Chapter 83 Safety and Survival at Sea
For online-only figures, please go to www.expertconsult.com
The 1979 Fastnet Race distinguished itself as the worst disaster in the long history of ocean yachting. A surprise killer storm crossed the Irish Sea between southwest England and southern Ireland and exploded without warning in the midst of the Fastnet racing fleet. Suddenly, 2700 men and women in 303 ocean sailing yachts unwittingly became participants in hundreds of incidents of survival at sea. Winds of force 10 (55 knots) with much stronger gusts, and seas as high as 15 m (50 feet) knocked down 48% of the fleet until their masts paralleled the water; 33% of the fleet experienced knockdowns substantially beyond horizontal, including total inversions and full 360° rolls in the case of at least 26 yachts. Despite a massive response of rescue personnel and equipment, 24 yachts were abandoned and five of those sank. Fifteen sailors died and 136 were rescued from disabled yachts or the water. The official Fastnet Race Inquiry noted, “The common link among all 15 deaths was the violence of the sea, an unremitting danger faced by all who sail.” It concluded: “The sea showed that it can be a deadly enemy and that those who go to sea for pleasure must do so in the full knowledge that they may encounter dangers of the highest order.”1 The Fastnet storm had two positive results. Boats, safety gear, and safety procedures were improved dramatically, and sailors began to talk realistically about the risks of sailing and came to regard safety as a necessary discipline. The first public safety at sea seminars were held in Annapolis and New York in the shadow of the Fastnet shock. Since then, hundreds of safety and seamanship seminars have been held across America and in other sailing locations.
Who’s At Risk?
Analysis of U.S. recreational boating accident statistics for 2010, the most recent data available from the Coast Guard in their annual report “Recreational Boating Statistics 2010,” indicates there were 672 boating fatalities in 2010 (736 in 2009). Capsizing and falls overboard from open motor boats, rowboats, canoes, and kayaks accounted for more than one-half of the fatalities. The most common types of vessels involved in open accidents were open motor boats (46%), personal water craft (20%), and cabin motorboats (14%). The Coast Guard Recreational Boating Accident Report Database (BARD) shows an average of 5000 recreational boating accidents annually. Sixty percent of all accidents involve operator and passenger controllable factors, and 25% involve boat or environmental factors. Careless/reckless operation, inattentive and inexperienced boat operators boating at unsafe speeds without proper lookout, and risky passenger/skier behavior cause most fatalities and injuries. Ninety percent of deaths occurred on boats where the operator has received no boating safety instruction. Collision with another boat is responsible for the greatest number of nonfatal injuries. Collision with a fixed object, flooding/swamping, water skier mishaps, and capsizing rank second, third, fouth, and fifth in accident rank, and account for 60% of fatalities. Open motorboats less than 8 m (26 feet) in length and jet-ski personal watercraft (PWC) comprise two-thirds of the water craft involved in collisions, and are the vessel types with the most casualties and deaths. Alcohol is a leading contributing factor in recreational boating fatalities, responsible for at least 20% to 25% of deaths. These statistics indicate that one of the greatest threats to a boater’s safety in “home waters” is the inexperienced operator heading directly toward you at excessive speed, unaware that you are dead ahead. Extra vigilance is required in order to take evasive action to avoid collision. Two-thirds of all fatalities in accidents on sailboats and small nonmotorized craft are from drowning, and 90% of victims are not wearing a lifejacket (see discussion below). Eight out of 10 drowned boaters are from boats less than 6 m (21 feet) long. The full report can be reviewed on line at http://www.uscgboating.org/statistics/accident_statistics.aspx.
Health Issues at Sea
Seasickness
Seasickness impairs cognitive function. Sailors often lose the ability to multitask, making it difficult to analyze and integrate complex data, which leads to impaired judgment and faulty decisions. Cognitive failure is also expressed as loss of short-term memory. This impairment makes it difficult to engage in problem solving. Compounding this problem are the medications used to prevent or treat seasickness. Their side effects may include drowsiness, confusion, and loss of concentration. The underlying mechanism of seasickness involves a conflict of sensory input processed by the brain to orient the body’s position. Someone positioned in the cabin of a heeling or rolling boat is inviting seasickness. Below decks, the eyes oriented to the cabin sole and ceiling detect no tilt from vertical, while fluid in the inner ear’s vestibular system (semicircular canals and the otolith organs) constantly shifts. Position sensors (proprioceptors) in the neck, muscles, and joints send additional signals, depending on how a person shifts and secures him or herself from falling. This mix of sensory data from the eyes, inner ear, and position sensors arrives in complex and conflicting combinations, creating a “sensory conflict” that activates the emetic center in the brainstem. According to Dr. Charles Omen, Director of the Man Vehicle Space Lab at MIT and an authority on motion sickness, sensory conflict is a sensory cue “expectancy” conflict, not an intermodality conflict. It occurs when signals from the inner ear don’t match expectations based on one’s own commanded self movement, or concurrent visual or proprioceptive cues. In response to this “sensory conflict,” there is activation of a complex and not well-understood anatomic connection between the vestibular nuclei in the brainstem, the cerebellum, and the autonomic and emetic centers. Stimuli from the vestibular and visual systems can independently initiate symptoms. Sickness occurs most commonly with acceleration in a direction perpendicular to the longitudinal axis of the body, which is why head movements away from the direction of motion are so provocative. When these stimuli are presented in isolation in the laboratory, visual stimulation is more important than vestibular input in causing motion sickness. There is no increase in symptoms when combined stimulation is applied. Blind people can become seasick; the conflict arises when input from the vestibular system does not match the individual’s expectations derived from previous motion experience. The intensity of conflicting input can be amplified when compared with these expectations. Deaf subjects are susceptible to motion sickness. If the semicircular canals and otolith organs produce sensory cues that are incongruous, seasickness ensues. If the visual system indicates movement but the vestibular system does not (in-flight simulators and movie theaters), motion sickness may ensue. Medication is more effective in preventing symptoms than in reversing them. Therefore, anti-seasickness medication should be administered before leaving port, or the night prior to departure (Table 83-1). One should begin any trip well hydrated and free of the after-effects of alcohol, which impairs vestibular function by sensitizing the vestibular apparatus to motion. One is advised to eat lightly. Anecdotal reports favor eating carbohydrates rather than protein, but no conclusive study favors any particular food or diet. Many sailors favor eating soda crackers or bread. One should try to snack on bland foods throughout the day, even if anorectic, to maintain energy levels until meals are regularly tolerated. Cheese and crackers, energy bars, fruit, trail mix, dry granola, and popcorn work best. Drinking small amounts of fluid frequently is recommended to avoid dehydration. Many sailors believe drinks high in vitamin C prevent seasickness; however, there are no data to support this notion.
