Chapter 15 Respiratory management in neurological rehabilitation
Introducton
Respiratory problems are not confined to respiratory patients. Every patient has the potential to develop respiratory dysfunction. This is particularly true for patients with neurological disorders. As well as problems with reduced central drive or neuromuscular weakness associated with pathology and trauma, many neurological patients are susceptible to respiratory infections through immobility or aspiration (see Table 15.1). As respiratory dysfunction can be life-threatening, it makes sense for every physiotherapist to be competent to conduct an assessment of the respiratory system, to be aware of the common problems with which patients present, and to have a basic toolkit of interventions designed to manage such problems. This chapter will cover the areas of respiratory assessment, problem recognition and respiratory physiotherapy management in neurological patients.
Table 15.1 Clinical course for some neurological conditions commonly associated with respiratory problems
Disorder | Clinical Course | Prevalence of Respiratory Involvement |
---|---|---|
CNS | ||
Multiple sclerosis | Relapsing | Pulmonary function impaired in 63%; respiratory failure or infection causes death in 5% |
Parkinson’s disease | Slowly progressive | Pneumonia accounts for 20% of deaths, possibly from bulbar or upper airway muscle involvement and impaired cough |
Spinal cord | ||
Trauma | Permanent | High lesions (C1–3) usually require long-term ventilation |
Motor neurone | ||
Postpolio syndrome | Very slowly progressive | Respiratory impairment usually only in those with initial respiratory muscle involvement |
Motor neurone disease | Progressive | Death almost uniformly due to respiratory complications |
Motor nerves | ||
Guillain-Barré syndrome | Slowly reversible | Respiratory failure in 28% |
Charcot–Marie–Tooth | Very slowly progressive | 96–100% have prolonged phrenic nerve conduction; 30% have vital capacity <80% predicted |
Neuromuscular junction | ||
Myasthenia gravis | Reversible | Aspiration pneumonia gives rise to crises with 6% mortality |
Botulism | Slowly reversible | 8% mortality due to respiratory failure |
Muscle | ||
Duchenne muscular dystrophy | Progressive | Respiratory failure is major cause of death |
(Adapted from Aboussouan 2005, with permission.)
Respiratory assessment
A comprehensive respiratory assessment as outlined in Box 15.1 is only possible in a patient in a stable situation. If any ‘red flags’ are noticed (see Box 15.2), the assessment may need to be adapted and shortened. Although it is generally recognized that neurological disease may result in respiratory dysfunction, its presentation in such patients may be atypical, because of wider effects of the underlying condition (Polkey et al., 1999). The tests starred (*) in Box 15.1 will now be described further in relation to neurological patients.
Box 15.1 Elements of a respiratory assessment
General end-of-bed observations:
History (from patient/relatives/friends):
Box 15.2 Respiratory ‘red flags’
Arterial blood gases
Arterial blood gas (ABG) sampling is performed to obtain accurate measures of arterial oxygen (PaO2), arterial carbon dioxide (PaCO2), and blood acidity/alkalinity (pH); these variables combined with body temperature allow for calculation of bicarbonate (HCO3) and arterial oxygen saturation (SaO2). Both PaO2 and PaCO2 can be affected by respiratory muscle weakness. Mild weakness leads to slight hypoxaemia (low oxygen, i.e. PaO2 <8 kPa/<60 mmHg) and hypocapnia (low carbon dioxide, i.e. PaCO2<4.7 kPa/<35 mmHg); severe weakness causes hypercapnia (high carbon dioxide, i.e. PaCO2 >6kPa/>45 mmHg), but only when muscle strength is <40% predicted (ATS 2002). However, ABG derangement is a late feature in neuromuscular disease, so normal results are compatible with significant weakness of the respiratory muscles (Hutchinson & Whyte, 2008). Patients with established respiratory failure from neuromuscular weakness will show hypoxaemia and a compensated respiratory acidosis (raised PaCO2 and HCO3 with a normal or mildly reduced pH).
Vital capacity
Vital capacity (VC) is the volume change at the mouth between full inspiration and complete expiration (see Figure 15.1). VC can be measured using conventional spirometers or recorded from equipment used to measure static lung volumes and their subdivisions. Guidelines for measurement have been published by the American Thoracic Society/European Respiratory Society task force (Wanger et al., 2005).
