CHAPTER 7 Skin and its appendages
In this chapter, the types and functions of skin in different parts of the body are described first, followed by the microstructure of the epidermis and dermis, and the appendages of skin including the pilosebaceous units and the sweat glands and nails. The development of skin, natural skin lines and age-related changes, and clinical aspects of skin, e.g. grafts, surgical skin flaps and wound healing, are also described. The integumental system includes the skin and its derivatives, hairs, nails, sweat and sebaceous glands; subcutaneous fat and deep fascia; the mucocutaneous junctions around the openings of the body orifices; and the breasts. Mucocutaneous junctions and breast tissues are covered in the appropriate regional sections.
TYPES AND FUNCTIONS OF SKIN
CLASSIFICATION OF SKIN
Although skin in different parts of the body is fundamentally of similar structure, there are many local variations in parameters such as thickness, mechanical strength, softness, flexibility, degree of keratinization (cornification), sizes and numbers of hairs, frequency and types of glands, pigmentation, vascularity, innervation. Two major classes of skin are distinguished: they cover large areas of the body and show important differences of detailed structure and functional properties. These are thin, hairy (hirsute) skin, which covers the greater part of the body, and thick, hairless (glabrous) skin, which forms the surfaces of the palms of the hands, soles of the feet, and flexor surfaces of the digits (Fig. 7.1, Fig. 7.2, see Fig. 7.4).
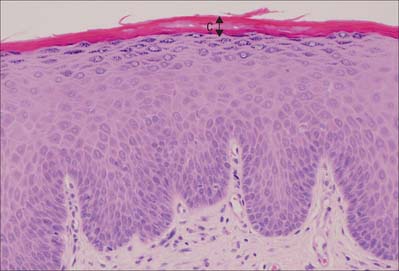
Fig. 7.2 The interfollicular epidermis of human thin skin. Note the thin cornified layer (C) in comparison with Fig. 7.4 (which is also at a lower magnification).
MICROSTRUCTURE OF SKIN AND SKIN APPENDAGES
EPIDERMIS
The epidermis (Fig. 7.2, Fig. 7.3) is a compound tissue consisting mainly of a continuously self-renewing, keratinized, stratified squamous epithelium: the principal cells are called keratinocytes. Nonkeratinocytes within the mature epidermis include melanocytes (pigment-forming cells from the embryonic neural crest), Langerhans cells (immature antigen-presenting dendritic cells derived from bone marrow), and lymphocytes. Merkel cells, which may function as sensory mechanoreceptors or possibly as part of the dispersed neuroendocrine system, are associated with nerve endings. Free sensory nerve endings are sparsely present within the epidermis. In routine histological preparations, the non-keratinocytes and Merkel cells are almost indistinguishable, and appear as clear cells surrounded by a clear space produced by shrinkage during processing. Their cytoplasm lacks prominent filament bundles.
The epidermis can be divided into a number of layers from deep to superficial as follows: basal layer (stratum basale), spinous or prickle cell layer (stratum spinosum), granular layer (stratum granulosum), clear layer (stratum lucidum) and cornified layer (stratum corneum) (Fig. 7.4). The first three of these layers are metabolically active compartments through which cells pass and change their form as they progressively differentiate. The more superficial layers of cells undergo terminal keratinization, or cornification, which involves not only structural changes in keratinocytes, but also alterations in their relationships with each other and with non-keratinocytes, and molecular changes within the intercellular space.
Keratinocytes
Basal layer
The basal or deepest layer of cells, adjacent to the dermis, is the layer where cell proliferation in the epidermis takes place. This layer contacts a basal lamina (Fig. 7.5, see Fig. 2.7), which is a thin layer of specialized extracellular matrix, not usually visible by light microscopy. By routine electron microscopy the basal lamina appears as a clear lamina lucida (adjacent to the basal cell plasma membrane) and a darker lamina densa. The basal plasma membrane of the basal keratinocytes, together with the extracellular basal lamina (lamina lucida and lamina densa) and anchoring fibrils within the subjacent dermal matrix (the lamina fibroreticularis), which insert into the lamina densa and loop around bundles of collagen, collectively form the basement membrane zone (BMZ) which constitutes the dermo-epidermal junction. This is a highly convoluted interface, particularly in thick, hairless skin, where dermal papillae (rete ridges) project superficially into the epidermal region, interlocking with adjacent downward projections of the epidermis (rete pegs) (Fig. 7.4).
