Respiratory Disorders of the Newborn
I Persistent Pulmonary Hypertension in the Newborn (PPHN)
1. Pulmonary hypertension impairs the transition from fetal to neonatal circulation.
2. Right-to-left shunting occurs when the ductus arteriosus and foramen ovale remain open or reopen after closure.
B Pathophysiology (Figure 27-1)
1. Pulmonary hypertension and high pulmonary vascular resistance (PVR) impede blood flow to the lungs.
2. The pulmonary arterioles react to the resulting hypoxemia, hypercapnia, and acidemia with vasoconstriction, further impeding pulmonary blood flow.
3. High PVR results in right heart pressures greater than systemic blood pressure, causing right-to-left shunting through the ductus arteriosus and foramen ovale.
4. This combination of responses leads to a cyclic pattern of decreased cardiac output, decreased pulmonary blood flow, and vasoconstriction.
1. Near-term, term, and postterm neonates
2. Majority present within the first 72 hours after birth
4. Signs and symptoms of respiratory distress to varying degrees
1. Contrast or “bubble” echocardiography
a. Demonstrates the presence of right-to-left shunt through the foramen or ductus.
b. Used to evaluate cardiac structures and rule out cyanotic cardiac anomalies.
a. Place infant in 100% oxygen for 5 to 10 minutes and obtain arterial blood gas.
b. If Pao2 does not increase, right-to-left shunting is present.
c. Does not differentiate between PPHN and congenital heart disease.
3. Comparison of preductal and postductal arterial Pao2
a. Obtain simultaneous arterial blood gases from a preductal artery (right radial or brachial) and postductal artery (left radial or umbilical).
b. A difference of ≥15 mm Hg in preductal and postductal Pao2 indicates ductal shunting.
c. A difference of <15 mm Hg indicates no ductal shunting but does not rule out PPHN or congenital heart disease.
d. Pulse oximetry sensors can be placed at preductal and postductal sites to assess the presence of shunting and allow continuous monitoring for recurrent shunting.
4. Hypoxemia-hyperventilation test
a. Most definitive test to detect PPHN.
b. The patient is hyperventilated at a high rate using a manual resuscitator and 100% oxygen.
c. Hyperventilation results in a decrease in Paco2, causing pulmonary vasodilation.
d. PPHN is confirmed when Pao2 is <50 mm Hg before hyperventilation and increases to ≥100 mm Hg during hyperventilation.
e. For most congenital heart diseases, Pao2 will not increase significantly during this test.
a. Stop the cyclic pattern of deterioration.
b. Increase pulmonary blood flow by decreasing PVR or increasing systemic vascular resistance (SVR).
2. Minimize handling and stimulation of the infant to avoid transient hypoxemia.
a. A selective pulmonary vasodilator approved for management of PPHN in neonates of gestational age ≥34 weeks.
b. Initial dose is 20 parts per million (ppm).
c. If there is no response at 20 ppm, increasing to 40 to 80 ppm will not improve oxygenation.
4. Mechanical ventilation and oxygen
a. Conventional mechanical ventilation (CMV) and high frequency oscillatory ventilation (HFOV) have been used with success.
b. Hyperventilation and induced alkalosis have been widely used but are controversial.
(1) Effects of hyperventilation and alkalosis
(a) Acute pulmonary vasodilation
(c) Cerebral vasoconstriction, which may lead to neurodevelopmental problems.
(2) Because of the higher level of ventilator support necessary, hyperventilation carries an increased risk of lung injury.
c. A widely practiced approach is to set the ventilator to produce mild hypocapnia and respiratory alkalosis.
d. Success has been reported using lung protective ventilator strategies without inducing alkalinization.
(3) Manage metabolic acidosis with sodium bicarbonate.
(4) Use low positive end-expiratory pressure (PEEP) in the absence of parenchymal lung disease.
e. Muscle relaxants and sedatives to prevent patient-ventilator dysynchrony and resulting fluctuations of Pao2.