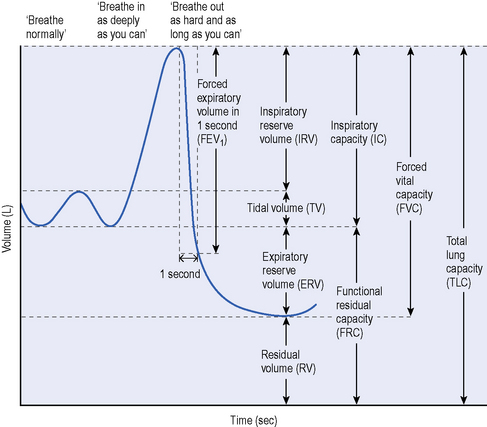
Figure 15.1 Lung volumes and capacities in neurological disease.
(From Aboussouan LF. Respiratory disorders in neurologic diseases. Cleve Clin J Med 2005; 72:511–520. Reprinted with permission. Copyright © 2005 Cleveland Clinic. All rights reserved.)
In acute neuromuscular disorders the VC and oxygen saturation should be rechecked at frequent intervals. A VC <1 L in an adult (<15 mL/kg), a fall in VC by more than 50% on serial testing, or onset of bulbar palsy are all indications to involve the intensive care unit (Hutchinson & White, 2008). In chronic neuromuscular disorders, monitoring can be less frequent, but a serial fall in VC (particularly a fall below 1.2–1.5 L or <40–50% of predicted) indicates a need for further respiratory assessment (Hutchinson & Whyte, 2008).
Peak cough flow
A normal cough requires the ability to generate sufficient inspiratory and expiratory power and a functional glottis.Ability to cough effectively is therefore compromised by either inspiratory or expiratory muscle weakness. However, expiratory muscle weakness has greater impact as mild to moderate expiratory muscle weakness can result in a weak cough, even if inspiration is normal (Boitano, 2006). In some neurological disorders, such as bulbar type motor neurone disease (MND; see Ch. 8), a functional glottis may be absent. Formal cough assessment requires the insertion of gastric balloons and is conducted in specialized laboratories, but cough strength can be assessed at the bedside using peak cough flow (PCF). PCF is a measure of maximal airflow generated during a cough manoeuvre. It provides a global indicator of cough strength that correlates well with the ability to clear secretions.
The recent joint guidelines from the British Thoracic Society (BTS) and Association of Chartered Physiotherapists in Respiratory Care for respiratory physiotherapy (Bott et al., 2009), include a guide for recording PCF in patients with neuromuscular weakness. It can be measured through either a mouthpiece or face mask attached to a peak flow meter and expressed in litres/minute or litres/second. The patient should be instructed to breathe in as deeply as possible and cough hard into the device. PCF is expected to be higher than peak expiratory flow, but in patients with bulbar dysfunction, this difference is not seen – potentially offering a way to monitor the onset of bulbar involvement (Boitano, 2006).
PCF is dependent on effort and lung volume, with cooperation being essential. The largest value from at least three acceptable attempts is usually recorded. Normal PCF is around 360 to 840 L/minute (Hutchinson & Whyte, 2008). Airway clearance becomes impaired and the risk of serious infection increases when PCF<160 L/minute, so in chronic progressive conditions, airway clearance techniques should be taught before these levels are approached (Tzeng & Bach, 2000). It has been suggested that any neuromuscular patients with a PCF<270 L/minute should be considered at risk of respiratory complications.
Inspiratory/expiratory pressures
Guidelines for testing the respiratory muscles have been published by the American Thoracic Society (2002). Inspiratory and expiratory pressures are recorded to assess respiratory muscle strength, but should be viewed as indices of global respiratory muscle output rather than as direct measures of their contractile properties (ATS, 2002). Mouth and nasal pressures can be recorded at the bedside using hand-held pressure meters. These volitional bedside tests are simple, portable and inexpensive; but their accuracy and reliability has been questioned because of their dependence on maximal effort and their significant learning effect. Non-volitional tests such as phrenic nerve stimulation are more reliable, but are expensive and confined to specialist centres.
Patients are normally seated and noseclips are not required. Careful instruction and encouraged motivation are essential. Measurements require maximal inspiratory or expiratory efforts against a quasi occlusion. The pressure must be maintained for at least 1.5 seconds, so that the maximum pressure sustained for 1 second can be recorded. The maximum value of three manoeuvres that vary by less than 20% is usually recorded, but some authors have recommended that more measures are needed to reach a true maximum, and even low variability between measures may not guarantee that maximal efforts have been made (Aldrich & Spiro, 1995). Although simple in principle, the manoeuvres are difficult for many patients and require a good seal around the mouthpiece. Low values may therefore be due to true muscle weakness, or a submaximal effort, or air leaks, e.g. in the case of facial muscle weakness. The sniff is an alternative manoeuvre that is more natural and easier for most patients (see section on Sniff test).
