16 Retinal Degenerations
Retinal Dystrophies
Age-related Macular Degeneration
Treatment of Age-related Choroidal Neovascularization
Idiopathic Polypoidal Chorioretinopathy (IPCV)
Inherited Central Receptor (Macular) Dystrophies
Inherited Retinal Dystrophies with Generalized Retinal Involvement
Progressive Retinal Dystrophies
Normal vision depends on the proper functioning of the macula which, when disturbed by disease, affects central vision and often causes the patient to present for the first time. Central distortion of vision (metamorphopsia) or minification of the image are pathognomonic for macular disease. Other symptoms apart from loss of acuity include photophobia, glare and dazzle (which can be severe), poor colour perception, night-blindness and a central or paracentral scotoma. Macular disease in infants frequently presents as congenital nystagmus.
Classifying macular disease by the anatomical layer that is primarily affected—choriocapillaris, Bruch’s membrane, retinal pigment epithelium (RPE), photoreceptors or neuroretina—is a commonly used and helpful way to conceptualize and diagnose retinal problems. Stereoscopic examination of the fundus with an indirect biomicroscopy lens through a dilated pupil is essential to assess the location of a lesion within the retina and its relationship to the superficial retinal vessels. However, although examination can infer the initial site of the lesion in many retinal diseases the primary defect cannot be precisely localized to a single cell layer as defects in one retinal layer produce changes in adjacent structures. For example, retinitis pigmentosa is associated with photoreceptor degeneration and also RPE and choriocapillaris atrophy with later neuroretinal degeneration and vascular attenuation. Our understanding of the pathogenesis of various retinal diseases has improved in recent years with advances in molecular genetics and cell biology and some diseases that were thought to affect, for example, primarily the photoreceptors have now been shown to have their defect in the RPE. Fundus photography, fundus fluorescein angiography (FFA), indocyanine green (ICG) angiography, optical coherence tomography (OCT) and autofluorescence (AF) imaging are valuable investigations for diagnosis and documentation. Many macular lesions are small and produce scotomas that are difficult to demonstrate; an Amsler grid can help in these cases. Electrodiagnostic tests and psychophysical testing are time consuming but very useful for diagnosing, documenting and assessing the prognosis of inherited retinal disease (see Ch. 1).
Occasionally deciding whether visual loss is caused by macular or optic nerve disease can be difficult as both reduce acuity and colour vision and can cause central scotomas. Retinal lesions tend to produce a ‘positive’ scotoma in which patients are aware of dark obscuration or something in front of their vision in contrast to the ‘negative’ scotoma of neurological disease (for example, patients do not notice a hemianopic field defect as ‘dark’). An afferent pupillary defect indicates optic nerve disease unless the macular lesion is extensive. Another useful and easily performed test is the photostress test in which recovery of visual acuity following dazzle with a bright light is delayed with macular lesions whereas vision recovers normally with optic nerve disease. Retinal lesions produce a blue-yellow pattern of colour deficit in contrast to the red-green pattern of optic nerve disease (see Ch. 19). These clinical tests are subjective, however, and less reliable than pattern electroretinography (PERG) which in most cases can distinguish macular from optic nerve disease (see Ch. 1).
AGE-RELATED MACULAR DEGENERATION
PATHOGENESIS OF AMD
AMD appears to result from defects in the RPE cell–Bruch’s membrane–choriocapillaris complex and is thought to be a collection of heterogeneous disorders associated with multiple genetic and environmental factors. Much current research centres on defects in the RPE cell and Bruch’s membrane (see Ch. 13). In normal ageing progressive thickening of Bruch’s membrane occurs with accumulation of lipids, noncollagenous proteins and other extracellular material such as advanced glycation endproducts, collagen and elastin changes and increased calcification. By the fourth decade all eyes show accumulation of membranous debris within both collagenous layers. With time, a second series of changes is seen with deposition of diffuse material both between the RPE and its basement membrane (basal linear deposit) and between the basement membrane and the inner collagenous layer (basal laminar deposit). The latter can become extensive with a complex morphology and may be confused with diffuse or soft drusen, drusen being focal accumulations between the RPE basement membrane and the inner collagenous layer of Bruch’s membrane. Lipofuscin accumulates with age in the RPE cell from metabolism of photoreceptor outer segments from oxidative damage or RPE overloading by failed photoreceptors. The specific mechanisms underlying these changes are not fully understood, but it is thought that the resultant increase in thickness and reduction in molecular transport through Bruch’s membrane and the RPE cell may contribute to photoreceptor cell death and AMD. In addition, anatomical changes and delayed filling on fluorescein angiography are seen in the choroidal circulation.