Medication | Dose | Interval |
---|---|---|
Diphenhydramine, (Benadryl) (OTC) | 25 or 50 mg tab | 6-8 hr |
Dimenhydrinate, (Dramamine) (OTC) | 50 or 100 mg tab (max 400 mg/day) | 4-6 hr |
Meclizine (OTC) | 12.5 or 25 mg tab (max 100 mg/day) | 6-8 hr |
Bonine (Meclizine) (OTC) | 25 mg chewable tab | 6-8 hr |
Cinnarizine (Stugeron) | 15 mg tab (max 100 mg/day) | 6-12 hr |
Scopolamine (Transderm Scōp) | 1.5 mg skin patch | 72 hr |
(Scopace) | 0.4 mg (max 2.4 mg/day) | 8 hr |
Promethazine, (Phenergan) | 12.5/25/50-mg tab, suppository, deep IM injection | variable intervals, depending on dose/preparation |
The antihistamines meclizine (Bonine) and dimenhydrinate (Dramamine) are available over the counter (OTC) without prescription. They are effective for many sailors, as are the other prescription medications listed in Table 83-1. The popular antihistamine cinnarizine (Stugeron) is not sold in the United States, but is available OTC in Europe, Bermuda, Mexico, and Canada. It can be obtained legally from http://www.canadadrugsonline.com. It is favored by many sailors because it is less sedating than all the other antihistamines and has fewer reported side effects (described below).
Health Maintenance at Sea
Fahrenheit
Hypothermia (see Chapters 5 and 6) may develop acutely when someone is suddenly immersed in cold water, or over a period of hours to days during prolonged exposure to the elements. Mild hypothermia, defined as a core temperature above 32° C (90° F), is the only level that can be treated aboard a boat. Deeper levels require evacuation to a medical facility. Sustained uncontrollable shivering is the most reliable and earliest sign of a drop in core temperature. Other early clues are alterations in motor skills and changes in mental status. As blood is diverted from the muscles and nerves, there is loss of manual dexterity, large muscle coordination, and strength. Clumsiness while performing simple tasks, such as adjusting binoculars or using navigational instruments, is apparent. Walking safely on deck and working with lines and gear becomes hazardous. Subtle changes in mental status cause impaired judgment, confusion, and disorientation. There are changes in personality and frequent errors in judgment. Initial treatment of a fully conscious and shivering mildly hypothermic person with a core temperature above 32° C (90° F) is to prevent further cooling and heat loss. The person is still capable of rewarming him or herself and does not require evacuation. Shelter the victim from wind and water. Replace wet clothing with multiple layers of dry insulating garments after the skin is completely dried. If dry clothing is not available, provide an extra vapor barrier with added foul weather gear. A windproof layer minimizes convective and evaporative heat loss. When practical, wrap the victim in blankets, sleeping bag, sails, or sail bags. Provide calories with simple carbohydrate foods and sweet liquid drinks, and allow vigorous shivering to continue in order to generate rewarming heat. Warm liquids are psychologically beneficial but will not influence rewarming rates.
Gear and Equipment
Life Jackets
Inflatable vests have a flat and lightweight design that allows them to lie flush against the body so they do not restrict movement. They have excellent wearability and superb flotation capabilities. The vests provide as much as 16 kg or 150 newtons (35 lb) of buoyancy, compared with 10 kg (22.5 lb) in type I and 7 kg (15.5 lb) in types II and III. In most cases, added buoyancy enables the person to float higher, making it easier to breathe, and reduces the risk of aspirating seawater in rough sea conditions. By keeping the head and chest higher out of the water, it is easier to adopt the heat escape lessening position (HELP) (see Chapter 75). With buoyancy high on the chest, there is also better righting ability and head support. The offshore model can be purchased with an integrated safety harness (Figure 83-1).
Emergencies at Sea
Crew Overboard
Remaining Aboard
Recovery of Crew Overboard
Special equipment designed for locating and retrieving a person overboard should be deployed immediately. This gear should be ready for easy deployment and must release instantly. Too often, the gear is protected against accidental loss by extra wraps of line to the stern pulpit or rigging. Any delay in releasing COB gear will leave it too far from the victim. A crew overboard pole is a 4- to 5-m (12- to 15-foot) floating flagpole that is ballasted to remain upright in rough seas. Without the drogue accessory (a parachute-shaped device to slow a vessel’s drift downwind), it will quickly drift away from the designated area. A man overboard module (MOM) automatically deploys a CO2-activated horseshoe buoy and a 2-m (6-foot) inflatable locator pole equipped with a drogue and water-activated, lithium-powered light (Figure 83-2). A variety of lights have been developed to serve as rescue beacons. The overboard marker strobe marks the site and illuminates the scene for the rescuers. It automatically activates when thrown into the water. Waterproof personal rescue strobe lights attached to a life jacket can flash for 8 hours at 1-second intervals and are visible a mile away. Other personal strobe lights can last up to 60 hours, with variable rates to conserve battery power. A U.S. Navy whistle has a special flat design to prevent the whistle body from holding water and dampening the sound. Attach one to every life jacket.