The normal ranges for PImax and PEmax are wide, so that values in the lower quarter of the normal range are compatible both with normal strength and with mild or moderate weakness. In adults a PImax of more than 80 cmH2O in males, or 70 cmH2O in females, excludes clinically significant respiratory muscle weakness (Hutchinson & Whyte, 2008).
Sniff test
A sniff is a short, sharp voluntary inspiratory manoeuvre involving contraction of the diaphragm and other inspiratory muscles. Sniff nasal inspiratory pressure (SNIP) has been proposed as a volitional, non-invasive measure of inspiratory muscle strength. Peak nasal pressure is measured in one occluded nostril during a maximal sniff, performed from relaxed end-expiration through the other open nostril. Portable commercial systems are available for measuring SNIP at the bedside. Patients should be encouraged to make maximal efforts, with a rest between sniffs. Most patients achieve a plateau within 5–10 attempts. A sniff test is not suitable in patients with nasal congestion, which leads to falsely low values (Fitting, 2006). The sniff is easily performed by most patients, requires little practice and is relatively reproducible. It is therefore a useful voluntary test for evaluating diaphragm strength in the clinical setting, giving equal or greater pressures than PImax. However, SNIP and PImax are not interchangeable and should be considered as complementing one another for the assessment of inspiratory muscle strength.
There are reference values for SNIP in adults (Uldry & Fitting, 1995) and children (Rafferty et al., 2000). Surprisingly, SNIP is similar in children and adults, despite a large difference in respiratory muscle mass. Values of maximal SNIP greater than 70 cmH2O (males) or 60 cmH2O (females) are unlikely to be associated with significant inspiratory muscle weakness (Hutchinson & Whyte, 2008). The SNIP test appears particularly suited to neuromuscular weakness because it obviates the use of a mouthpiece and because it is easily mastered by most patients. However, assessment of severe muscle weakness should not rely on SNIP alone, but should include other tests such as PImax, vital capacity, nocturnal oximetry or ABGs (Fitting, 2006).
Respiratory problems associated with neurological conditions
At a simplistic level, the mechanics of the respiratory system function as a pump to drive air into the body, analogous to the heart pumping blood around the body. The four essential elements of the pump are intact central neurological drive, neural pathways, thoracic cage and respiratory muscles. The respiratory system is only effective when there is a balance between the load on the system and the capacity of the respiratory pump to overcome this load (Polkey et al., 1995). Respiratory load is determined by the lung, thoracic and airway mechanics; respiratory capacity is the neuromusculoskeletal competence. Problems arise either from an increase in load (e.g. excessive secretions or bronchoconstriction) or from a decrease in capacity (e.g. neuromuscular pathology) or from a combination of both. Common relevant neurological problems are reduced airway protection and respiratory muscle weakness:
Table 15.2 Primary respiratory muscles and their level of innervations
Respiratory Muscles | Spinal Level |
---|---|
Sternomastoids | C1–C3 |
Diaphragm | C3–C5 |
Scalenes | C3–C8 |
Parasternal intercostals | T1–T5 |
Interosseus intercostals | T1–T11 |
External/internal abdominal obliques | T6–T12 |
Transversus abdominis | T7–L1 |
Some of these problems will be discussed further in the next section on physiotherapy management.
Physiotherapy management of specific problems
Problem 1: Breathlessness/dyspnoea
Once any treatable cause for breathlessness has been identified, appropriate action by the multidisciplinary team needs to be taken to relieve that cause where practicable (e.g. antibiotics for a respiratory infection, chest drain for pneumothorax). Symptom relief can be achieved through physiotherapy management of the breathless patient as described in Hough (2001). Techniques in current physiotherapy practice include: positioning, relaxation and various forms of breathing retraining (e.g. pursed lip breathing, Papworth breathing, Buteyko breathing) as well as complementary therapies such as acupuncture; however, there is a lack of evidence for effectiveness of any of these techniques in neurological patients. An overview of dyspnoea in neurological conditions has recently been published (Murtagh et al., 2006).