DRUSEN AND ATROPHIC MACULAR CHANGES
Drusen lie in Bruch’s membrane and stain progressively during fluorescein angiography. They are assessed more easily by fluorescein angiography than by fundoscopy as angiography shows RPE atrophy more clearly. Different drusen staining patterns emerge during angiography. Conventionally, drusen that stain readily are taken to be ‘hydrophilic’, whereas those that do not are ‘hydrophobic’. Hydrophilic drusen are more proteinaceous and are associated with an increased risk of choroidal neovascularization; hydrophobic drusen have increased lipid and are more associated with PED. Other factors, such as drusen size and extracellular matrix characteristics probably influence staining too. However, it is the extent of RPE atrophy seen as window defects (see Ch. 13) on angiography or as blackness on autofluorescence imaging—rather than drusen—that better reflects visual loss.
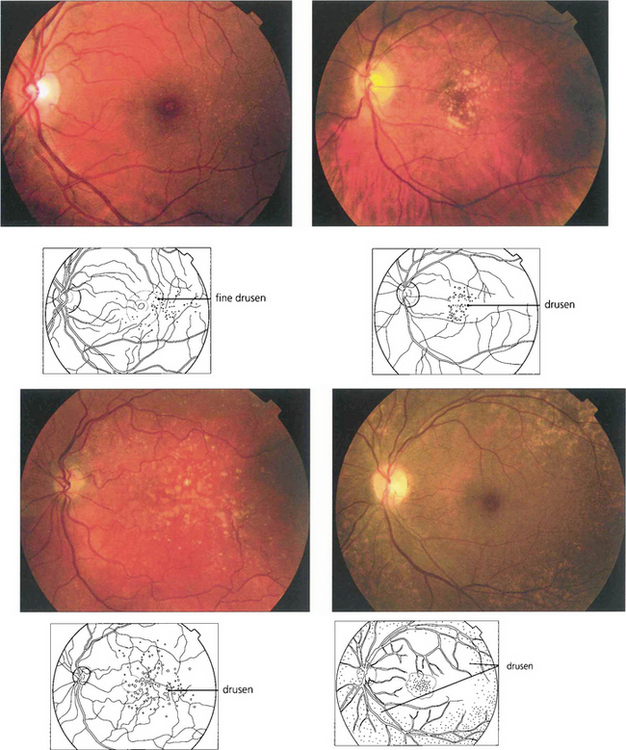
Fig. 16.3 Drusen come in various patterns, shapes and sizes with variable amounts of RPE change. Some patients develop widespread drusen throughout the posterior pole.
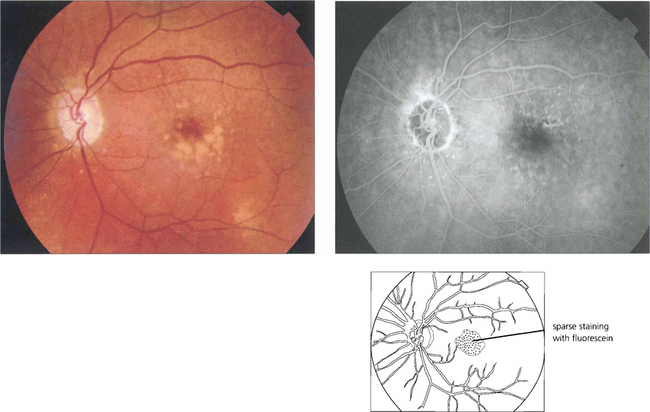
Fig. 16.4 The drusen in this patient’s macular area are relatively hydrophobic, failing to stain as angiography progresses.