5. Administer surfactant if the infant has respiratory distress syndrome (RDS).
a. Increase SVR with vasopressors such as dopamine and dobutamine.
b. Decrease PVR and hypertension with vasodilators (e.g., prostaglandin I, tolazoline, or nitroprusside).
c. Correct hypotension using volume expanders and dopamine.
d. PPHN is reversed when the pulmonary artery pressure is less than the arterial pressure.
7. Extracorporeal membrane oxygenation (ECMO) is used for severe cases.
II Respiratory Distress Syndrome
1. RDS is a disease resulting from immature lung anatomy and physiology.
2. The primary abnormality is surfactant deficiency.
3. Without enough surfactant, alveoli collapse with each breath, and the lungs cannot maintain expansion.
4. Underdeveloped alveoli and pulmonary capillary beds further compromise gas exchange.
B Factors that increase the risk of developing RDS
4. Male infants have twice the risk of female infants.
5. Maternal hemorrhage during delivery
C Pathophysiology (Figure 27-2)
1. Surfactant deficiency causes atelectasis and reduced lung compliance.
2. Gas exchange is impaired, resulting in hypoxemia, hypercapnia, and acidemia.
3. Work of breathing increases, and the neonate begins to fatigue and hypoventilate.
4. Pulmonary vasoconstriction and increased PVR lead to hypoperfusion of the lung.
5. PPHN with shunting through the ductus arteriosus (right-to-left shunt).
6. Hypoxia causes damage to alveolar epithelium.
7. Pulmonary capillary permeability increases, and plasma leaks from the capillaries.
8. Fibrin present in plasma forms a hyaline membrane that lines the alveoli and bronchioles.
9. Alveolar ventilation decreases, and diffusion gradient increases.
10. High FIO2 and airway pressures during mechanical ventilation cause further tissue damage.
E Radiographic findings (Figure 27-3)
1. Classic appearance in the untreated newborn is reticulogranular infiltrates described as having a “ground glass” appearance.
2. Air bronchograms show collapsed alveoli surrounding air-filled bronchi.
3. Chest radiograph changes take place within 8 hours.
4. Severe RDS may progress to a “total whiteout,” in which the heart border and diaphragm are indistinct because of severe atelectasis.
1. Monitoring of vital signs and arterial blood gases.
2. Manage hypoxemia; maintain Pao2 of 60 to 80 mm Hg.
3. Continuous positive airway pressure (CPAP) may be used initially.
4. Mechanical ventilation is usually required to manage severe acidemia, hypercapnia, and hypoxemia.
5. High respiratory rate, FIO2, and airway pressures are often required.
6. HFOV may be used either initially or in cases in which CMV is unsuccessful.
7. Surfactant replacement therapy
a. Synthetic and animal-derived products are available (Table 27-1).
TABLE 27-1
Surfactant Replacement Therapy
Generic Name | Brand Name | Source | Characteristics | Route | Dosage |
Colfosceril | Exosurf | Synthetic surfactant | No surfactant associated proteins | Side port on endotracheal tube adaptor | Initial: Two 2.5-ml/kg half-doses; avoid endotracheal suctioning for 2 hr after treatment |
Beractant | Survanta | Exogenous surfactant from bovine lung extract | Contains surfactant associated proteins | Intratracheal | Initial: 4 ml/kg; if needed, 4 doses in the first 48 hr of life; ≥ 6 hr between each dose |
Poractant alfa | Curosurf | Derived from minced porcine lung extract | Contains surfactant associated proteins | Intratracheal | Initial: 2.5-ml/kg dose divided in 2 aliquots; up to 2 more doses of 1.25 ml/kg, 12 hr apart, if needed |
Calfactant | Infasurf | Derived from lavage of calf lungs | Contains surfactant associated proteins | Intratracheal | Initial: 3-ml/kg dose divided into 2 aliquots; 3 doses of 3 ml/kg, 12 hr apart, if needed |
b. Method of administration depends on the drug used.