Oxygen therapy for dyspnoea
Even in patients with primary respiratory conditions, the use of oxygen to relieve breathlessness is controversial. In patients with neuromuscular weakness, although low flow oxygen is commonly prescribed and may relieve some symptoms and hypoxia, it usually results in a further increase in arterial CO2 and may therefore aggravate other symptoms. It should therefore be avoided or used with extreme caution in this group (Bott et al., 2009).
Problem 2: Sputum retention and atelectasis
In patients with neurological disorders, an ineffective cough is the main reason for sputum retention. Recent evidence-based guidelines on airway clearance techniques gave 10 recommendations for various patient populations (McCool & Rosen, 2006). However, only three of these recommendations related specifically to patients with neuromuscular weakness (i.e. manual cough assist (MCA), mechanical cough assist and expiratory muscle strength training) and none were supported by any robust evidence (Haas et al., 2007).
Box 15.3 provides the indicators of atelectasis or respiratory infection. Airway clearance therapy can be divided into techniques: to augment inspiration, to augment expiration/cough, and to mobilize/remove secretions (see Box 15.4 for a list of techniques in common clinical use).
Rising | Falling |
---|---|
Temperature Pulse rate Anxiety Secretion quantity/viscosity Breathlessness |
Vital capacity Peak cough flow |
Box 15.4 Techniques for managing sputum retention and/or atelectasis
Abdominal binder Active cycle of breathing techniques Assisted cough Autogenic drainage BiPAP/CPAP/IPPB* Bronchoscopy ELTGOL** Glossopharyngeal breathing Incentive spirometry In-exsufflator |
Intrapulmonary percussive ventilation Manual hyperinflation and suction (intubated patients) Manual physiotherapy techniques Nebulizers/humidifiers Physical exercise Positioning/postural drainage Positive pressure devices (e.g. PEP mask) Respiratory muscle training Suction |
* Bilevel positive airways pressure, continuous positive airways pressure, intermittent positive pressure breathing
** Slow expiration with the glottis open in a lateral posture known as ELTGOL (from the French: Expiration Lent Totale avec la Glotte Ouverte in infraLateral)
Augmenting inspiration
Inspiration can be augmented through thoracic expansion exercises in those who can perform them voluntarily, or by ‘air stacking’ which can be achieved under volition by glossopharyngeal breathing (see Bott et al., 2009 for a description), or by the use of a manual resuscitator bag or ventilator. Its effectiveness depends on ability to achieve glottis closure (Gauld, 2009). The aim is to increase volume so that expiratory flow can be increased and a cough is more effective. No barotrauma or complications have ever been reported from air stacking.
Augmenting expiration/cough
Manual cough assist
This involves using an abdominal thrust or a thoracic compression to augment the patient’s own cough – usually after augmented inspiration. The abdominal thrust is like a well-known manoeuvre to prevent choking, the Heimlich manoeuvre (Heimlich, 1975), which involves an upward thrust below the ribcage, so this technique should not be performed if the patient has a full stomach. Many patients prefer a thoracic squeeze, but its effectiveness is limited if there is decreased chest wall compliance, as is common in Duchenne muscular dystrophy (DMD; see Ch. 10) and scoliosis. When performing an abdominal thrust it is important to stabilize the chest wall and be careful not to injure abdominal organs or cause gastric reflux (Finder et al., 2004). Various hand placements can be used to provide costal lateral compression. Evidence for benefits of MCA is not high level, but it has consistently been found to increase peak cough flow. Its use is limited by severe scoliosis, orthopaedic or abdominal abnormalities, abdominal surgery, or the presence of abdominal/pelvic catheters. MCA should not be used in patients with Greenfield filters (filter in inferior vena cava to prevent pulmonary emboli) (Boitano, 2006). Recent research has reported that best cough improvement results from a combination of breathstacking plus MCA and that most benefit is derived by patients with VC >340 mL and PEmax < 34 cmH2O, but that MCA seems to interfere with spontaneous cough in patients with stronger cough and PEmax >34 cmH2O (Toussaint et al., 2009).
Mechanical cough assist (insufflation-exsufflation)
Devices such as the CoughAssist (Figure 15.2) provide mechanical insufflation-exsufflation (MIE) via a face mask and promote maximum lung inflation during inspiration with the use of positive pressure. A normal cough is simulated when inflation is followed by an abrupt switch to negative pressure in the upper airway. The device can also be used in patients with tracheostomy or endotracheal tubes.