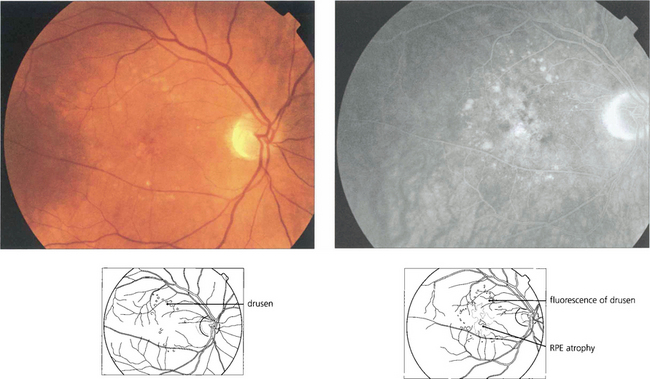
Fig. 16.5 This patient has more hydrophilic drusen. Note the submacular hyperfluorescence, which probably denotes early occult choroidal neovascularization.
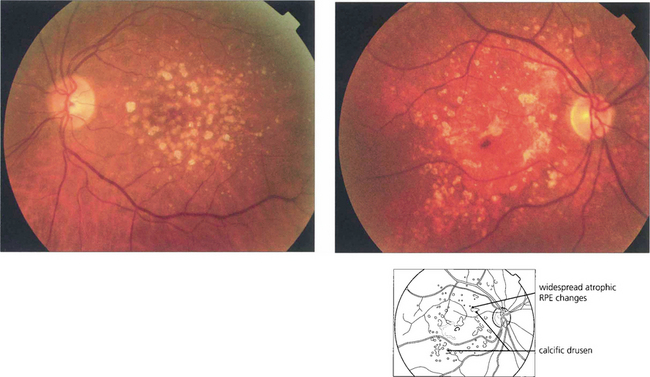
Fig. 16.6 Calcific drusen have a flat, glistening, white and refractile appearance (left). These eyes tend to lose vision from RPE atrophy rather than neovascularization, as seen in the more gross example (right).
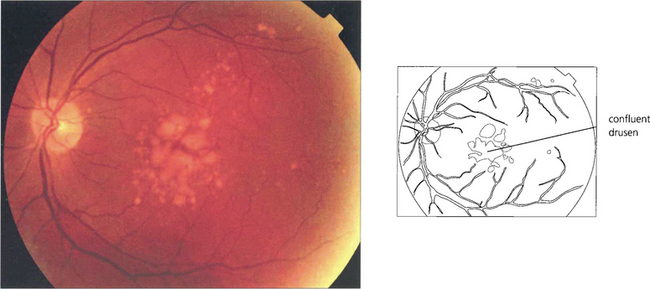
Fig. 16.7 This patient has a large area of confluent drusen under the macula. Such patients have a considerable risk of developing choroidal neovascular lesions.
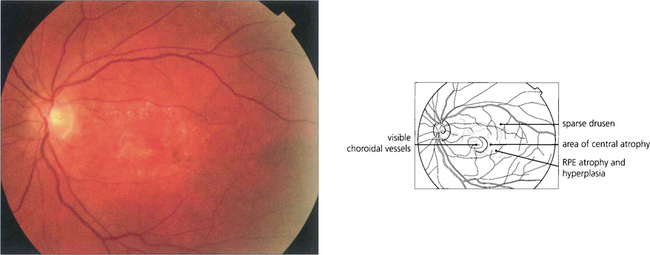
Fig. 16.8 The degree of RPE atrophy varies considerably. This patient has small, sparse drusen, subretinal pigmentation from RPE hyperplasia and a central–paracentral RPE defect, accounting for an acuity of 20/100 and a paracentral scotoma.
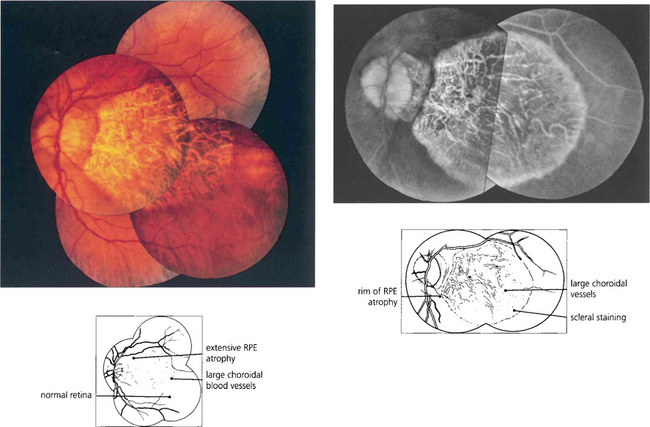
Fig. 16.9 Central areolar choroidal atrophy can be dominantly inherited but it is also a phenotypic variant of AMD in which there is a sharp circumscribed demarcation line between normal and abnormal retina with central atrophy of the choriocapillaris, RPE and photoreceptors revealing large choroidal vessels in the base (left). These lesions start in the macular area and gradually increase in extent over time with severe loss of visual acuity. Drusen are not normally seen. (right) Angiogram of the same patient shows the large window defect due to loss of the RPE and choriocapillaris. Large choroidal vessels are seen in the base and fill normally. Note the relative hyperfluorescence of the rim of the lesion from scleral staining (see Ch. 13).
