CHAPTER 18 RESUSCITATION FLUIDS
The practice of giving intravenous fluids or medication has been used since the 1600s. Slow progress was made after William Harvey provided a modern description of the circulatory system in 1638. William O’Shaughnessy theorized that patients suffering from volume loss secondary to cholera would benefit from restoration of blood to its natural specific gravity. This became the first concept of contemporary intravenous fluid therapy. Thomas Latta was credited with actually applying O’Shaughnessy’s theory and treating victims of cholera in 1832. Later in the century, Sydney Ringer described a physiologic solution with a focus on electrolyte concentrations in his animal models. Despite these early trials, application of these concepts was criticized and largely forgotten until the 20th century. The advent of modern surgery renewed interest in resuscitation as therapy to maintain intravascular volume.
CLASSES OF SHOCK
Shock is inadequate tissue perfusion. The humoral sympathetic response to shock causes vasoconstriction in the clinical appearance of the patient. These findings were observed by Cannon when he correlated the volume of blood loss with the patient’s clinical signs, symptoms, and blood pressure. His findings have since been validated with repeated observational studies in humans, as well as experimental studies in animals. The classes of shock are now well defined, and provide a quick means of assessing a patient’s blood loss and the level of resuscitation required (Table 1).
AUTORESUSCITATION
Vasoconstriction and Reduction of Capillary Hydrostatic Pressure
The subsequent decrease in capillary hydrostatic pressure reduces the volume of plasma lost from the vasculature into the interstitial space, and increases return of interstitial fluid into the vascular space. This is an additional method of increasing venous return to the heart. Given the large capacitance of the venous system, larger volumes of circulating blood are stored within the veins compared to the arteries. According to the Frank-Starling laws of cardiac performance, the cardiac output is directly proportional to the venous return to the heart (preload) (Figure 1). As venous return decreases, so does cardiac output, and thereby arterial pressure to perfuse vital organs.
Hormonal Response
The reduction of capillary hydrostatic pressure and the hormonal response increase venous return during the first 1–2 hours following injury, but this may be inconsequential in the setting of severe hemorrhage. Cessation or slowing of ongoing hemorrhage also contributes to a spontaneous rise in blood pressure by limiting ongoing fluid losses. The remainder of the response is carried out through vasoconstriction and the redistribution of blood flow, accounting for the majority of increased venous return. If prolonged, this can have a damaging, and sometimes irreversible effect upon the tissues supplied by the splanchnic circulation. This will result in ischemia and potential reperfusion injury.
Phases of Shock
Combined, the aforementioned physiologic effects produce the classic signs and symptoms exhibited by patients in hypovolemic shock. The progression from compensated to uncompensated shock gives rise to the physical findings of the systemic insult (Figure 2). Initially, compensated shock affects the periphery, manifesting the symptoms of cool extremities, cyanosis, mottling of the skin, and decreased capillary reperfusion (blanching). As the severity of shock progresses, vasoconstriction affects the torso, producing oliguria as the main clinical sign. Finally, in severe classes of shock, perfusion of the heart and brain are affected, producing cardiac dysrhythmias and a reduced level of consciousness. Over the hours following injury, these mechanisms are in constant flux, in efforts to restore homeostasis to the injured system.
HYPOTENSIVE RESUSCITATION
Choice of Fluids
The use of crystalloids versus colloids has been an ongoing debate for decades. In the Vietnam War, isotonic crystalloids were used when laboratory work from the 1960s by Shires and others showed larger volume resuscitation with isotonic crystalloids resulted in the best survival. They also noted that extracellular fluid redistributed into both intravascular and intracellular spaces during shock, and rapid correction of this extracellular deficit required an infusion of a 3:1 ratio of crystalloid fluid to blood loss. Figure 3 shows the influence of fluids on the extracellular fluid compartments. Using this resuscitation strategy, the rate of mortality and acute renal failure decreased but a new entity of shock lung, now better known as acute respiratory distress syndrome (ARDS), was discovered. In reviewing studies comparing crystalloids and colloids with respect to pleural effusions and pulmonary dysfunction, two trials reported no differences. Two other series showed more pulmonary complications among patients resuscitated with colloid. When mortality was used as an endpoint, the use of crystalloids in trauma patients was associated with increased survival.
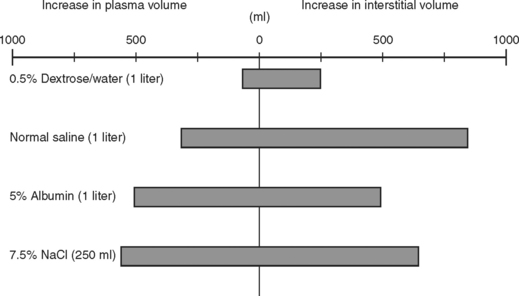
(Data from Imm A, Carlson RW: Fluid resuscitation in circulatory shock. Crit Care Clin 9:313, 1993.)