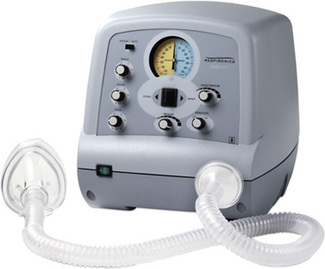
Figure 15.2 The CoughAssist® mechanical insufflator-exsufflator.
Photograph reproduced with kind permission from Philips Respironics.
MIE is indicated when inspiratory, expiratory and bulbar muscles are dysfunctional, but the latter are not completely paralysed and glottic closure is still possible (as is the case for most patients with neuromuscular diseases). Potential contraindications include a history of bullous emphysema (abnormal increase in size of air spaces arising from destruction of airway walls), susceptibility to pneumothorax (air in the pleural space causing collapse of lung tissue), or recent pulmonary barotrauma (damage to the lung from rapid or excessive pressure changes). If bulbar function is intact, MIE is not needed because cough flow can be greatly increased by abdominal thrusts following air stacking. MIE has been in use for secretion clearance since its first description in 1954. Despite increasing acceptance for airway clearance in patients with neuromuscular disease, there remains a lack of agreement on settings, with pressure settings ranging from 15 cmH2O to 45 cmH2O (insufflation and exsufflation pressures). Fauroux et al. (2008) demonstrated greater efficacy at higher settings (up to peak pressures of 45 cmH2O). During periods of acute illness the settings may need to change as lung mechanics change (Finder et al., 2004). Bach (2003) believes that mechanical insufflation/exsufflation is critical for avoiding hospitalizations, pneumonias, episodes of respiratory failure, and tracheostomy for patients with DMD, spinal muscular atrophy, and non-bulbar ALS.
Expiratory muscle training (see Respiratory muscle training section)
Mobilizing/removing secretions
In patients with neurological disorders manual physiotherapy techniques may be impossible due to chest wall or spinal deformities, or osteoporosis (Haas et al., 2007). Research in this group has focused on using mechanical devices such as the intrapulmonary percussive ventilator (IPV) or high frequency chest wall oscillator/compressor (HFCWO/C). HFCWO/C is administered via an external device (a commercially available vest) that delivers rapid small compressions to the thorax and generates high flows in small airways, to mobilize secretions from smaller airways to larger airways. Results for the use of HFCWO/C in neurological conditions have been inconsistent, so that although the technique is well tolerated and may be beneficial in some patients, it is not possible to recommend its use at present. IPV vibrates the airways internally, via the mouth, while the patient breathes spontaneously. Insufficient research has been conducted to judge its value in patients with neurological disorders. According to Haas (2007) when faced with a neurological patient with problems clearing secretions, and trying to choose which therapy to apply, the physiotherapist should ask four questions:
Problem 3: Hypoventilation
In the absence of significant respiratory muscle weakness, it may be possible to correct hypoventilation through strategies such as positioning to improve diaphragm mechanics, thoracic expansion exercises, respiratory muscle training or general physical exercise. In patients with significant respiratory muscle weakness, some form of non-invasive ventilation is often recommended – see section on respiratory failure below. Box 15.5 provides the signs and symptoms of respiratory muscle weakness or fatigue. Paradoxical (inward) inspiratory movement of the abdominal wall signifies respiratory strength reduced to at least about 30% of normal (Polkey et al., 1999).
Box 15.5 Common signs and symptoms of respiratory muscle weakness/fatigue
Respiratory muscle training
The respiratory muscles are physiologically, structurally and biochemically similar to other skeletal muscles, and it is therefore generally accepted that they will respond to appropriate strength and endurance training programmes in a similar manner (see Ch. 18). The controversy surrounds the issue of whether an increase in respiratory muscle strength or endurance confers any significant health benefit. There are a number of commercial devices available, but as with skeletal muscle training, there is a lack of consensus as to the optimal training programme (i.e. load, repetitions, frequency).
Although RMT has been studied extensively in some respiratory conditions, there is currently little evidence for use in patients with neurological conditions beyond spinal cord injury (SCI; see Ch. 4). According to a recent systematic review by Sheel et al. (2008), there are insufficient data to recommend the use of either exercise training or inspiratory muscle training for improved respiratory function in people with SCI. From another systematic review by Van Houtte et al. (2006) it was concluded that respiratory muscle training in SCI tended to improve expiratory muscle strength, vital capacity and residual volume. Insufficient data were available to make conclusions concerning the effects on inspiratory muscle strength, respiratory muscle endurance, quality of life, exercise performance or respiratory complications.