The majority of basal layer cells (Fig. 7.3) are columnar to cuboidal in shape, with large (relative to their cytoplasmic volume) mainly euchromatic nuclei and prominent nucleoli. The cytoplasm contains variable numbers of melanosomes and, characteristically, keratin filament bundles corresponding to the tonofilaments of classic electron microscopy. In the basal keratinocytes these keratins are mostly K5 and K14 proteins. The plasma membranes of apposed cells are connected by desmosomes, and the basal plasma membrane is linked to the basal lamina at intervals by hemidesmosomes (Fig. 7.5, see Fig. 1.5). Melanocytes (see Fig. 7.9), occasional Langerhans cells (see Fig. 7.3) and Merkel cells (see Fig. 3.30) are interspersed among the basal keratinocytes. Merkel cells are connected to keratinocytes by desmosomes, but melanocytes and Langerhans cells lack these specialized contacts. Intraepithelial lymphocytes are present in small numbers.
At any one time the basal layer of the epidermis contains keratinocytes with different fates. These include multipotent stem cells. On division these may self-renew or produce a daughter cell which is committed to differentiate after undergoing further transit amplifying cell divisions. The activity of stem cells and transit amplifying cells in the basal layer provides a continuous supply of differentiating cells which enter the prickle cell layer. The great majority of these cells are postmitotic, although some cell division may occur in the more basal regions of the prickle cell layer. Stem cells are thought to reside mainly in the troughs of rete pegs, and in the outer root sheath bulge of the hair follicle, but they cannot easily be distinguished morphologically. The distribution of stem cells and the size of their proliferative units (see below) may be quite variable in human skin (Ghazizadeh & Taichman 2005).
The organization of the basal layer and overlying progeny cells is thought to form a series of columns. Several layers of prickle and granular cells overlie a cluster of six to eight basal cells, forming a columnar proliferative unit. Each group of basal cells consists of a central stem cell with an encircling ring of transit amplifying proliferative cells and postmitotic maturing cells. From the periphery of this unit, postmitotic cells transfer into the prickle cell layer. The normal total epidermal turnover time is between 52 and 75 days. In some pathologies of skin, turnover rates and transit times can be exceedingly rapid, e.g. in psoriasis, total epidermal turnover time may be as little as 8 days. The control of keratinocyte proliferation and differentiation is beyond the scope of this publication but is reviewed in Niemann & Watt (2002) and Byrne et al (2003).
Prickle cell layer
The prickle cell layer (Fig. 7.3, Fig. 7.6) consists of several layers of closely packed keratinocytes that interdigitate with each other by means of numerous cell surface projections. The cells are anchored to each other by desmosomes that provide tensile strength and cohesion to the layer. These suprabasal cells are committed to terminal differentiation and gradually move upwards towards the cornified layer as more cells are produced in the basal layer. When skin is processed for routine light microscopy, the cells tend to shrink away from each other except where they are joined by desmosomes, which gives them their characteristic spiny appearance. Prickle cell cytoplasm contains prominent bundles of keratin filaments, (mostly K1 and K10 keratin proteins) arranged concentrically around a euchromatic nucleus, and attached to the dense plaques of desmosomes. The cytoplasm also contains melanosomes, either singly or aggregated within membrane-bound organelles (compound melanosomes). Langerhans cells (see Fig. 7.11) and the occasional associated lymphocyte are the only non-keratinocytes present in the prickle cell layer.