RETINAL PIGMENT EPITHELIAL DETACHMENT
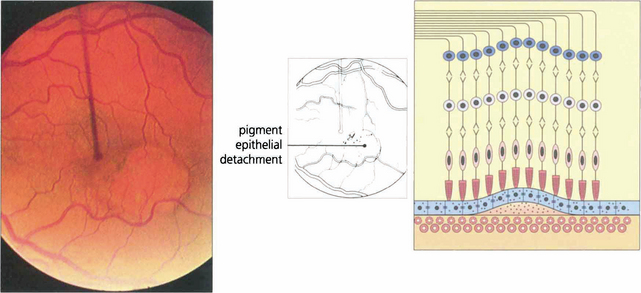
Fig. 16.10 Colour photograph showing the well circumscribed elevation of the macula, which has a more prominent and thicker appearance than that seen with central serous retinopathy (see Fig. 16.38). In elderly patients other signs of macular degeneration such as drusen, pigmentation and atrophy are frequently seen. Any surrounding lipid exudate or haemorrhage strongly suggests choroidal neovascularization within the lesion.
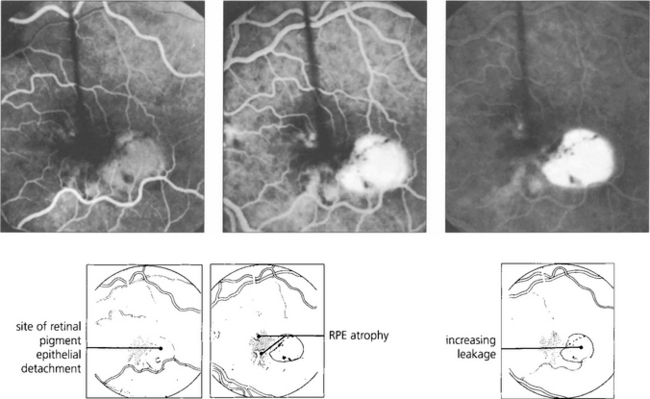
Fig. 16.11 The fluorescein angiogram shows early diffuse leakage which increases in intensity as the run progresses but remains localized to the area of the lesion. Other degenerative changes in the RPE are often seen.
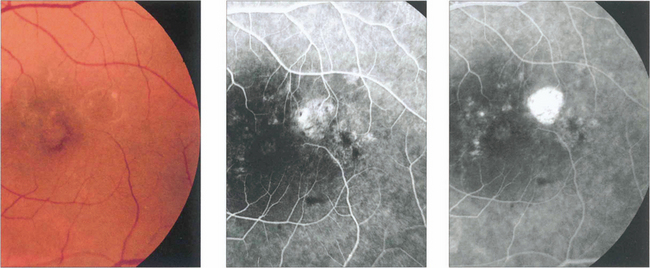
Fig. 16.12 Elderly patients may have an underlying neovascular membrane, which is indicated by any unevenness of staining or hyperfluorescence within the lesion.
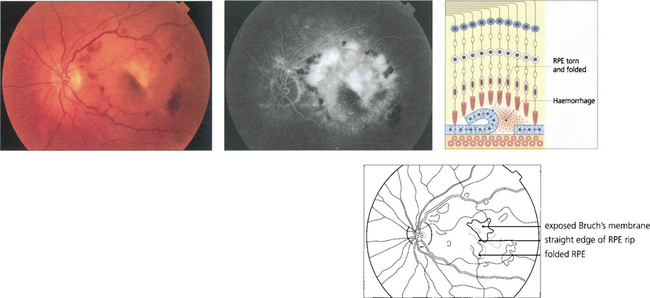
Fig. 16.13 Excessive tension within the PED causes it to rip and fold back on itself. This is often associated with haemorrhage into the lesion and acute loss of acuity in the eye. Characteristically RPE rips show a dark area where the RPE has folded back on itself separated by a straight line from the area of denuded RPE.