(1) Can be given through a side port adaptor on the endotracheal tube (ETT).
(2) Or through a small catheter inserted just past the tip of the ETT.
c. Results in rapid and dramatic improvement in lung compliance and gas exchange.
d. High levels of ventilatory support usually can be significantly reduced.
e. Widespread use of surfactant has markedly decreased mortality from RDS.
f. To avoid potential pneumothorax, it is important to decrease airway pressures and tidal volumes promptly when compliance improves during or immediately after surfactant administration.
g. The most common adverse effects are transient oxygen desaturation and bradycardia.
9. Maintain normal body temperature, and minimize stimulation of infant.
10. Provide appropriate fluid, electrolytes, glucose, and calories.
11. Maintain blood pressure and hematocrit.
III Meconium Aspiration Syndrome (MAS)
1. Ten percent to 22% of newborns have meconium-stained amniotic fluid.
1. Meconium is a viscous fluid consisting of undigested amniotic fluid and epithelial cells.
2. Meconium is present in the colon late in gestation.
3. Meconium staining of the amniotic fluid can be a sign of fetal distress and hypoxemia.
4. Fetal responses to intrauterine hypoxemia
a. Anal sphincter relaxes, and peristalsis increases.
b. Meconium passes into amniotic fluid.
c. Fetus makes gasping respiratory efforts, drawing meconium into the pharynx.
5. During the newborn’s initial breaths, meconium present in the pharynx is aspirated below the vocal cords.
1. The effects of meconium aspiration on the lower airways:
a. Creates an airway obstruction
(1) Meconium acts as a ball valve in the airways, allowing inspiration but obstructing effective expiration.
(2) The results are air trapping, hypoventilation, and atelectasis.
b. Begins an inflammatory response in the lungs known as chemical pneumonitis
2. During the first few hours after aspiration, hypercapnia, hypoxemia, and metabolic acidosis develop.
3. As pulmonary vascular resistance increases, the infant often develops PPHN.
4. If mechanical ventilation is required, recovery may be complicated by barotrauma and pneumothorax.
1. Bilateral diffuse, coarse, patchy infiltrates
1. Meconium staining of the amniotic fluid.
2. Sign and symptoms of respiratory distress
3. Arterial blood gases show hypoxemia, hypercapnia, and respiratory and lactic acidosis.
4. Breath sounds are coarse with rhonchi and rales.
6. In severe cases the chest is hyperexpanded from air trapping.
1. If meconium-stained amniotic fluid is present, the mouth, nose, nasopharynx, and oropharynx are always suctioned when the newborn’s head presents during birth, before the first breath is taken.
2. If meconium is present in the oropharynx, the vocal cords are viewed under direct vision, and the airway is suctioned above the cords.
3. If meconium is suctioned above the vocal cords, the trachea is intubated with a meconium aspirator, and the airway below the cords is suctioned.
4. The airway must be cleared of meconium by suction before any positive pressure breaths are given.
5. Supplemental blow-by oxygen is usually necessary.
6. CPAP is often used to improve oxygenation.
7. Mechanical ventilation is initiated if the infant fails to respond to oxygen with CPAP and develops worsening hypoxemia, hypercapnia, and acidemia.
8. HFOV may be used as primary therapy or when ventilation with CMV is unsuccessful.
9. Term infants often require sedation and sometimes muscle relaxers to maintain synchrony with the ventilator.
10. Inhaled nitric oxide can be used if PPHN is present.
11. Secretion clearance techniques, such as frequent endotracheal suctioning and chest physical therapy, are often useful.