Granular layer
Extensive changes in keratinocyte structure occur in the three to four layers of flattened cells in the granular layer. The nuclei become pyknotic and begin to disintegrate; organelles such as ribosomes and membrane-bound mitochondria and Golgi bodies degenerate; and keratin filament bundles become more compact and associated with irregular, densely staining keratohyalin granules (Fig. 7.6). Small round granules (100 × 300 nm) with a lamellar internal structure (lamellar granules, Odland bodies, keratinosomes) also appear in the cytoplasm. Keratohyalin granules contain a histidine-rich, sulphur-poor protein (profilaggrin) which, when the cell reaches the cornified layer, becomes modified to filaggrin. The lamellar granules are concentrated deep to the plasma membrane, with which they fuse, releasing their hydrophobic glycophospholipid contents into the intercellular space within the layer and also between it and the cornified layer. They form an important component of the permeability barrier of the epidermis, rendering it relatively waterproof.
Clear layer
The clear layer is only found in thick palmar or plantar skin. It represents a poorly understood stage in keratinocyte differentiation. It stains more strongly than the cornified layer with acidic dyes (Fig. 7.6), is more refractile optically, and often contains nuclear debris. Ultrastructurally, its cells contain compacted keratin filaments and resemble the incompletely keratinized cells which are occasionally seen in the innermost part of the cornified layer of thin skin.
Cornified layer
The cornified layer (Fig. 7.3, Fig. 7.6) is the final product of epidermal differentiation, or cornification. It consists of closely packed layers of flattened polyhedral squames (Fig. 7.7), ranging in surface area from 800 to 1100 μm2. These cells overlap at their lateral margins and interlock with cells of apposed layers by ridges, grooves and microvilli. In thin skin this layer may be only a few cells deep, but in thick skin it may be more than 50 cells deep. The plasma membrane of the squame appears thicker than that of other keratinocytes, partly due to the cross-linking of a soluble precursor, involucrin, at the cytoplasmic face of the plasma membrane, in the complex insoluble cornified envelope. The outer surface is also covered by a monolayer of bound lipid. The intercellular region contains extensive lamellar sheets of glycolipid derived from the lamellar granules of the granular layer. The cells lack a nucleus and membranous organelles, and consist solely of a dense array of keratin filaments embedded in a cytoplasmic matrix which is partly composed of filaggrin derived from keratohyalin granules.
Keratins
Keratins are the intermediate filament proteins found in all epithelial cells. There are two types, type I (acidic) and type II (neutral/basic); they form heteropolymers, are coexpressed in specific pairs and are assembled into 10 nm intermediate filaments. Fifty-four different keratin genes have been recognized and their protein products are numbered. The nomenclature for human keratins and keratin genes has recently been revised and is given in Schweizer et al (2006). Different keratin pairs are expressed according to epithelial cell differentiation; antibodies to individual keratins are useful analytical tools (Fig. 7.8). Keratins K5 and K14 are expressed by basal keratinocytes. New keratins, K1 and K10, are synthesized suprabasally. In the granular layer the filaments become associated with keratohyalin granules containing profilaggrin, a histidine-rich phosphorylated protein. As the cells pass into the cornified layer, profilaggrin is cleaved by phosphatases into filaggrin which causes aggregation of the filaments and forms the matrix in which they are embedded. Other types of keratin expression occur elsewhere, particularly in hair and nails, where highly specialized hard, or trichocyte, keratin is expressed. This becomes chemically modified and is much tougher than in the general epidermis. For a recent review of keratin function see Gu & Coulombe 2007.
Melanocytes
Melanocytes are melanin pigment-forming cells derived from the neural crest (Fig. 7.9, Fig. 7.10). They are present in the epidermis and its appendages, in oral epithelium, some mucous membranes, the uveal tract (choroid coat) of the eyeball, parts of the middle and internal ear and in the pial and arachnoid meninges at the base of the brain. The cells of the retinal pigment epithelium, developed from the outer wall of the optic cup, also produce melanin, and neurones in different locations within the brainstem (e.g. the locus coeruleus and substantia nigra) synthesize a variety of melanin called neuromelanin. True melanins are high molecular weight heteropolymers attached to structural protein. In humans there are two classes, the brown-black eumelanin, and the red-yellow phaeomelanin, both derived from the substrate tyrosine. Most natural melanins are mixtures of eumelanin and phaeomelanin, and phaeomelanic pigments, trichochromes, occur in red hair.