By courtesy of Ms H Jackson.
CHOROIDAL NEOVASCULAR MEMBRANES
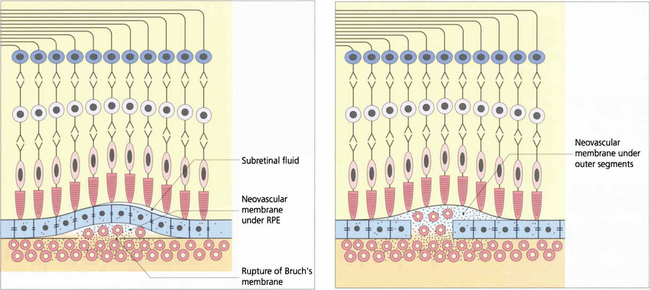
Fig. 16.14 CNV can grow between Bruch’s membrane and the RPE (type 1) or in the subretinal space between the RPE and photoreceptors (type 2); combinations of these occur as well. The appearance of CNV on angiography depends on the amount of haemorrhage, pigment hyperplasia and RPE atrophy. Based on FFA findings, choroidal neovascular membranes (CNV) are divided into classical and occult forms. Classical CNV is defined as well demarcated areas of hyperfluorescence that can be seen in the early phase of fluorescein angiography with progressive leakage of dye obscuring the boundaries of the CNV as angiography progresses; these may correspond to type 2 membranes. Occult CNV is either a fibrovascular PED with areas of irregular elevation of RPE producing an area of stippled hyperfluorescence as the angiogram progresses or late leakage from an undetermined source; these may correspond to type 1 membranes. An alternative explanation of the different angiographic appearances is that a classical CNV represents a type 1 membrane with overlying RPE atrophy. Further clinical pathological studies are needed.
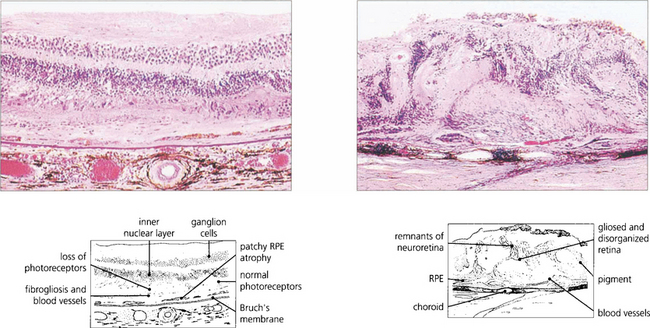
Fig. 16.15 Serial sections of eyes with drusen have shown that neovascularization of drusen in the posterior pole is not uncommon and that only a minority of these lesions progress to a clinically apparent CNV. Pathology of end-stage disciform macular degeneration shows a layer of vascularized fibrous tissue replacing the photoreceptors (left). In a gross example (right) the retina is completely destroyed by an elevated mass of vascularized fibroglial tissue.
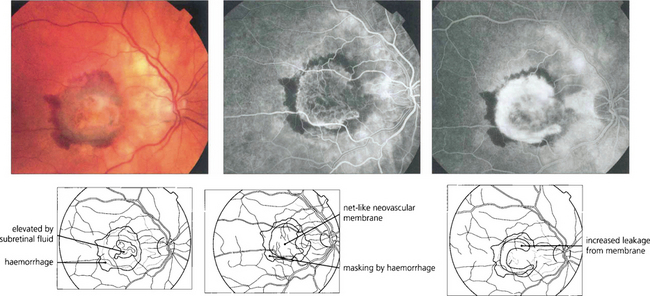
Fig. 16.16 The typical classical CNV lesion, no matter what the aetiology, consists of subretinal elevation either beneath or adjacent to the fovea. Recent lesions often have a pale, raised appearance with variable amounts of lipid or haemorrhage. A subretinal haemorrhage in the macular area should always raise the suspicion of a neovascular cause. Presenting symptoms are either metamorphopsia or blurring of vision, depending on the size and site of the lesion. Early-phase fluorescein angiography of the same lesion shows the classical appearance of a neovascular complex lying beneath the retina which, because it is derived from the choroid, fills early in angiography. Individual vessels in the neovascular membrane may be identified in the earliest stages as a net-like membrane. In common with other neovascular tissue, the capillaries in the neovascular membrane have loose endothelial cell junctions and in late stages the membrane shows intense leakage into the adjacent retina, blurring the membrane. These lesions are amenable to photodynamic treatment.