12. ECMO may be required to manage severe MAS (Table 27-2).
TABLE 27-2
ECMO/ECLS Use and Survival for Neonates
Diagnosis | Number of Cases* | Percentage Survival |
MAS | 6263 | 94 |
CDH | 4101 | 53 |
Sepsis | 2307 | 75 |
PPHN | 2649 | 79 |
RDS | 1357 | 84 |
Others | 1411 | 65 |
*Includes all cases reported to the registry.
From ELSO registry data. January 2003.
1. Stiff lungs with low compliance (e.g., RDS)
2. Hyperinflated lungs (e.g., MAS)
3. Hypoplastic lungs (e.g., congenital diaphragmatic hernia)
a. Asymptomatic air leaks occur in 2% to 10% of term infants.
b. Caused by high pressures (40 to 80 cm H2O) that the infant generates to take its first breath.
c. Usually minor and resolve without treatment in 24 to 48 hours.
6. Surfactant replacement therapy has significantly reduced the incidence of pneumothorax.
1. Air trapping in obstructed airways.
2. Regional overdistention and rupture of alveoli can occur when more compliant alveoli are adjacent to atelectatic alveoli.
3. Ruptured alveoli can result in pulmonary interstitial emphysema (PIE).
1. Chest radiography is the gold standard.
2. Transillumination is useful for critically ill infants if there is a delay in obtaining the chest radiograph.
a. Transillumination is the passage of light through body tissues for the purpose of examining a structure.
b. A fiberoptic transilluminator is placed against both sides of the infant’s chest superior and inferior to the nipple.
c. If the lung is inflated the tissue will absorb most of the light.
d. A greater appearance of light through the chest suggests an abnormal amount of air in the thoracic cavity.
e. In full-term infants, pneumothorax may not be visible under transillumination.
1. Prompt decompression by chest tube placement
a. The tube is inserted at the second to third intercostal space lateral to the midclavicular line.
b. The tube is connected to an underwater seal drainage unit with −20 cm H2O of suction.
2. Chest tube placement usually results in immediate improvement.
3. Needle aspiration is sometimes performed in an emergency.
A Incidence of pneumonia during birth or after delivery
1. Bacteria usually cause the infection.
a. Group B Streptococcus is the most common pathogen.
b. Gram-negative organisms (e.g., Escherichia coli, Klebsiella, Pseudomonas, Serratia marcescens)
c. Less frequently caused by Staphylococcus aureus or Staphylococcus epidermis
2. Candida can cause fungal pneumonia.
1. The neonate can acquire infection via three routes.
a. Transplacental, when pathogens are transferred across the placenta in utero.
b. Perinatal, by aspiration of contaminated amniotic fluid during labor and delivery.
c. Postnatal, from the mother, caregivers, or environment during the hospital stay.
2. Rupture of placental membranes ≥12 hours before birth increases the chance that infectious agents will spread to the amniotic fluid and the fetus.
3. Bacterial pneumonia causes inflamed, fluid-filled alveoli more often than viral pneumonia, and in severe cases necrosis of lung tissue develops.
4. Sepsis can rapidly develop from gram-negative pulmonary infections.
5. Bacterial pneumonia acquired in utero leads to stillbirth and premature delivery in many cases.
6. Pneumonia caused by viruses and mycoplasmae involve the bronchi and interstitium, resulting in loss of ciliary function and mucus stasis.
7. Fungal infections can produce a layer of hyphae that line the upper and lower respiratory tract, eventually leading to ulcerations in the airway.
1. Signs and symptoms are similar to those of respiratory distress, transient tachypnea of the newborn, and sepsis.
2. A chest radiograph is often necessary to differentiate between diagnoses.
1. Chest radiographs have various patterns of infiltrates that are often characteristic of a specific causative organism (Table 27-3).