In albinism, the tyrosinase required for melanin synthesis is either absent or inactive, and melanocytes, though present, are relatively quiescent cells in an otherwise normal epidermis. Melanocytes decrease significantly in numbers in old age, and are absent from grey-white hair. For further reading on melanocyte function in health and disease, see Goding (2007).
Langerhans cells
Langerhans cells (Fig. 7.11) are immature dendritic antigen-presenting cells (see p. 79) regularly distributed throughout the basal and prickle cell layers of the epidermis and its appendages, apart from the sweat gland. They are also present in other stratified squamous epithelia, including the buccal, tonsillar and oesophageal epithelia, as well as the cervical and vaginal mucosae and the transitional epithelium of the bladder. They are found in the conjunctiva, but not in the cornea. In routine preparations they appear as clear cells, relatively high in the stratified layer. They enter the epidermis from the bone marrow during development to establish the postnatal population (460–1000/mm2, 2–3% of all epidermal cells, with regional variations), and this is maintained by continual replacement from the marrow.
The nucleus is euchromatic and markedly indented and the cytoplasm contains a well-developed Golgi complex, lysosomes (which often contain ingested melanosomes), and a characteristic organelle, the Birbeck granule. The latter are discoid, cup-shaped, or have a distended vesicle resembling the head of a tennis racket; in section they often appear as a cross-striated rod 0.5 μm long and 30 nm wide. When stimulated by antigen, Langerhans cells migrate out of the epidermis to lymphoid tissues (see Fig. 4.14). Their numbers are increased in chronic skin inflammatory disorders, particularly of an immune aetiology, such as some forms of dermatitis.
DERMIS
The dermis (Fig. 7.1, Fig. 7.4, see Fig. 7.17) is an irregular, moderately dense connective tissue. It has a matrix composed of an interwoven collagenous and elastic network in an amorphous ground substance of glycosaminoglycans, glycoproteins and bound water, which accommodates nerves, blood vessels, lymphatics, epidermal appendages and a changing population of cells. Mechanically, the dermis provides considerable strength to the skin by virtue of the number and arrangement of its collagen fibres (which give it tensile strength), and its elastic fibres (which give it elastic recoil). The density of its fibre meshwork, and therefore its physical properties, varies within an area, in different parts of the body, and with age and sex. The dermis is vital for the survival of the epidermis, and important morphogenetic signals are exchanged at the interface between the two both during development and postnatally. The dermis can be divided into two zones, a narrow superficial papillary layer and a deeper reticular layer: the boundary between them is indistinct.
Two major categories of cell are present in postnatal dermis, permanent and migrant, as is typical of all general connective tissues (see Ch. 2). The permanent resident cells include cells of organized structures such as nerves, vessels and cells of the arrector pili muscles, and the fibroblasts, which synthesize all components of the dermal extracellular matrix. The migrant cells originate in the bone marrow (Fig. 4.12) and include macrophages, mast cells, eosinophils, neutrophils, T and B cells (including antibody-secreting plasma cells), and dermal interstitial dendritic cells which are capable of immune surveillance and antigen presentation.
Layers of the dermis
Papillary layer
The papillary layer is immediately deep to the epidermis (Fig. 7.4), and is specialized to provide mechanical anchorage, metabolic support, and trophic maintenance to the overlying epidermis, as well as supplying sensory nerve endings and blood vessels. The cytoskeleton of basal epidermal keratinocytes is linked to the fibrous matrix of the papillary dermis through the attachment of keratin filament bundles to hemidesmosomes, then via anchoring filaments of the basal lamina, to the anchoring fibrils of type VII collagen which extend deep into the papillary dermis (Fig. 7.5). This arrangement provides a mechanically stable substratum for the epidermis.