Electronic overboard alarms are available for crew; these are small transmitters alerting the mother ship receiver. The personal alarms are activated when they contact the water or when manually turned on (Figure 83-3, online). All onboard GPS units have a MOB function, which, if activated, will create a waypoint at the vessel’s position when pressed. It will also make the waypoint “active,” so the vessel can return to the latitude and longitude of the person at the time of the fall. The GPS is a powerful search and rescue tool, but is not a substitute for maintaining strict visual contact and using signal lights and markers. The inherent small degree of error with GPS in marking the waypoint of the MOB is magnified in heavy winds, large seas, and strong currents, because the person may drift downwind or down-current while the boat returns to the scene. GPS receivers receive signals from U.S. Air Force satellites and then compute the location accurately to within 10 m. It may still be impossible to locate the person in rough seas or during reduced visibility.
Rather than rely on the ship’s emergency locator beacon, sailors can wear them on their person. A small unit can be worn (on a life jacket) by a crew member as a personal locator beacon (PLB). Weighing as little as 500 mL (1 pint), and not much larger than a cell phone, it also transmits on the 406- and 121.5-MHz frequencies. The battery life for this compact unit exceeds 24 hours. PLBs do not float upright in the transmitting position. The transmitter requires manual activation and must be held out of the water with the antenna pointed skyward (Figure 83-4). It would be difficult to do this without wearing a life jacket. Some units have an integrated GPS to yield location accuracy to within less than a 91-m (300-foot) radius, versus a 5-km (3-mile) radius from the 406-MHz beacon operating alone. The problem is that most boats, other than professional rescue vessels [or SAR aircraft], are not equipped with a receiver capable of locating the source of the “homer” beacon on the PLB. All PLBs require registration with NOAA. A less technical signaling device designed for night use is the Rescue Laser Flare. This is a handheld light that produces a visible line of laser beam to attract the attention of search boats and aircraft within a 10- to 20-mile radius (Figure 83-5, online).
Crew Overboard Maneuvers
The goal is to have the MOB back on the boat as quickly as possible. Practice the different techniques and decide which method and modifications work most effectively. The Lifesling, developed to enable one person to retrieve a person overboard, is a flexible floating collar that doubles as a hoisting sling (Figure 83-6, online). Deploy the collar from the stern pulpit and deliver it by repeatedly circling the victim, much as a ski boat maneuvers to deliver the towrope to a fallen water skier. After securing the horseshoe over the head and under the arms, pull the victim back to the boat and hoist him or her in the apparatus with the assistance of a halyard and winch (Figure 83-7). Lifelines are an obstacle to bringing the MOB back on deck, but are an important source of protection for the remaining crew. You may elect to secure lifelines at the stern (or transom) with lashing rather than shackles or pins so they can be easily cut and released in a recovery should the need arise.
If the crew has lost sight of the victim, immediately call for assistance. A mayday call on VHF-FM channel 16 will notify the Coast Guard and simultaneously alert all ships in the area monitoring this channel. The last known position should be obtained using GPS or reference to any navigational buoys and landmarks on shore. Rescuers should perform repeat searches of the area, because it is easy to miss someone in poor visibility or choppy seas. The victim will be far more likely to be located if he has the correct gear. Ideally, this includes a high-visibility (orange or yellow) life jacket with reflective tape combined with a safety harness, personal strobe light, loud whistle, packet of waterproof self-launching meteor flares, fluorescent dye marker, and a PLB or other crew-overboard alarm. Perhaps the most important factors for a successful rescue are the crew’s familiarity with the boat and the MOB equipment, and their teamwork, leadership, and expertise developed from practicing the maneuvers. A full review of the 2005 Crew Overboard Rescue Symposium conducted on San Francisco Bay can be found at http://www.boatus.com/foundation/findings/COBfinalreport. This study reviews the challenges for a successful recovery, required skills of the crew, preferred recovery maneuvers, and equipment that can be helpful in locating and retrieving the victim. It is a “must” read for every boater. It is also available in a slightly different format from U.S. Sailing on the website http://www.ussailing.org/safety/Studies/COB.pdf.
Flooding
Flooding, and the potential for sinking, is a threat to every boater. Boat U.S. Marine Insurance examined 50 claims from recreational boats that sank while underway, ranging from a tiny personal watercraft to a 16.5-m (54-foot) ocean-going sailboat. The full report is on the website at http://www.boatus.com/foundation/guide/index.html. Thirty-four percent of the boats sank because of leaks at thru-hulls, outdrive boots, or the raw water cooling system/exhaust. The single most critical reason small motorboats flood in open water relates to transom height. Engine cut-outs may be only inches above the waves, and the motor well may not protect the cockpit. Often, weight distribution of passengers and gear to the stern contributes to the problem.
Flooding may occur from failure of systems or construction (6% of the boats sank after coming down hard off of waves and therefore splitting open), or structural damage from collision and extreme weather (Box 83-1). Before abandoning ship, quickly assess the damage. The limiting factor is time. Stock the proper tools and repair supplies in a damage control kit (Box 83-2), and know how to respond quickly and skillfully. Assign duties to the crew before departure so that they know what to do in the event of flooding (and crew overboard, fire, grounding, and dismasting). Duties include damage control, radio transmission of a Mayday (can be cancelled later if necessary), and preparation to abandon ship.
BOX 83-1 Sources of Flooding
Reliable bilge pumps are the best defense against flooding (Figure 83-8, online). An excellent pump can buy time for locating and plugging the leak. However, no pump, manual or electric, can keep up with even a modest-sized hull breach. If a boat is equipped with an automatic bilge pump (or pumps), install a cycle counter on the pump and an “on” light to alert the crew when the pump is activated. A second emergency pump mounted above the first, using a separate float switch, can provide added pumping capability if the first pump cannot keep up with the leak. In this case, an alarm installed in the circuit can alert the crew to flooding.