There is some limited evidence of benefit from the use of RMT in multiple sclerosis (MS; see Ch. 5) and DMD and currently a Cochrane review by Eagle and Chatwin (2006) is underway (online protocol updated 14/01/2009), which aims to assess the benefit and risk of RMT in children and adults with neuromuscular disease.
Problem 4: Respiratory failure
Acute respiratory failure
Guillain-Barré and myasthenia gravis account for the majority of cases of acute respiratory failure associated with neuromuscular disease in the developed world (Mehta, 2006). To avoid emergency intubation and ventilation it is essential to monitor patients regularly for signs and symptoms of impending failure (see Box 15.6).
Management of respiratory failure
Non-invasive ventilation
In some institutions non-invasive ventilation (NIV) is managed by physiotherapists. NIV is effective in providing intermittent, often nocturnal, support in various neuromuscular diseases and should be considered the standard of care for patients with MND who develop respiratory muscle weakness with PImax of <60% predicted or hypercapnia (Lechtzin, 2006). It is inappropriate for acute respiratory failure unless upper airway function is well preserved (Mehta, 2006) and therefore there is limited evidence to support its use in Guillain-Barré or myasthenia gravis.
NIV can be delivered via volume limited or pressure limited ventilators. Volume limited units are used mainly for long-term invasive ventilation. Pressure limited ventilators often allow the setting of both inspiratory and expiratory pressures (i.e. bilevel). Pressures are set according to patient tolerance, but Boitano (2006) suggests that ‘wide-span’ bilevel airway pressure (BiPAP) support, in which the difference between inspiratory and expiratory pressures is ≥ 10 cmH2O, most effectively augments ventilation. Typical pressures would, therefore, be approximately 12–14 cmH2O for inspiration and 2–4 cmH2O for expiration. A minimal expiratory pressure is better tolerated by patients with neuromuscular disease provided upper airway patency remains good during sleep. A spontaneous timed rate of 12–16 breaths per minute is necessary to provide back-up support during REM sleep when diaphragm weakness may preclude ability to trigger a breath. Interfaces vary, but nasal masks are the most common. Some patients with mouth weakness struggle to prevent air leakage around the mouth, in which case a chin strap or full face mask may be needed. If a mask fails to fit well or is too uncomfortable, a nose plug interface may be used, although this may not work as effectively. Hess (2006) has written a review of NIV equipment and its application in neuromuscular disease.
A Cochrane review into the use of nocturnal ventilation for hypoventilation in neuromuscular disorders has concluded that current evidence about any therapeutic benefit is weak, but consistent, suggesting alleviation of the symptoms of chronic hypoventilation in the short term. In three small studies survival was prolonged mainly in participants with MND. With the exception of MND, they recommended that further larger randomized trials are needed to confirm long-term beneficial effects of nocturnal mechanical ventilation on quality of life, morbidity and mortality, to assess its cost–benefit ratio in neuromuscular and chest wall diseases and to compare the different types and modes of ventilation (Annane et al., 2007).
Respiratory physiotherapy management post brain injury
Respiratory dysfunction is the most frequent medical complication in patients with brain injury (see Ch. 3).About 20–50% of neurosurgical patients develop pulmonary complications (such as aspiration of gastric contents or pneumonia), which significantly worsen neurological outcome and increase mortality. In traumatic brain injury the Brain Trauma Foundation (2000) recommends prevention of hypoxia by maintaining PaO2 >8 kPa (60 mmHg) and arterial oxygen saturation of greater than 90%. However, use of high levels of oxygen is not recommended and a SaO2 of 91–94% is considered optimal in neurosurgical patients. In patients with brain injury PaO2 is believed to be less important than brain tissue partial pressure (PbtO2). Normal values for PbtO2 are 2.13 kPa (16 mmHg) +/- 5.32 kPa (40 mmHg). A PbtO2 <0.67 kPa (5 mmHg) is associated with severe brain injury and a low chance of brain survival.
Hypocapnia (low CO2) is a potent cerebral vasoconstrictor, effectively reducing intracranial pressure (ICP), so short periods of hyperventilation to induce hypocapnia to PaCO2 of 4.66 kPa (35 mmHg), and even of 3.33 kPa (25 mmHg) may be used acutely in the treatment of increased ICP (Rozet & Domino, 2007). However, hypocapnia may exacerbate brain ischaemia in patients with brain injury, although the degree of ischaemia remains controversial. It is suggested that lowering the PaCO2 produces vasoconstriction of the blood vessels, thus reducing blood flow and, ultimately, brain oxygen delivery.