The superficial surface of the dermis is shaped into numerous papillae or rete ridges, which interdigitate with rete pegs in the base of the epidermis and form the dermo-epidermal junction at their interface. The papillae have round or blunt apices which may be divided into several cusps. In thin skin, especially in regions with little mechanical stress and minimal sensitivity, papillae are few and very small, while in the thick skin of the palm and sole of the foot they are much larger, closely aggregated, and arranged in curved parallel lines following the pattern of ridges and grooves on these surfaces (Fig. 7.1). Lying under each epidermal surface ridge are two longitudinal rows of papillae, one on either side of the epidermal rete pegs through which the sweat ducts pass on the way to the surface. Each papilla contains densely interwoven, fine bundles of types I and III collagen fibres and some elastic fibrils. Also present is a capillary loop (Fig. 7.4), and in some sites, especially in thick hairless skin, Meissner’s corpuscle nerve endings.
Hypodermis
The quantity and distribution of subcutaneous fat differs in the sexes. It is generally more abundant and widely distributed in females. In males it diminishes from the trunk to the extremities, and this distribution is more obvious in middle age, when the total amount increases in both sexes. The amount of adipose tissue in the hypodermis, as elsewhere, reflects the quantity of lipid stored in its adipocytes rather than a change in the number of cells. There is an association with climate (rather than race), and superficial fat is more abundant in colder geographical regions. The hypodermis is most distinct on the lower anterior abdominal wall, where it contains much elastic tissue and appears many-layered as it passes through the inguinal regions into the thighs. It is similar in the limbs and the perineum, but is thin where it passes over the dorsal aspects of the hands and feet, the sides of the neck and face, around the anus, and over the penis and scrotum. It is almost absent from the external ears but is particularly dense in the scalp, palms and soles, where it is crossed by numerous strong connective tissue bands binding the hypodermis and skin to underlying structures: these are part of the deep fascia, but are known regionally as aponeuroses of the scalp, palm and sole.
PILOSEBACEOUS UNIT
The pilosebaceous unit consists of the hair and its follicle with an associated arrector pili muscle, sebaceous gland, and sometimes an apocrine gland (Fig. 7.1, Fig. 7.12). Not all elements of the unit occur together in all body regions.
Hairs
Hairs are filamentous cornified structures present over almost the entire body surface. They grow out of the skin at a slant (Fig. 42.1) as is evident in the sloping of the hairs on the dorsum of forearm, hand and fingers towards the ulnar side. Hairs are absent from several areas of the body, including the thick skin of the palms, soles, the flexor surfaces of the digits, the thin skin of the umbilicus, nipples, glans penis and clitoris, the labia minora and the inner aspects of the labia majora and prepuce. The presence, distribution and relative abundance of hair in certain regions such as the face (in males), pubis and axillae, are secondary sexual characteristics which play subtle roles in sociosexual communication. There are racial variations in density, form, distribution and pigmentation, as well as individual variations. Hairs assist minimally in thermoregulation: on the scalp they provide some protection against injury and the harmful effects of solar radiation. They have a sensory function.
Hair follicle
The hair follicle (Fig. 7.1, Fig. 7.12, Fig. 7.13A) is a downgrowth of the epidermis containing a hair, which may extend deeply (3 mm) into the hypodermis, or may be more superficial (1 mm) within the dermis. Typically, the long axis of the follicle is oblique to the skin surface; with curly hairs it is also curved. There are cycles of hair growth and loss, during which the follicle presents different appearances. In the anagen phase the hair is actively growing and the follicle is at its maximum extent of development. In the involuting or catagen phase, hair growth ceases and the follicle shrinks. During the resting or telogen phase the inferior segment of the follicle is absent. This is succeeded by the next anagen phase. Further details of the hair growth cycle are given below, after the description of the anagen follicle and hair.