Sleep and sleep pharmacology
After reading this chapter, the reader will be able to:
1. Define terms that pertain to sleep and sleep pharmacology
2. Describe sleep, its individual stages, and their electrophysiologic correlates
3. Comprehend the basic neurophysiologic mechanisms that promote brain arousal and wakefulness
4. Comprehend the basic neurophysiologic mechanisms that promote sleep onset and maintenance
5. Describe basic circadian processes and their interaction with the sleep-wake cycle
6. Recognize several sleep disorders that are amenable to pharmacotherapy
7. Describe the rationale for using certain classes of drugs to treat specific sleep-related disorders
Compounds whose parent structure is uric acid. These compounds depress central nervous system activity. Long-acting barbiturates such as pentobarbital have been used to treat epilepsy. Barbital was used during the early 20th century to facilitate sleep in individuals with insomnia.
Compounds whose parent structure is a fusion of a diazepine ring with a benzene ring. Benzodiazepines enhance activity of the inhibitory neurotransmitter γ-aminobutyric acid (GABA). Benzodiazepines, which reduce anxiety and promote muscle relaxation, also promote sleep. The earliest benzodiazepines were chlordiazepoxide (Librium) and diazepam (Valium). Benzodiazepines for insomnia are now being replaced by nonbenzodiazepines such as zolpidem (Ambien), eszopiclone (Lunesta), and others.
Circadian rhythms “Circa” is Latin for “about,” and “diem
” is Latin for “day.” Circadian rhythms refer to the approximately 24-hour cycle of biochemical, physiologic, and behavioral processes.
Measurement and recording of the gross electrical activity of the brain. During EEG recordings, electrodes are typically placed across multiple scalp regions. The electrodes are connected to amplifiers and filters that detect, magnify, and record the electrical activity of the brain.
Presence of excessive sleepiness. Daytime sleepiness is so great that it leads to inappropriate daytime napping or sleep. Excessive sleepiness is not alleviated by prolonged sleep times or by napping.
Class of drugs used to induce sleep.
Group of sleep disorders manifested by undesirable motor, sensory, or behavioral phenomena that occur during sleep. The International Classification of Sleep Disorders, Revised (ICSD-R) lists 24 parasomnias. More commonly encountered parasomnias include confusional arousals, sleep terrors, and sleepwalking.
Measurement and recording of EEG activity, typically coupled with measurement and recording of cardiorespiratory activity and eye movements, during sleep.
History of treatment of sleep disorders
Humans spend about a third of their life sleeping, and sleep disorders, which affect a large proportion of the general population and occur in all age groups, represent a major public health and global economic burden.1 It is estimated that 50 million to 70 million adults in the United States have a chronic sleep disorder that interferes with their daily functioning and adversely affects their health and quality of life. Until the mid-1960s, the field of sleep medicine focused primarily on describing and treating insomnia, parasomnias (e.g., sleep walking, night terrors) and hypersomnias, such as narcolepsy. Patients experiencing these symptoms typically sought consultations from neurologists, psychiatrists, psychologists, or their family physician. Until more recently, treatments were generally based on empiric pharmaceutical intervention and behavioral modification protocols or psychotherapy or both.
It is beyond the scope of this chapter to address the entire spectrum of sleep disorders classified in the International Classification of Sleep Disorders, Revised (ICSD-R) or to provide an exhaustive summary of all the pharmacologic, neutraceutical, or cognitive behavioral therapies employed in this field. This chapter describes the classes of drugs that are likely to be encountered when treating patients with sleep disorders. First, a broad but brief overview of the history and evolution of sleep research and sleep pharmacology is presented followed by a brief overview of the brain mechanisms underlying the processes of wakefulness and sleep, including circadian aspects. Some key sleep-related disorders are described, and typical compounds that may be used to treat those disorders are reviewed. Adult sleep apnea syndrome, which is primarily treated with mechanical devices such as oral appliances or application of continuous positive airway pressure (CPAP), are not discussed. Apnea and bradycardia of prematurity, which are treated primarily with respiratory stimulants, are discussed in Chapter 8.
