Respiratory Distress Syndrome
After reading this chapter, you will be able to:
• List the anatomic alterations of the lungs associated with respiratory distress syndrome.
• Describe the causes of respiratory distress syndrome.
• List the cardiopulmonary clinical manifestations associated with respiratory distress syndrome.
• Describe the general management of respiratory distress syndrome.
• Describe the clinical strategies and rationales of the SOAP presented in the case study.
• Define key terms and complete self-assessment questions at the end of the chapter and on Evolve.
Respiratory distress syndrome (RDS) is the most common cause of respiratory failure in the preterm infant. Over the past several decades, a number of names have been used to identify infants with RDS (Box 34-1). A common thread running through most of the names is the term “respiratory distress,” which characterizes an immature lung disorder in a preterm infant caused by inadequate pulmonary surfactant. RDS is a major cause of morbidity and mortality in the premature infant born after less than 37 weeks’ gestation. The introduction of exogenous surfactant therapy has greatly improved the clinical course of this disorder and reduced the morbidity and mortality rates.
Anatomic Alterations of the Lungs
In what appears to be an effort to offset alveolar collapse, the respiratory bronchioles, alveolar ducts, and some alveoli dilate. As the disease intensifies, the alveolar walls become lined with a dense, rippled hyaline membrane identical to the hyaline membrane that develops in acute respiratory distress syndrome (ARDS) of the adult patient (see Chapter 27). The membrane contains fibrin and cellular debris.
During the later stages of the disease, leukocytes are present, and the hyaline membrane is often fragmented and partially ingested by macrophages. Type II cells begin to proliferate, and secretions begin to accumulate in the tracheobronchial tree. The anatomic alterations in RDS produce a restrictive type of lung disorder (see Figure 34-1).
The major pathologic or structural changes associated with RDS are as follows:
• Interstitial and alveolar edema and hemorrhage
• Intraalveolar hyaline membrane
• Pulmonary surfactant deficiency or qualitative abnormality
• Hypoxia-induced vasospasm and vasoconstriction:
• Hyperperfusion (in cases lasting longer than 24 hours; leads to excessive lung fluid and pulmonary edema)
Etiology and Epidemiology
1. Because of the pulmonary surfactant abnormality, alveolar compliance decreases, resulting in alveolar collapse.
2. The pulmonary atelectasis causes the infant’s work of breathing to increase.
3. Alveolar ventilation decreases in response to the decreased lung compliance and infant fatigue, causing the alveolar oxygen tension (Pao2) to decrease.
4. The decreased Pao2 (alveolar hypoxia) stimulates a reflex pulmonary vasoconstriction.
5. Because of the pulmonary vasoconstriction, blood bypasses the infant’s lungs through fetal pathways—the patent ductus and the foramen ovale.
6. The lung hypoperfusion in turn causes lung ischemia and decreased lung metabolism.
7. Because of the decreased lung metabolism, the production of pulmonary surfactant is reduced even further, and a vicious cycle develops (Figure 34-2).
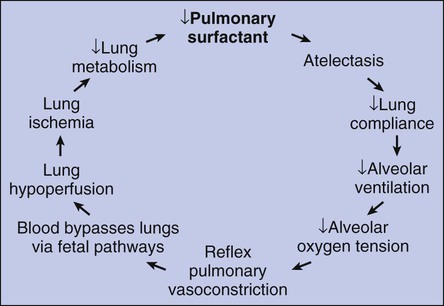
General Management of Respiratory Distress Syndrome
Finally, because of the decreased pulmonary surfactant associated with RDS, the administration of exogenous surfactant preparations such as beractant (Survanta), calfactant (Infasurf), and poractant alfa (Curosurf) is helpful. The term exogenous, used to describe these artificial surfactant agents, indicates that these preparations are from outside the patient’s body. Exogenous surfactant preparations originate from other humans, from animals, or from laboratory synthesis. These agents replace the missing pulmonary surfactant of the premature or immature lungs of the baby with RDS until the lungs are mature enough to provide adequate pulmonary surfactant. Figure 34-4 provides comparison chest radiographs of an infant without exogenous surfactant and the same infant 45 minutes after treatment.
Respiratory Care Treatment Protocols
Oxygen Therapy Protocol
Oxygen therapy is used to treat hypoxemia, decrease the work of breathing, and decrease myocardial work. Because of the hypoxemia that often develops in RDS, supplemental oxygen is usually required (see Oxygen Therapy Protocol, Protocol 9-1).
Respiratory Assessment and Plan
O Dyspneic and cyanotic. Retracting and using accessory muscles. Flaring of nostrils. RR 64 with “grunting.” HR 165. Bilateral crackles. CXR: Bilateral “ground-glass” haziness. ABGs on 30% O2: pH 7.25, Paco2 52, 21, Pao2 35, and Sao2 60%.
• Infant respiratory distress syndrome (history)
• Alveolar hyaline membrane, atelectasis (CXR)
P Mechanical Ventilation Protocol: Intubate, ventilate, positive end-expiratory pressure (PEEP) per neonatal intensive care unit (NICU) protocol. Oxygen Therapy Protocol: Continuous transcutaneous oximetry. Exogenous surfactant per protocol.
Discussion
Many of the clinical manifestations seen in this case are associated with Atelectasis (see Figure 9-8) and Increased Alveolar-Capillary Membrane Thickness (see Figure 9-10). For example, the use of accessory muscles of inspiration was likely a compensatory mechanism activated to offset the increased stiffness of the lungs (decreased lung compliance) caused by the atelectasis and alveolar hyaline membrane. The atelectasis and alveolar hyaline membrane were objectively verified by the chest x-ray film. In addition, the severity level of the anatomic alterations and clinical manifestations seen in this case was very high. This was objectively confirmed by the arterial blood gas analysis that identified the acute ventilatory failure with severe hypoxemia.
Thus the aggressive implementation of mechanical ventilation and use of artificial surfactant were certainly justified. Neonatal intensive care units usually are staffed by an in-house neonatologist, who can guide the respiratory therapist through the intricacies of therapy. Artificial surfactant has markedly improved the outlook for these infants. However, the respiratory care practitioner should be on the alert for sudden changes in lung compliance that often occurs shortly after the administration of artificial surfactant. If the infant is on a pressure-cycled ventilator, this is especially important to avoid volutrauma.* As in adults with ARDS, in which the pathology is very similar, constant attention must be given to the possibility of nosocomial infection, fluid overload, and cardiovascular instability. In addition, lung protection strategies such as PEEP, permissive hypercapnia, and use of small ventilator tidal volumes are commonly used in RDS cases.