Large holes can be overlaid with a collision mat (Figure 83-9) placed outside the hull to supplement a temporary interior patch (see below). The mat is a piece of heavy canvas or vinyl-coated fabric with grommets and lines that enable it to be positioned and secured on the exterior surface. It is held in place by pressure and lines. Collision mats can be purchased or improvised by using a small sail or awning material, although it is a mistake to make the mat too large; generally 1.2 m (4 feet) on an edge of the triangular shape is adequate. The water pressure automatically spreads the patch over the hole to form an effective seal and holds it in place. A commercial hull repair kit features flexible oval concave sheet metal plates with rubber gaskets. A bolt is welded to the intended exterior piece. The idea is to slip one oval through the hull breach, then back it from the inside with the second metal plate and tighten both together with a thumbscrew.
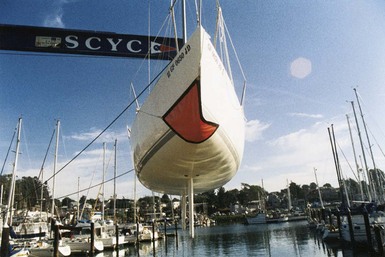
FIGURE 83-9 Triangular patches made from coated fabrics can be held in place with lines attached to each corner.
If flooding and sinking are inevitable, the captain should consider running the boat onto shore.
Fire
Uncontrolled fire is a disaster aboard ship. Fires aboard wood and fiberglass boats have the potential to double in size every 10 seconds. Approximately 7500 pleasure boat fires and explosions occur annually; of these, 10% are declared total losses. More than one-half of the 2700 fire-related injuries incurred each year occur on small, open motor boats. According to statistics compiled by Boat U.S. Marine Insurance claims investigations (www.boatus.com/seaworthy/fire/default.asp), the leading causes of fires on boats (55%) are AC and DC wiring faults. The most common electrical problem is related to chafed wires. Many fires are started by battery cables, bilge pump wires, and even instrument wires chafing on hard objects like vibrating engines or sharp-edged bulkheads. The DC voltage regulator is responsible for 25% of electrical fires. Eleven percent of fires are started by the boat’s AC system, frequently at the shore power inlet box. A small number of fires every year are caused by AC heaters and other household appliances that have been brought on board. Nearly one-quarter of boat fires (24%) were started by overheated propulsion systems. Frequently, an intake or exhaust cooling water passage was obstructed, causing the engine to overheat and begin to melt down hoses and impellers. These fires tended to be less serious, but because of the amount of smoke and the fact the fires come from areas with flammable fuels, they appeared more threatening. Often the fires were simply smoldering rubber, until the engine compartment was opened, allowing fresh air to enter. Lightning is a major cause of boat fires at marinas in Florida. Box 83-3 lists ways to prevent fires aboard ship.
BOX 83-3 Fire Prevention at Sea
The Coast Guard requires all recreational vessels to have portable fire extinguishers (Figure 83-10, online). The standard multipurpose dry chemical extinguisher (filled with monoammonium phosphate) and the newer dry chemicals discussed previously can be used on all types of fires: class A, B, or C. They extinguish and suffocate the fire by preventing access to oxygen. A major drawback is the extremely brief window of opportunity provided to put out the fire. The common B-1 extinguisher discharges completely in just 10 to 13 seconds. Units with greater capacity simply deliver more chemical over the same period. Because of the short discharge time, more than one fire extinguisher should be available to cope with a large blaze.
Dedicate one portable extinguisher to the engine compartment, a second to the galley, a third to the area under the fore hatch, and place the fourth (and largest size practical to stow) in a cockpit locker. One should never have to walk more than one-half the boat’s length to reach an extinguisher. The ideal location for a portable extinguisher in a closed compartment is next to the exit door. Fires involving common combustible solid material can be brought under control by cooling them with large amounts of water. Fires involving flammable or combustible liquids can be smothered to remove the oxygen by using a fire blanket. Electrical fires can be difficult to extinguish because the source of the heat (a shorted wire) can re-ignite the fire even after a fire extinguisher has been used. Terminating the electric power can often control electrical fires, especially when a short circuit is generating sufficient heat to cause other materials to combust. Every boat must have a main battery switch and/or AC breaker to turn off the entire electrical system. Water does not extinguish electrical fires, but may be effective on the resulting Class A fire after the electrical circuit has been disconnected. See Box 83-4 for fire-fighting guidelines.
BOX 83-4 Guidelines for Fighting Fire at Sea
Collisions with Other Vessels
Radar is invaluable in poor visibility (Figure 83-11, online). At night or in fog, radar can be used to identify other ships, hazards to navigation, squalls, and other local weather that may endanger a vessel.
For collision avoidance, many cruising sailboats install a masthead strobe light. Although these are highly visible, they are not accepted internationally as legal running lights, and the Coast Guard does not recommend them. A flashing strobe light may be interpreted as a distress signal, paradoxically inviting an unwelcome convergence of ships. Shining a powerful spotlight on the mainsail, turning on spreader lights, or igniting a white “anti-collision” flare can be an effective alternative means of alerting a ship to the danger of collision. It is recommended that cruising sailboats have masthead running lights for coastal and offshore cruising (to extend the range of visibility), in addition to deck-level lights for meeting, crossing, and passing maneuvers when vessels are nearby. The LED tricolor masthead light combines sidelights, sternlight, and all-round white light. It is noted for its energy efficiency, durability, longevity, and brightness. The U.S. Coast Guard Navigation Rules, International-Inland, specifies light requirements for every description of watercraft. Details can be found online at http://www.boatus.org/onlinecourse/reviewpages/boatusf/project/info2c.html.