In addition to “sleeping pills,” many nondepressant medications are used in sleep medicine. In disorders such as restless legs syndrome (RLS) and PLMD, sleep onset is often delayed and fragmented. First-line therapy for these disorders includes dopamine agonists and, in some cases, opiates. Respiratory stimulants are another class of medications sometimes employed in the treatment of sleep disorders. Medroxyprogesterone and acetazolamide have been used in an effort to enhance ventilation in patients with high altitude–induced central sleep apnea or obesity-hypoventilation syndrome. More recently, the antidepressant mirtazapine has been shown to reduce apnea severity in animal models emulating sleep apnea as well as in some patients.2 An effective pharmaceutical treatment for obstructive sleep apnea does not yet exist.
Progression of sleep
Sleep was originally thought to be a passive rather than an active process. Canton, a physiologist at the Royal Infirmary in Liverpool, is credited with the discovery of electroencephalography (EEG), an important milestone in the understanding of sleep as an active process. His experimental observations on cortical currents in rabbits and monkeys were published in the British Journal of Medicine.3 Using a mirror galvanometer, Canton observed an increase in the amplitude of waves measured from the cortex during states of sleep as opposed to a decrement in cortical amplitudes during wakefulness. Canton was the first to perform sleep EEG in mammals.4 Subsequently, several observations including those of von Economo and the experiments by Morruzi and colleagues clearly showed that sleep is not a passive phenomenon but involves several brain regions, especially the diencephalon and the brainstem, which actively control sleep and states of arousal.5–8 In 1957, Dement and Kleitman reported the cyclical alternating pattern of non–rapid eye movement (NREM) and REM sleep.9
When determining the most appropriate pharmaceutical intervention for a sleep disorder, it is important first to consider how sleep is defined and measured and the normative values of time spent in sleep and in each sleep stage (i.e., sleep architecture). Mammalian sleep can be defined as a cyclical, reversible behavioral state of perceptual disengagement from, and unresponsiveness to, the external environment. Within normal human monophasic sleep, sleep is polygraphically characterized into two distinct stages based on a constellation of behavioral and electrophysiologic parameters. These two stages are NREM and REM sleep. NREM sleep is categorized further into stages N1-N3 (formerly known as stages 1 to 4); N1 is the lightest and stage N3 is the deepest sleep stage. Figures 23-1 to 23-4 show examples of EEG-defined wakefulness followed by examples of stages N1 to N3.
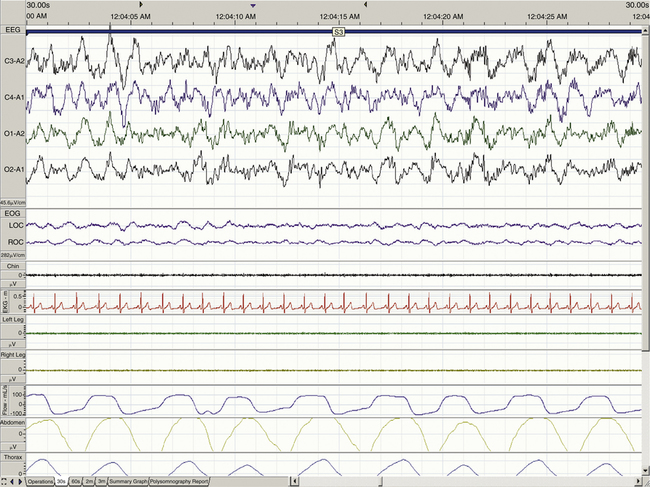
The term for REM sleep is derived from the periodic bursts of REMs during sleep. REM sleep has both tonic (persistent) and phasic (episodic) components. During tonic REM sleep, the EEG tracing shows a similar pattern to that of N1, but it may also exhibit increased activity in the theta frequency range (3 to 7 Hz) and sawtooth-type waves. REM sleep is also accompanied by a generalized muscle atonia, with the exception of the extraocular muscles and the diaphragm. Figure 23-5 shows an example of EEG-defined REM sleep.