The goals of respiratory management in brain injury patients are therefore to attain normocapnia and prevent hypoxia. The same physiotherapy techniques described earlier may be used to achieve these goals in a spontaneously breathing patient, but invasive mechanical ventilation will frequently be needed (see Box 15.7).
Box 15.7 Indicators of need for intubation/ventilation in the neurosurgical patient
(Adapted from Rozet & Domino, 2007.)
Management of brain injury patients requiring invasive ventilation
Care of the ventilated patient with brain injury is a complex area, and respiratory physiotherapy will be aimed primarily at minimizing secretion retention, maximizing oxygenation, and re-expanding atelectatic lung segments. Techniques include those already described with the addition of more specialized techniques such as manual hyperinflation, suction and rotational therapy (see Stiller, 2000 for a full description). Some patients will require a tracheostomy, the detailed management of which is described in Jones and Moffatt (2002). A tracheostomy is a surgical procedure to create a direct airway through an opening in the trachea which facilitates secretion removal and protects the airway. It may be carried out for patients requiring prolonged invasive ventilation or for patients breathing spontaneously. It is therefore possible for a tracheostomy to remain in situ after a patient has been weaned from invasive ventilation. Physiotherapists are frequently involved with decisions relating to timing of weaning from mechanical ventilation and removal of tracheostomy tubes.
According to Olson et al. (2007) aggressive respiratory care in brain injury patients who require ventilation is essential to promote recovery without respiratory complications. However, although there is comparatively strong evidence for physiotherapy as the treatment of choice in acute lobar atelectasis, the evidence for the role of the physiotherapist in preventing respiratory complications (rather than treating them), is less strong. The need to avoid excessive stimulation of patients at risk of intracranial hypertension is often cited as a reason for avoiding some techniques in these patients, but there is a lack of conclusive evidence supporting the hypothesis that the techniques negatively impact ICP (Olson et al., 2007). Sogame et al. (2008) recommend daily respiratory physiotherapy and the use of a protocol to optimize early ventilator withdrawal for all ventilated neurosurgical patients. Recent guidelines for physiotherapy in the critically ill have been published (Gosselink et al., 2008), however their recommendations are not specific to brain injury patients, for whom minimal handling may be best practice. When managing a brain-injured patient via mechanical ventilation, the physiotherapist must make a clinical judgement based on the balance between potential positive and negative effects of interventions.
Aboussouan L.S. Respiratory disorders in neurologic diseases. Cleveland Clinic Journal of Medicine. 2005;72(6):511-520.
Aldrich T.K., Spiro P. Maximal inspiratory pressure: does reproducibility indicate full effort? Thorax. 1995;50:40-43.
Annane D., Orlikowski D., Chevret S., et al. Nocturnal mechanical ventilation for chronic hypoventilation in patients with neuromuscular and chest wall disorders. Cochrane Database Syst. Rev.. (4):2007. CD001941
ATS. ATS/ERS Statement on respiratory muscle testing. Am. J. Respir. Crit. Care Med.. 2002;166(4):518-624.
Bach J.R. Mechanical insufflation/exsufflation: has it come of age? A commentary. Eur. Respir. J.. 2003;21(3):385-386.
Boitano L.J. Management of airway clearance in neuromuscular disease. Respir. Care. 2006;51(8):913-922.
Bott J., Blumenthal S., Buxton M., et al. Guidelines for the physiotherapy management of the adult, medical, spontaneously breathing patient. Thorax. 2009;64(Suppl. 1):i1-i51.
The Brain Trauma Foundation. The American Association of Neurological Surgeons. The Joint Section on Neurotrauma and Critical Care. Resuscitation of blood pressure and oxygenation. J. Neurotrauma. 2000;17(6–7):471-478.
Eagle M., Chatwin M. Respiratory muscle training in children and adults with neuromuscular disease (Protocol). Cochrane Database Syst. Rev.. (3):2006. CD006155. DOI:10.1002/14651858.CD006155
Fauroux B., Guillemot N., Aubertin G., et al. Physiologic benefits of mechanical insufflation-exsufflation in children with neuromuscular diseases. Chest. 2008;133(1):161-168.