Mariners should recognize the different patterns of lights displayed by commercial ships, Beyond U.S. coastal waters, many ships do not always show the appropriate lights. The risk of collision is therefore increased. Box 83-5 reviews measures to prevent collisions at sea.
BOX 83-5 Preventing Collisions at Sea
COLREGS, International regulations to prevent collisions at sea.
Sailors crossing the North Atlantic in summer along a great circle route to Europe need to beware of colliding with icebergs. Every year from February to July, more than 10,000 icebergs are separated from Greenland glaciers. Global warming will have a profound effect on the number of icebergs in any given area, and in fact may increase local production as ice shelves fragment and costal water temperatures change. At least one thousand icebergs drift in the Labrador Current south and east of Newfoundland and become a hazard to mariners above 41° north latitude. Navigation around icebergs is complicated by dense fog often present over the Grand Banks region and some difficulty of detecting icebergs with radar. U.S. and Canadian Coast Guards broadcast iceberg reports twice daily. The International Ice Patrol broadcast times and frequencies can be obtained by telephoning the International Ice Patrol in New London, Connecticut, at 860-441-2626 or online at http://www.uscg-iip.org.
Thunderstorms and Associated Weather Events
In the United States, squalls occur predominantly during the spring and summer in association with thunderstorms generated by towering cumulus clouds (Figure 83-12, online). The larger the cloud’s size (and radar echo), the more wind potential in the cloud. The taller the cloud (especially above 6096 m [20,000 feet]), the more energy potential for stronger winds. Most squall formations occur at night when cloud tops radiate heat back into space, enhancing their ability to grow. Squall-generated winds rarely strike without warning. A rapid fall in temperature is the precursor of a local storm. The bigger the drop, the stronger the winds, and rain that precedes the wind suggests stronger winds are coming. Occasionally, thunderstorms may form with the building of the cumulus cloud and not be associated with the typical rain, thunder, and lightning. These violent winds are called white squalls. The only warning may be the sudden appearance of a cold, shifting wind with an increase in velocity. Large sailboats, such as the clipper topsail schooner The Pride of Baltimore, have been knocked down and sunk by these unexpected powerful winds. In May 1986, a sudden storm rumbled across the Atlantic and unleashed its strength over a small patch of the Bermuda Triangle. With furious precision, its 70-knot winds overwhelmed the 97-ton, 34-m (110-foot) clipper ship heading home from Europe; survivors reported that the ship immediately barrel-rolled in the heavy wind and sank.
To avoid or prepare for the potential fury of a thunderstorm, the mariner must monitor local weather conditions. The National Oceanographic and Atmospheric Administration (NOAA) transmits on VHF-FM radio recorded messages, which are repeated every 4 to 6 minutes, and updated every 2 to 3 hours with the latest local information. The broadcasts usually can be received 20 to 60 miles from the transmitting antennas. The stations are identified on the radio channel display as WX-1, WX-2, and WX-3. Nationwide, more than 860 transmitters provide coverage to all 50 states and the adjacent marine areas. In many areas, the Coast Guard broadcasts weather information on VHF channel 22. Listings of schedules and frequencies for coastal and offshore weather broadcasts are available in a number of publications, including the Admiralty List of Radio Signals, Volume III, and Reed’s Nautical Almanac. Box 83-6 lists the definitions of weather warnings.
BOX 83-6 Storm Warnings
Many newer VHF radios have a weather-alert function known as Weather Watch. When the radio receives a special warning signal from NOAA, it sounds a special alerting tone, signifying an urgent NOAA weather forecast. Some radios automatically tune to the active weather channel, whereas others require manual tuning. In 1997, NOAA improved their severe weather warnings by encoding both the area affected by the severe weather and the nature of the severe weather (e.g., hurricane, winter storm, high wind, severe thunderstorm). Called SAME (Specific Area Message Encoding), this feature is intended to make weather warnings more customized so that users will not tune out possibly life-saving warnings. Offshore sailors can receive a variety of high-seas marine weather broadcasts with single-sideband (SSB) high-frequency (HF) radios. The U.S. Coast Guard broadcasts National Weather Service high-seas forecasts and storm warnings from each coast. All broadcasts are upper single side-band (USB) HF, with additional broadcasts on MF. The time schedules and frequencies for these transmissions can be found in the U.S. Defense Mapping Agency (DMA) Publication 177, Radio Navigation Signals. A less comprehensive list for specific locations can also be found in Reed’s Nautical Almanac. For schedules and much more information on National Weather Service marine products, visit http://www.nws.noaa.gov/om/marine/hfvoice.htm.
The U.S. Coast Guard sends a facsimile of a weather chart (weatherfax) from their East, Gulf, and West Coast communication centers. These marine weather charts are updated every 6 hours and available within 3.5 hours of the valid update time. Weatherfaxes contain graphic charts and forecasts compiled by the NWS; they are broadcast on high-frequency radio bands between 3.5 and 30.0 MHz. Lists of transmitters, frequencies, and times can be found in Reed’s Almanac or online at http://www.nws.noaa.gov/om/marine/fax.pdf. The NWS Internet page at http://www.nws.noaa.gov lists all weather charts broadcast over SSB. This site contains text forecasts, ocean current and surface analysis charts, wave charts, buoy reports, and prognosis charts (500-millibar [mb] charts) for weather outlooks of 12, 24, 36, 48, and 96 hours.
Onboard cellular connection, modem, and laptop computers are required to obtain this Internet information. Beyond cellular range, a dedicated weather fax unit or SSB, laptop, or weatherfax module is needed. Software can integrate weather forecasting with optimal routing for safety and sailing performance. Weather files (called GRIB files) can be downloaded and overlaid onto an electronic chart, and routing formulas can then be applied incorporating the specific boat’s known performance characteristics. Mariners can obtain sea state and weather data directly from NOAA buoys moored offshore by using the Internet. The National Data Buoy Center website is http://www.ndbc.noaa.gov; clicking on a specific station shows data gathered by the buoy in the preceding minutes.