Aserinsky and Kleitman were the first to observe the electrophysiologic characteristics of REM sleep and, in particular, the rapid, jerky, and binocularly symmetric eye movements in this sleep stage.10 EEG patterns similar to wakefulness were noted, showing the characteristic fast desynchronized rhythms in the cortical EEG, and the term paradoxical sleep was introduced by Jouvet and Michel in 1960; the term active sleep was used by other researchers. These terms are used interchangeably in the literature, although subtle differences exist. Additionally, autonomic activation occurs during this state as respiratory and heart rates are increased. Dream recall is also common when subjects are awakened during this stage, whereas dream recall during NREM sleep is relatively rare (Table 23-1).
TABLE 23-1
Electroencephalographic Correlates of Sleep Stages
CHARACTERISTICS | |||||
SLEEP STAGES | TST (%) | EEG | EOG | EMG | OTHER VARIABLES |
Stage awake (relaxed wakefulness) | Alpha activity (8-12 Hz) or low-amplitude beta (13-35 Hz), mixed-frequency waves | REM (in sync or out of sync deflections), eye blinks | Relatively high tonic EMG activity | Alpha activity in occipital leads compared with central leads, eye opening suppresses alpha activity, movement artifacts | |
N1, formerly known as stage 1 | 2-5 | Low-voltage, mixed-frequency waves (2-7 Hz range), mainly irregular theta activity, triangular vertex waves | SEMs, waxing and waning of alpha rhythm | Tonic EMG levels typically below range of relaxed wakefulness | Alpha ≤50%, vertex sharp waves in central leads, absence of spindles and K complexes |
N2, formerly known as stage 2 | 45-55 | Relatively low-voltage, mixed-frequency waves, some low-amplitude theta and delta activity | No eye movement | Low chin muscle activity | Sleep spindles (7 to 14 Hz) and K-complexes occur intermittently |
N3, formerly known as stages 3 and 4 | 5-20 | ≥20%-50% of epoch consists of delta (0.5-2 Hz) activity | No eye movement | Chin muscle activity is lower than N1 and N2 | Sleep spindles may be present |
Stage REM | 20-25 | EEG is relatively low voltage with mixed frequency resembling N1 sleep | Episodic rapid, jerky, and usually lateral eye movements in clusters | EMG tracing almost always reaches its lowest levels owing to muscle atonia | Phasic and tonic components, presence of sawtooth waves, alpha waves are 1-2 Hz slower than waves occurring during wakefulness and non-REM sleep |
Sleep stages occur in cycles that repeat approximately every 90 to 120 minutes. A normal sleep cycle begins with N1 and proceeds through N3. Sleep rapidly passes through the same stages in reverse order before REM sleep is initiated, usually first occurring about 90 minutes after sleep onset. Although significant interindividual variation in sleep need is noted, adult humans typically sleep about 7 to 9 hours per night and spend almost one-third of their life sleeping. Figure 23-6 provides a graphic representation of the cyclical distribution of sleep states across a single night in a normal healthy adult.
Neurophysiologic mechanisms
Arousal and wakefulness
In a series of postmortem examinations of the brains of patients who had died as a result of the outbreak of encephalitis lethargica after World War I, von Economo8 observed that lesions in the rostral midbrain and posterior hypothalamus had a profound effect on sleep and wakefulness. He derived two significant correlates from his observations. The first was that lesions in the preoptic and basal forebrain (BF) areas caused severe insomnia. The second was that lesions in the posterior and lateral hypothalamus (LH) caused severe hypersomnia. On the basis of these data, von Economo hypothesized the existence of a group of sleep-promoting neurons around the hypothalamic optic chiasm and, conversely, a group of wake-promoting neurons in the area of the posterior hypothalamus. Both observations have been proved to be essentially correct, but it was only toward the end of the 20th century that the hypothalamic influences on sleep and wakefulness were integrated into the mechanisms of vigilance state control. Before that, the emphasis had been on brainstem mechanisms and the ascending reticular activating system (ARAS).