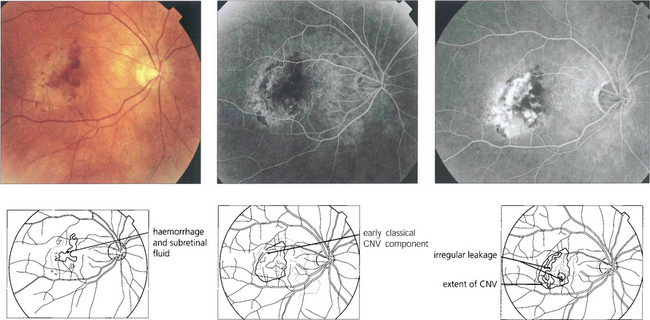
Fig. 16.17 Occult CNV membranes are more common than classical lesions. This patient presented with distortion and blurring of vision to 20/80. Angiography shows an irregular leakage in the lesion, staining intensively in the later phases. These lesions do not benefit as much from photodynamic treatment.
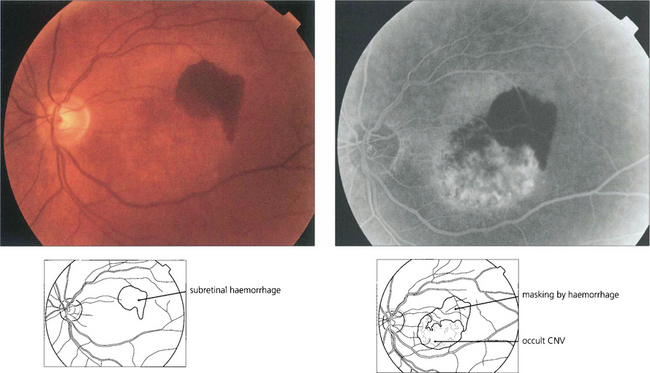
Fig. 16.18 CNV is often associated with subretinal haemorrhage which may produce a sudden drop in visual acuity causing the patient to present. In this situation haemorrhage may mask the true extent of the membrane and fluorescein angiography should be postponed until sufficient haemorrhage has been absorbed for visualization. ICG angiography may help in these circumstances as it reveals the extent of the membrane despite the overlying haemorrhage.
OTHER CAUSES OF CHOROIDAL NEOVASCULAR MEMBRANES
ANGIOID STREAKS
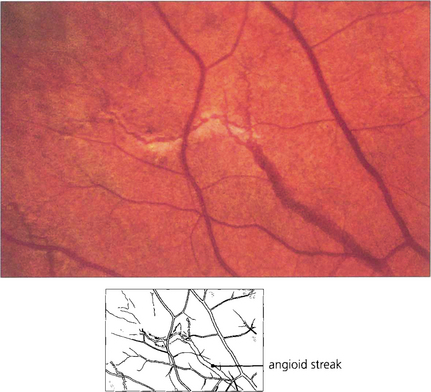
Fig. 16.21 Angioid streaks are splits in Bruch’s membrane. They appear as linear fractures extending from the optic disc and ramifying towards the periphery mimicking retinal blood vessels. They are usually reddish brown but may show degenerative pigmentary changes. Their appearance on angiography depends on the degree of associated pigmentary disturbance and RPE loss. The majority of streaks are hyperfluorescent, although early in the run they may mask background choroidal fluorescence. Subretinal neovascularization in the papillomacular region or alongside the optic disc is a frequent complication. Angioid streaks occur as an isolated ocular finding or with systemic associations which include elastic tissue disorders such as pseudoxanthoma elasticum and Ehlers–Danlos syndrome.
