject is an elderly person with considerable macular pigmentation, which masks fluorescence from the choroidal circulation. D, Angiogram of the macula of a young subject (left eye) showing the macular capillaries in detail. Note the central avascular fovea. Compare with C.
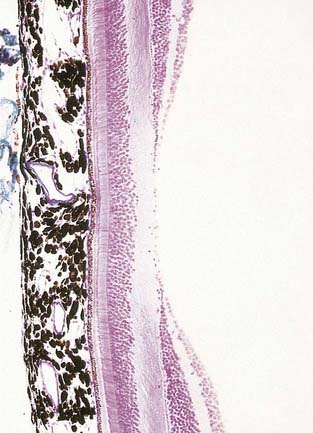
Fig. 12.6 Section through the fovea centralis.
(By permission from Young, B., Heath, J.W., 2000. Wheater’s Functional Histology, Churchill Livingstone, Edinburgh.)
Near the centre of the retina, there is a region 5 to 6 mm in diameter that contains the macula lutea (see Fig. 12.5C, D), an elliptical yellowish area measuring approximately 2 mm horizontally and 1 mm vertically. Its colour is due to the presence of xanthophyll derivatives. The macula lutea contains a central depression, the fovea centralis or foveola, with a diameter of approximately 0.4 mm, where visual resolution is highest (see Fig. 12.6.) Here, all elements except pigment epithelium cone photoreceptors are displaced laterally. The minute size of the foveola is the reason why the visual axes must be directed with great accuracy to achieve the most discriminative vision.
About 3 mm medial (nasal) and 1 mm superior to the foveola, the optic nerve becomes continuous with the retina at the optic disc (‘blind spot’). It is approximately 1.5 mm in diameter. The name ‘optic papilla,’ which is often applied to the disc, is a misnomer because almost all of a normal disc is level with the retina. Centrally, it contains a shallow depression, where it is pierced by the central retinal vessels (see Figs 12.2, 12.4, 12.5A, B). The disc is devoid of photoreceptors and is therefore insensitive to light. By ophthalmoscopy, the disc is normally pink, but it is much paler than the retina and may be grey or almost white. In optic atrophy the capillary vessels disappear, and the disc is then white.
Layers of the Retina
The retina is organized into layers or zones (Fig. 12.7), where distinctive components of its cells are clustered together or in register to form continuous strata. These layers extend uninterrupted throughout the photoreceptive retina except at the exit point of the optic nerve fibres at the optic disc, although certain layers are much reduced at the foveola where the photoreceptive elements predominate. The names given to the different layers reflect, in part, the components present within them, and also their position in the thickness of the retina. Conventionally, those structures farthest from the vitreous (i.e. toward the choroid) are designated as outer or external, and those toward the vitreous are inner or internal.
Customarily, 10 retinal layers are distinguished (Fig. 12.8), beginning at the choroidal edge and passing toward the vitreous. These are the retinal pigment epithelium, layer of rods and cones (outer and inner segments), external limiting membrane, outer nuclear layer, outer plexiform layer, inner nuclear layer, inner plexiform layer, ganglion cell layer, nerve fibre layer, and internal limiting membrane. Some of these are subdivided into substrata, and an innermost plexiform layer between layers 8 and 9 has also been demonstrated.
Rod and cone cells reach radially inward from the rod and cone lamina through the outer nuclear layer, where they have their nuclei, to the outer plexiform layer, where they synapse with bipolar and horizontal cells. Bipolar cells possess dendrites in the outer plexiform layer, cell bodies and nuclei in the inner nuclear layer, and axons in the inner plexiform layer, where they synapse with ganglion cell and amacrine cell dendrites. Horizontal cells have their dendrites and axons in the outer plexiform layer and their nuclei in the inner nuclear layer; ganglion cells have their dendrites in the inner plexiform layer, their cell bodies in the ganglion cell layer and their axons in the layer of nerve fibres (and within the optic nerve). Amacrine cell dendrites are mainly in the inner plexiform layer, although some (interplexiform cells) extend into the outer plexiform layer; amacrine cell dendrites are situated in either the inner nuclear layer or the outer part of the ganglionic layer (displaced amacrines). Pigment cells lie behind the retina, and several types of retinal glial cell are distributed in distinctive locations among its different layers.
The composition of the retinal layers is as follows:
Layer 7: Inner Plexiform Layer
This is divisible into three layers, depending on the types of contact that occur. The outer or ‘off’ layer contains synapses between ‘off’ bipolar cells, ganglion cells and some amacrines; a middle or ‘on’ layer contains synapses between the axons of ‘on’ bipolars and the dendrites of ganglion cells and displaced amacrines; and an inner ‘rod’ layer contains synapses between rod bipolars and displaced amacrines. (Refer to Wässle and Boycott 1991 for an explanation of the ‘off’ and ‘on’ cell designations.)