Ascending reticular activating system
Physiologic analysis of the mechanisms of EEG arousal and wakefulness began with the classic studies of Bremer, who, in 1935, showed that if the brainstem of a cat was completely transected at the level of the midbrain (i.e., to produce the cerveau isolé), the result was that the cat maintained a persistent state of sleep. Different interpretations of this result were possible until Moruzzi and Magoun6 demonstrated the existence of an active arousal center below the level of the transaction, in the pons (i.e., the brainstem). Subsequent lesion and electrical stimulation studies identified the brainstem core, or pontine reticular system, as a critical component of arousal and wakefulness. This system was termed the ascending reticular activating system and was morphologically defined by cell bodies that projected from the brainstem to innervate the midbrain and cortex.
A more recent advance has been the identification of the importance of a cholinergic activating system in EEG arousal. This is one of the major components of the ARAS, and its identification depended on methods that were developed for labeling neurons that contain specific neurotransmitters. Steriade and colleagues11 identified cells located near the pons-midbrain junction that increased their discharge rate about 60 seconds before the first change to an aroused state was noted on the EEG. These neurons were found to project to the thalamus, and the change in their discharge rate was the first indication of arousal. Subsequent work identified these neurons as containing the neurotransmitter acetylcholine and being localized to the laterodorsal pontine tegmentum/pedunculopontine tegmentum (LDT/PPT) region.
Cholinergic systems are not the exclusive substrate of EEG arousal, however, and evidence that multiple systems are involved in arousal and wakefulness came from the inability of lesions of any single one of these systems to disrupt EEG arousal on a permanent basis.12 Other brainstem reticular neuronal projections to the thalamus using glutamate neurotransmission and noradrenergic and serotoninergic projections from the locus caeruleus and raphe nuclei also play important roles in maintaining wakefulness. In addition to brainstem nuclei, a cholinergic input to the cortex that ascends from the BF nuclei, especially the nucleus basalis of Meynert, plays an important role. Histaminergic neurons localized in the tuberomammillary nucleus (TMN) of the posterior hypothalamus also promote wakefulness. Discovery of the orexin/hypocretin system in 1999 (see section on Narcolepsy later) led to another CNS arousal system being identified. It is probably the latter hypothalamic systems that were affected in the brains examined by von Economo.8
Thalamic mechanisms of arousal
Most AAS projections that mediate EEG arousal and wakefulness synapse in the thalamus, which is an essential center for the organization of EEG arousal and for maintaining activation at a cortical level. Thalamic mechanisms at a cellular level influence the differences between wakefulness and NREM sleep. A detailed consideration of these thalamic mechanisms is beyond the scope of this chapter; they depend primarily on the cells in the thalamus that project to the cortex (i.e., thalamocortical neurons).13 Thalamocortical neurons differ in their rate and pattern of discharge depending on the vigilance state. When the arousal-related glutamatergic, cholinergic, noradrenergic, and serotoninergic projections are active, they drive the thalamocortical neurons to discharge in single spike mode. This discharge keeps the EEG in an active state, which contributes to arousal and wakefulness.
Sleep onset and processes that maintain sleep
An important consideration is the mechanism that begins this process of reducing the drive from the activating (wakefulness) systems.14 In other words, how does sleep begin? Electrophysiologic recordings of cells in the BF and anterior hypothalamic regions showed that some of these neurons discharge only during sleep, and this was hypothesized to be an active sleep-promoting mechanism. Confirmation came from studies by Sherin and colleagues,15 who used anatomic techniques to detect neurons in the ventrolateral preoptic (VLPO) area that were selectively active during NREM sleep. Subsequent immunohistochemical studies identified the neurotransmitters contained in these cells as inhibitory γ-aminobutyric acid (GABA) and glycine. Anatomic work showed neurons containing GABA and glycine projected not only to wakefulness-promoting histaminergic neurons in the TMN and other hypothalamic centers including the BF, but also to all the brainstem nuclei important in EEG arousal.16 This group of cells coordinates the inhibition of activity