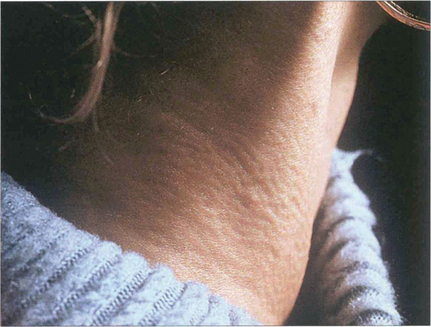
Fig. 16.22 Pseudoxanthoma elasticum is a recessively inherited disorder affecting connective tissue elastic fibres. Patients have cutaneous, ocular and vascular manifestations and suffer premature hypertension, atheroma, cardiac valve disorders and gastrointestinal haemorrhage. This patient has the characteristic ‘chicken skin’ changes on her neck, which she disguises by wearing a polo-neck shirt.
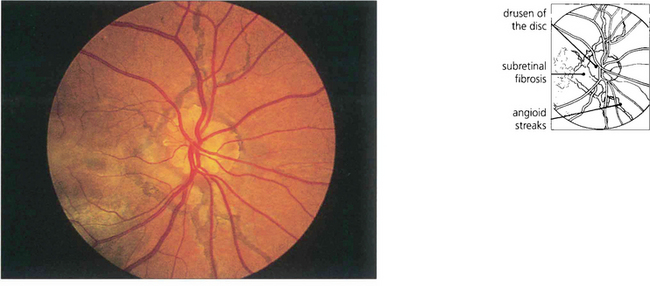
Fig. 16.23 Remarkably conspicuous angioid streaks radiate from the patient’s optic disc which also has the well recognized association of pronounced optic disc drusen. Juxtapapillary and subretinal fibrosis from neovascularization can be seen underlying the macula. This patient has widespread RPE changes within and adjacent to areas of subretinal neovascularization; visual prognosis is poor.
By courtesy Mr R K Blach.
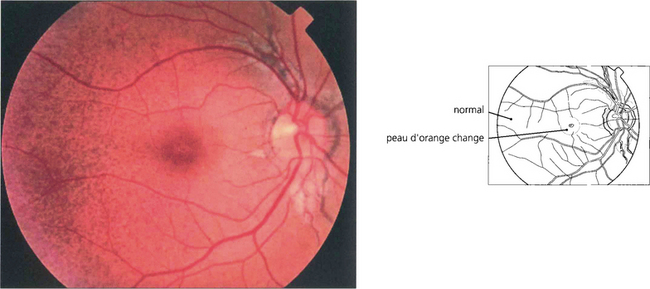
Fig. 16.24 In patients with pseudoxanthoma elasticum, Bruch’s membrane and the RPE may also be altered, giving the fundus a slightly mottled, yellowish and refractile appearance known as ‘peau d’orange’. This is most noticeable in the posterior pole and contrasts with the normal appearing periphery.
CHOROIDAL RUPTURE
‘Choroidal rupture’ is caused by severe blunt injury to the eye but the appearance is in fact due to rupture of Bruch’s membrane (see Ch. 14). CNV can develop some time later in lesions extending into the macular area.
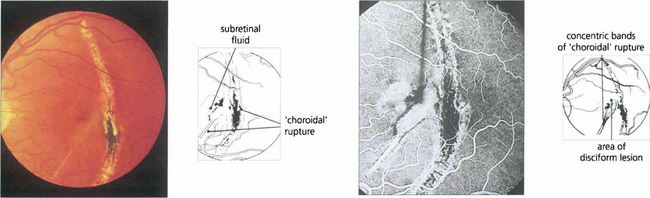
Fig. 16.25 In this patient, rupture is seen as a typical chorioretinal scar lying concentric to the optic disc with fresh, pale, submacular fluid that has caused recent onset of blurring and distorted vision. Fluorescein angiography demonstrates the extent of the RPE and choriocapillaris disruption with a subfoveal CNV. Photodynamic therapy has a potential role in the managment of these patients.