Lightning
Lightning is one of nature’s most destructive phenomena (see Chapter 3). Boating, fishing, and swimming rank second only to playing sports on an open field as the most dangerous activities associated with a lightning strike. Although the odds that an individual will be struck and killed by lightning are 1 in 3 million, the odds for a boat being struck are greater. A cruising sailor in Florida, the lightning capital of America, can expect at least one strike to his or her boat in its lifetime.
Crew members may become part of the current path if they are in contact with, or come between, two different metal objects that are not interconnected (e.g., by grasping the stanchion or rigging while holding an aluminum steering wheel). The best protection in the event of a direct lightning strike, even if the boat is grounded and bonded, is to remain low in the boat (preferably in a cabin) and away from metal objects, wiring, and electric conductors (Box 83-7).
BOX 83-7 Protecting Crew from Lightning Injury
Other Weather Phenomena
The expected track error for a hurricane is 160 km (100 miles) on either side of the predicted track for each 24-hour forecast period. For a 72-hour forecast, an error of 480 km (300 miles) to the left or right of the predicted forecast track can be expected, and for 96 hours, 650 km (400 miles) to either side is applied. That would make the storm’s potential swath for destruction 800 miles wide, a considerable area to avoid if a vessel can only travel at 6 to 7 knots in storm conditions. In order to take meaningful evasive action, a hurricane needs to be monitored at least every 6 hours, which is the official forecast interval for the NWS Tropical Prediction Center. The National Hurricane Center in Coral Gables, Florida, provides advisory updates on developing tropical storms and hurricanes 24 hours a day. Voice recordings can be heard by calling 305-229-4483 or online at http://www.nhc.noaa.gov.
Emergency Communication and Distress Signals
VHF-FM Marine Radios
Cruising boats should have both a fixed mounted radio wired to the ship’s electrical system as well as a portable handheld VHF radio (Figure 83-13). After a knockdown or capsize, a handheld radio can operate independently of the ship’s radio antenna (the mast may have been lost or the antenna damaged). It is indispensable in SAR operations and helicopter evacuation, when one is required to be mobile and on the deck. Handheld radios can also be used to communicate with the mother ship from a dinghy, and are essential for communicating with rescue personnel from a life raft.
The new multichannel VHF survival radios are ideal for life raft or lifeboat use. They are waterproof, submersible to 3 m (10 feet), and float. The operating life with the lithium battery is a minimum of 8 hours. Normal handheld VHF radios sink, so they should be placed in a foam flotation case for protection. The most important VHF channel is channel 16, the distress and safety frequency (156.8 MHz). This calling frequency is used to initiate contact between any two vessels and is the only frequency constantly monitored by the Coast Guard. When a radio is not active on another channel, it should be left eavesdropping for distress calls on channel 16. Table 83-2 lists other VHF-FM channels often used by pleasure craft.
06 | Intership safety communications |
09 | Boater calling. Use this to initiate contact with other boats, and ship to shore (hailing channel). Commercial and noncommercial. |
12 | Port operations, traffic advisories |
13 | Intership navigation safety (bridge-to-bridge). Use this channel to contact a ship when there is danger of collision. |
14 | Port operations and some Coast Guard (CG) shore stations |
16 | International distress, safety, and calling |
22A | Working CG ships and shore stations, CG marine information broadcasts |
68, 69, 71 | Noncommercial intership and ship to coast |
70 | Digital selective calling (voice communications not allowed) |
72 | Noncommercial intership only |
78A | Noncommercial intership and ship to coast |
87A, B | Reserved for AIS digital communications |
WX 1 to 7 | NOAA (National Oceanic and Atmospheric Administration) Weather radio broadcasts on 162 MHz |
SSB-HF Radios
For communication offshore beyond the VHF range, a more powerful and elaborate SSB radio transmitter is required. The SSB’s clear advantage over the satellite phone is that a transmitted distress message will be heard by anyone who may be listening. Medium-frequency/high-frequency marine radio-telephone equipment operates between 2 and 26 MHz using SSB emissions. This equipment can also be used to receive high seas weather broadcasts (see discussion on thunderstorms and weather) and, in combination with a laptop computer and a special HF modem, can provide an easy and relatively inexpensive way to send and receive e-mail. The five principal SSB e-mail system providers for the recreational market are CruiseEmail, MarineNet, SailMail, WinLink, and SeaWave. Each provider uses a different software package with the Pactor-2 or Pactor-3 modem. E-mail is not just for social exchanges; it offers cruising boats a safety advantage for communicating safety-related data to boats around the world. Depending on the radiofrequency band and atmospheric conditions, communication range may be several thousand miles. See http://www.sailmail.org for excellent information about using the SSB for e-mail. Another popular use of the Internet is the phone program Skype. This service allows mariners to retrieve voicemail, and to make and receive telephone calls by connecting a microphone and headset to a laptop computer while connected to the Internet.