Finder J.D., Birnkrant D., Carl J., et al. Respiratory care of the patient with Duchenne muscular dystrophy: ATS consensus statement. Am. J. Respir. Crit. Care Med.. 2004;170(4):456-465.
Fitting J.W. Sniff nasal inspiratory pressure: simple or too simple? Eur. Respir. J.. 2006;27(5):881-883.
Gauld L.M. Airway clearance in neuromuscular weakness. Dev. Med. Child. Neurol.. 2009.
Gosselink R., Bott J., Johnson M., et al. Physiotherapy for adult patients with critical illness: recommendations of the European Respiratory Society and European Society of Intensive Care Medicine Task Force on Physiotherapy for Critically Ill Patients. Intensive Care Med.. 2008;34(7):1188-1199.
Haas C.F., Loik P.S., Gay S.E. Airway clearance applications in the elderly and in patients with neurologic or neuromuscular compromise. Respir. Care. 2007;52(10):1362-1381.
Heimlich H.J. A life-saving maneuver to prevent food-choking. JAMA. 1975;234(4):398-401.
Hess D.R. Noninvasive ventilation in neuromuscular disease: equipment and application. Respir. Care. 2006;51(8):896-911.
Hough A. Physiotherapy in Respiratory Care: an evidence-based approach to respiratory and cardiac management: A problem-solving approach. Cheltenham, UK: Nelson Thornes, 2001.
Hutchinson D., Whyte K. Neuromuscular disease and respiratory failure Practical Neurology. 2008;8:229-237.
Jones M., Moffatt F. Cardiopulmonary physiotherapy. Informa Healthcare. 2002.
Lechtzin N. Respiratory effects of amyotrophic lateral sclerosis: problems and solutions. Respir. Care. 2006;51(8):871-881.
McCool F.D., Rosen M.J. Nonpharmacologic airway clearance therapies: ACCP evidence-based clinical practice guidelines. Chest. 2006;129(Suppl. 1):250S-259S.
Mehta S. Neuromuscular disease causing acute respiratory failure. Respir. Care. 2006;51(9):1016-1021.
Murtagh F., Burman R., Edmonds P. Breathlessness in neurological conditions. In: Booth S., Dudgeon D., editors. Dyspnoea in advanced disease. A guide to clinical management. Oxford: Oxford University Press; 2006:99-112.
Olson D.M., Thoyre S.M., Turner D.A., et al. Changes in intracranial pressure associated with chest physiotherapy. Neurocrit. Care. 2007;6(2):100-103.
Polkey M.I., Lyall R.A., Moxham J., et al. Respiratory aspects of neurological disease. J. Neurol. Neurosurg. Psychiatry. 1999;66(1):5-15.
Polkey M.I., Green M., Moxham J. Measurement of respiratory muscle strength. Thorax. 1995;50:1131-1135.
Rafferty G.F., Leech S., Knight L., et al. Sniff nasal inspiratory pressure in children. Pediatr. Pulmonol.. 2000;29(6):468-475.
Rozet I., Domino K.B. Respiratory care. Best Pract. Res. Clin. Anaesthesiol.. 2007;21(4):465-482.
Sheel A.W., Reid W.D., Townson A.F., et al. Effects of exercise training and inspiratory muscle training in spinal cord injury: a systematic review. J. Spinal Cord Med.. 2008;31(5):500-508.
Sogame L.C., Vidotto M.C., Jardim J.R., et al. Incidence and risk factors for postoperative pulmonary complications in elective intracranial surgery. J. Neurosurg.. 2008;109(2):222-227.
Stiller K. Physiotherapy in intensive care: towards an evidence-based practice. Chest. 2000;118(6):1801-1813.
Toussaint M., Boitano L.J., Gathot V., et al. Limits of effective cough-augmentation techniques in patients with neuromuscular disease. Respir. Care. 2009;54(3):359-366.
Tzeng A.C., Bach J.R. Prevention of pulmonary morbidity for patients with neuromuscular disease. Chest. 2000;118(5):1390-1396.
Uldry C., Fitting J.W. Maximal values of sniff nasal inspiratory pressure in healthy subjects. Thorax. 1995;50(4):371-375.
Van Houtte S., Vanlandewijck Y., Gosselink R. Respiratory muscle training in persons with spinal cord injury: a systematic review. Respir. Med.. 2006;100(11):1886-1895.
Wanger J., Clausen J.L., Coates A., et al. Standardisation of the measurement of lung volumes. Eur. Respir. J.. 2005;26(3):511-522.