OCULAR HISTOPLASMOSIS AND PUNCTATE INNER CHOROIDOPATHY
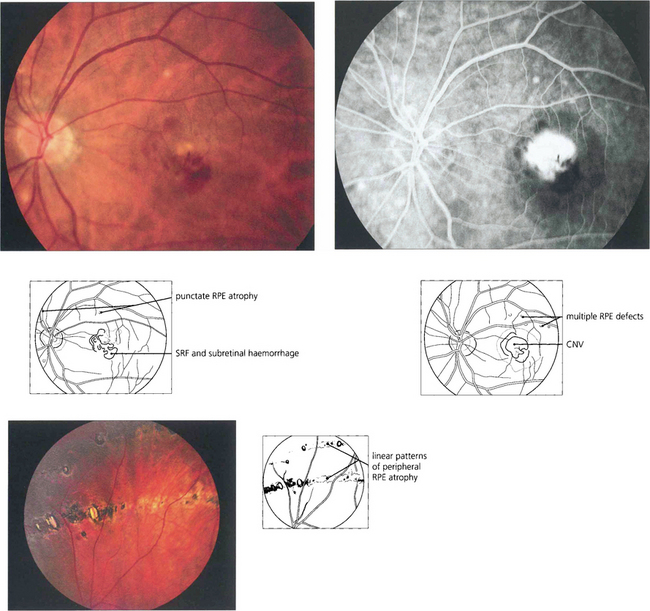
Fig. 16.26 The typical appearance of the fundus comprises the triad of atrophic changes around the optic disc, localized punched-out areas of pigment epithelial atrophy in the posterior pole and atrophic, usually linear, RPE lesions in the equatorial retina (bottom left). Atrophic changes in the macular region carry a substantial risk of developing CNV, typically in early middle age.
PERIPAPILLARY CNV
Choroidal neovascularization may develop adjacent to the optic disc, either spontaneously or in association with disc abnormalities such as tilting or chronic pathological disc swelling or drusen of the disc (see Ch. 17).
MYOPIC MACULAR DEGENERATION
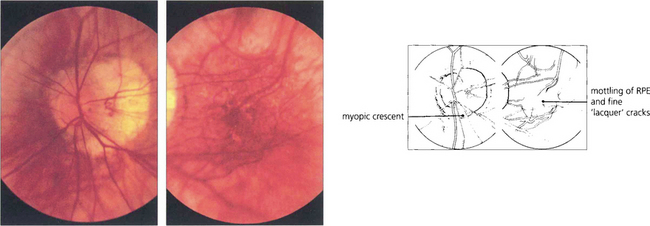
Fig. 16.29 High myopes have an increased risk of developing atrophic macular degeneration—seen as chorioretinal thinning or atrophy, posterior staphylomata and splits in Bruch’s membrane termed ‘lacquer’ cracks—and also peripheral lattice degeneration. This patient shows a large myopic crescent on the temporal side of the optic disc. Retinal vessels are pulled straight as they enter the enlarged posterior pole. In the macula there is patchy pigmentary disturbance and fine cracks in Bruch’s membrane.
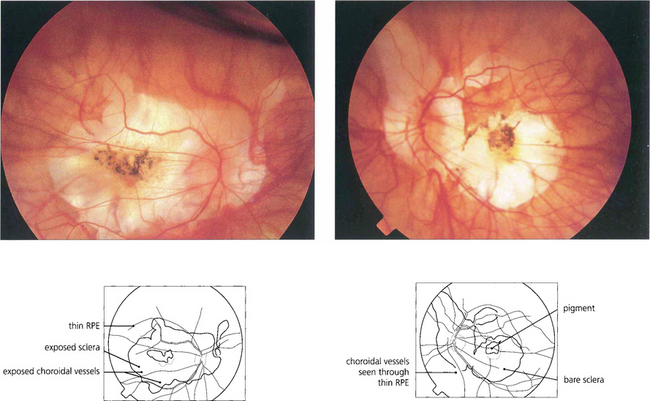
Fig. 16.30 This patient shows marked myopic chorioretinal atrophy in both eyes with exposed sclera at each posterior pole.
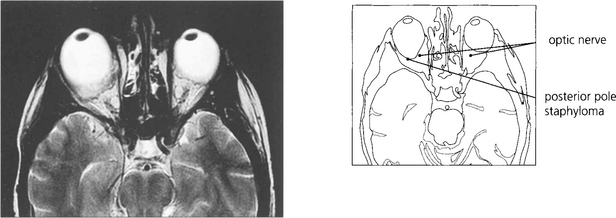
Fig. 16.31 Magnetic resonance imaging demonstrates a large posterior pole staphyloma extending posteriorly to the optic disc in the right eye.