The international distress and calling frequency is 2182 kHz, located in the medium-frequency range. The frequency 2182 kHz is used for distances from 20 to 100 miles, day or night. The frequency 2670 kHz is designated for distress and safety communications with the U.S. Coast Guard. On the high-frequency bands, the frequencies 4125 kHz (Channel [Ch.] 450), 6215.5 kHz (Ch. 650), 8291.0 kHz (Ch. 850), 12,290.0 kHz (Ch. 1250), and 16,420 kHz (Ch. 1650) have all been designated for distress and safety calls. The high-frequency transceivers can call and receive voice and digital communications to and from anywhere in the world on land and sea. The Coast Guard transmits voice and weather information on various marine HF frequencies. The transmitters cover the Atlantic and Pacific Oceans, Caribbean, Gulf of Alaska, and Gulf of Mexico. Up-to-date schedules and frequencies are online at http://www.nws.noaa.gov/om/marine/hfvoice.htm and http://www.weather.gov/om/marine/hfvprod.htm. The best way to select an optimum emergency frequency is to listen to the quality of a radio broadcast. A station that your radio receives loud and clear will also provide good reception for your broadcast at that time. SSB is an excellent receiver for voice weather and weatherfax broadcasts, as discussed previously. Optimal use of a marine SSB radio requires instruction and practice. Marine Radio (ShipCom) in Mobile, Alabama is the sole provider of worldwide ship-to-shore HF SSB (and VHF in some locations) radiotelephone service in the United States. Complete information regarding these radiotelephone channels can be obtained by calling 251-666-5110 or at http://www.shipcom.com/services.
Global Maritime Distress and Safety System (GMDSS)
Since February 1, 1999, ships and coastal stations have not been required to monitor the traditional distress frequencies, such as VHF channel 16 or 2182 kHz. However, until the new Global Maritime Distress and Safety System (GMDSS) is implemented, the Coast Guard and many recreational and commercial ships will continue a radio watch on these frequencies. The GMDSS is a worldwide infrastructure, controlled from a shore-based communications center, to coordinate assistance to vessels in distress. This fully automated system uses satellite and digital communication techniques that require upgraded radio equipment and communication protocols. GMDSS simplifies routine communications at sea and facilitates regular weather forecasts, navigation warnings, and distress relays in the form of maritime safety information (MSI). DSC technology permits a VHF radio (with DSC capability) to call another radio selectively using digital messages, similar to the modem on a computer. As with a direct-dial telephone call on land, only the vessel called receives the initial message. Every vessel has its own unique Maritime Mobile Service Identity (MMSI) number. The radio must therefore be registered in order to be properly identified in an emergency or to be called directly by another boat using a DSC radio. To obtain and register a MMSI number, one goes to the Boat U.S. website at http://www.boatus.com/mmsi, or the Seas Tow website at http://www.seatow.com/boating_safety/mmsi. The vessel’s MMSI is registered in the Coast Guard’s national distress database together with information about the boat. For VHF radios, channel 70 (156.525 MHz) is authorized exclusively for distress alerts, safety announcements, and calling using DSC techniques. No other uses are permitted. Distress messages can be sent automatically with DSC radios. The vessel’s identity is permanently coded into the unit, and its position can be determined from the data output of a GPS receiver linked to the radio. On a DSC-equipped radio, the receiver sounds an alarm if it receives an “all ships call” (distress or otherwise), group call, or a call specifically to that vessel.
The GMDSS also uses satellite communication links, such as the International Maritime Satellite Organization (INMARSAT), to provide long-range global communication. Geostationary (geosynchronous) INMARSAT satellites cover the entire world between 70° north and 70° south latitude (there is no coverage at the poles, and spotty coverage in the Southern Ocean). The satellites completely orbit Earth over the equator at an altitude of 36,210 km (22,500 miles) during a period of 24 hours. They provide high-quality worldwide speech (voice is full duplex, just like a telephone), data, and facsimile communication as well as reliable distress alerting and follow-up communication within the GMDSS. Products in the INMARSAT Fleet Broadband systems vary in size, price, power consumption, and transmission speed. INMARSAT C is the gold standard for global marine data communication. It offers digital data messaging (no voice) and is the only two-way marine satellite data message system approved for GMDSS safety at sea. The hardware is small enough to fit in any boat. It can send a distress signal at the touch of a button and easily be integrated with a GPS receiver. Pressing the distress key sends a message with the ship’s identity and location to the nearest RCC. More information about GMDSS can be obtained from the Coast Guard website at http://www.navcen.uscg.gov/marcomms/gmdss/default.htm.
Emergency Beacons
Emergency position-indicating radio beacons (EPIRBs) are hand-held portable radio transmitters that can transmit signals interpreted as mayday calls (Figure 83-14). These signals are the satellite-linked equivalent of a 9-1-1 call for mariners in distress. In the absence of a marine radio, an EPIRB is the most important piece of signaling equipment. An EPIRB should be used only as a last resort when all other means of communication have failed, and when there is a life-threatening emergency. It should be located in a readily accessible location.
The first-generation class A and class B beacons are no longer in use, having been replaced by the superior Categories 1 and 2 EPIRBs and PLBs. The second-generation 406-MHz EPIRB provides the most reliable worldwide coverage. Satellites with 406-MHz transponders can store the signal in memory until a ground station is in view and then can retransmit the signal. The distress signal is quickly relayed to a ground station called a local user terminal (LUT), passed on to the mission control center (MCC), and relayed to the appropriate maritime rescue coordination center (MRCC). The satellites are able to compute the position of the beacon within 2 km (1.24 miles). The 406-MHz EPIRB transmits a digital signal with a unique identification code, which can be instantly identified through a NOAA encoded-transmission program. If the unit is properly registered with NOAA, vital information regarding the vessel can be passed on to SAR units. All registered EPIRBs are issued a dated decal that provides proof of registration and includes a unique 15-character hexadecimal code, registration expiration date, and the vessel’s eight-digit registration code. Every owner who registers an EPIRB receives a sticker from NOAA printed with the beacon’s registration number. This number should be verified to match the ID number on the beacon before it is attached to the unit. If the numbers do not match, the beacon is not properly registered. According to NOAA, 30% of beacons are never registered by their owners. Registration can be done online at http://www.beaconregistration.noaa.gov/.
Visual and Sound Distress Signals
The simplest signaling strategies and devices are often overlooked. Box 83-8 lists some simple signaling techniques. Visual pyrotechnic distress signals have a replacement interval of 42 months from the date of manufacture and are labeled with expiration date somewhere on the device. Expired flares can be kept onboard as spares, but will not be counted in the vessel’s inventory.
BOX 83-8 Visual and Sound Distress Signals at Sea
Handheld flares have varying effectiveness for attracting attention during daylight (Figure 83-15). Luminosity ratings range from 500 to 15,000 candlepower. The lowest rated flares are virtually invisible in daylight at 0.4 km (0.25 mile), and the highest rated flares are only slightly more visible. For daytime use, orange smoke devices are the most effective way to attract attention. SOLAS-graded smoke canisters are superior to hand-held smoke flares. They float and emit orange smoke for 3 to 4 minutes. Immediately after ignition, throw the canister in the water downwind of the craft. Smoke signals have a visible range of 1.6 to 5 km (1 to 3 miles) in daylight, depending on the wind. Helicopter pilots find smoke signals highly visible and especially useful to indicate the strength and direction of the wind at sea level (Figure 83-16).
Red meteor (aerial) hand-launched or pistol-launched flares (Figure 83-17) should be also launched downwind approximately 80 degrees above the horizon. These flares are less effective than parachute flares because burn time is only 5 to 9 seconds. It is best to fire them in sequence—the first to attract attention and the second about 10 seconds later to confirm distress and general position. Because of their generally lower altitude and brightness, visibility is limited to 5 to 8 km (3 to 5 miles) at night. Because of these limitations, these flares are best suited for guiding nearby rescuers to the boat, after it has been spotted.
The Decision to Abandon Ship
How to abandon ship
Each crew member should have an easily accessible prepacked waterproof bag containing extra dry clothing, personal medications, passport, prescription glasses and sunglasses, personal strobe, safety harness and tether, wallet, and any other personal valuables and necessities. If the ship’s bag has been properly stocked, little else is needed from the sinking ship except synthetic blankets (not down sleeping bags, which never dry), jerry jugs of fresh water (two-thirds full so they float), extra food (especially high-carbohydrate sports bars), and navigation tools. If time permits, additional communication and signaling equipment should be collected from the stricken vessel, even if equipment was already placed in the bag or raft. This includes a 406-MHz EPIRB, a SART (if available), and a waterproof handheld VHF radio. In a life raft, one can never have too many EPIRBs, radios, flares, and fishhooks (Box 83-9).
BOX 83-9 Abandon Ship Bag for Ocean Passage
Signals and Communication
Protection
Preparation for Rescue: Life in the Raft
Water
Life rafts, if they have any water rations, generally carry 1 L (2 pints) per person in 125-mL sachets; some rafts only carry as little as a pint per person. It is recommended that castaways should restrict water intake to about 600 mL (1.25 pints) daily, unless supplies are plentiful; however, this is not the absolute minimal requirement. Analysis of protracted voyages in life rafts during World War II found that the critical amount of potable water for survival was 120 to 240 mL (4 to 8 oz) per day. This figure was based on the relation between survival rates and availability of water in 121 life raft voyages involving 3616 men. Reviewing 1314 men at risk who drank only 120 to 240 mL (4 to 8 oz), 96 (7%) died. Consumption under 120 mL (4 oz) resulted in much higher death rates. In a subgroup of 62 men who were adrift in two boats for periods of 37 and 49 days with water rations between 120 to 240 mL (4 to 8 oz), only one died.2
Food
Certain fish are inherently poisonous (see Chapter 72); these are usually located around shoals and reefs in shallow waters. These include pufferfish, porcupine fish, and the ocean sunfish (Mola). Any fish with spines or bristles instead of scales should not be eaten.
Plankton also has a high cellulose and chitin content and cannot be ingested in large quantities. Some toxic plankton are difficult to detect. The red tides that cause paralytic shellfish poisoning result from the blooms of dinoflagellates (see Chapter 72).
Rescue and Evacuation of the Sick and Injured
Amver
The Automated Mutual Assistance Vessel Rescue System (Amver) provides resources to help any vessel in distress on the high seas. Amver, sponsored by the U.S. Coast Guard, is a unique, computer-based, and voluntary global ship reporting system used worldwide by search and rescue authorities to arrange for assistance to persons in distress at sea. With Amver, rescue coordinators can identify participating ships in the area of distress and divert the best-suited ship or ships to respond. Some 12,000 ships from over 140 nations participate in Amver. An average of over 2800 ships are on the Amver plot each day. These merchant ships are not designed for SAR and their crews may not be trained for recovering survivors from small boats or life rafts under storm conditions. Offshore, however, they may be the only rescue option. For more details see http://www.amver.com/.
Helicopter Evacuation
The transfer device is either a rescue basket or a Stokes litter (Figure 83-18). Selection depends on the victim’s medical condition and the necessity to remain horizontal during the hoist. The horizontal position is particularly important for persons with spine injuries or severe hypothermia. A basket is the preferred device for lifting. It is easy to enter, especially in rough weather, and has positive flotation so that it will not sink. Just climb in and fasten the straps. The basket will settle on the sea surface, enabling someone in the water to float into it.
The helicopter has enormous potential for safe and effective SAR. The U.S. Coast Guard operates two types of helicopters (Table 83-3).
Model | HH-60 Jayhawk | HH-65 Dolphin |
---|---|---|
Crew | Pilot, co-pilot, rescue swimmer, flight mechanic | |
Maximum takeoff weight | 9926 kg (21,884 lb) | 4300 kg (9480 lb) |
Engines | 2 × 1890-HP gas turbines | 2 × 934-HP gas turbines |
Maximum speed | 180 knots | 165 knots |
Range | 700 NM | 365 NM |
Duration | 6.5 hours | 3.5 hours |
Capacity* | 4+6 | 4+5 |
* Capacity refers to additional people (i.e., passengers).
To view an excellent instructional video for recreational boaters on helicopter rescue, go to the Cruising Club of America web site: http://www.cruisingclub.org/seamanship/seamanship_safety_heli.htm.