TABLE 27-3
Chest X-ray Findings in Neonatal Pneumonia
Causative Agent | Appearance of Chest Radiograph |
Group B β -hemolytic Streptococcus (GBS) | Diffuse opacities (“white out”), patchy infiltrates, pleural effusions |
Streptococcus pneumoniae | Patchy lobar infiltrates, pleural effusion |
Klebsiella | Bilateral consolidation, lung abscess, pneumatocele |
Pseudomonas and Serratia | Parenchymal consolidation (patchy or basilar), pneumatocele |
Respiratory syncytial virus | Hyperexpansion, patchy consolidation |
Candida albicans | Diffuse granularity, coarse infiltrates, opacification |
From Merenstein GB, Gardner SL: Handbook of Neonatal Intensive Care, ed 5, St. Louis, Mosby, 2002.
2. Tracheal aspirates and blood cultures help identify causative organisms and diagnose sepsis.
3. Complete blood count (i.e., neutrophil count, platelet count) may be useful to diagnose sepsis and other infections, but results from these tests are not specific enough to differentiate between causative factors.
1. Supplemental oxygen to maintain Spo2 at ≥90%.
2. Broad-spectrum antibiotics initially (e.g., ampicillin, gentamicin).
3. If the organism is identified, antibiotics may be changed.
1. Mortality has been estimated at 20% for perinatally acquired pneumonia.
2. Mortality for postnatal pneumonia is approximately 50%.
3. The most recent reviews indicate a decrease in overall mortality to approximately 10% in perinatally acquired pneumonia attributed to perinatal use of antibiotics.
VI Transient Tachypnea in the Newborn (TTN)
1. The fetal lung is normally filled to the functional residual capacity level with fluid that is produced in the lungs (not amniotic fluid).
2. As the neonate passes through the birth canal during a normal delivery, “thoracic squeeze” removes one third of the fluid present in the lungs at birth.
3. The remaining fluid is removed slowly by the neonate’s lymphatic system.
4. The excessive fluid in the lungs and interstitium interferes with the ability to hold bronchioles and alveoli open.
5. Air trapping and small airway collapse result, causing changes in pulmonary mechanics and breathing pattern of the infant.
6. TTN is usually a benign and self-limiting condition.
7. However, TTN may be complicated by PPHN or evolve into RDS.
1. Term infants with tachypnea that develops 2 to 6 hours postpartum
2. Mild to moderate respiratory distress
4. Arterial blood gases show mild hypoxemia and mild acidemia.
1. Fluid in fissures and effusions
2. Streaky appearance of lung parenchyma
4. Radiographic findings may mimic cardiac problems, but in TTN these changes resolve within 72 hours.
A Apnea of prematurity or primary apnea
1. The younger the gestational age, the greater the incidence of apnea.
2. Not caused by a specific disease entity.
3. There are several factors that have been associated with primary apnea.
a. Response to hypoxemia and hypercapnia
(1) Neonatal response differs from the adult response.
(2) Adults respond with a sustained increase in ventilation.
(3) Infants have a brief increase in ventilation, followed by respiratory depression.
b. Immaturity of the neurons that control respiratory rate and rhythm may contribute to primary apnea. Premature infants have fewer dendrites and synaptic connections, and this may alter transmission of impulses involved in control of breathing.
c. Apnea in infants occurs more often during sleep, especially rapid eye movement (REM) sleep. The known neurologic effects during REM sleep include inhibition of spinal motor neurons, increased movement of the eyes, muscle twitching, and increased cerebral blood flow. There may be other effects that directly or indirectly influence drive to breathe.
d. Respiratory muscle fatigue: The premature infant has an increased workload from the combination of a compliant chest wall and less compliant lungs.
B Secondary apnea is caused by specific disorders that directly or indirectly lead to hypoxemia and depression of the drive to breathe.
2. Central nervous system disorders such as intraventricular hemorrhage or seizures
3. Infection, particularly sepsis
4. Left-to-right shunting through a patent ductus arteriosus (PDA)
1. Environmental: Sustained increases or sudden changes in temperature
2. Vagal response to endotracheal suctioning
3. Secretions or other foreign material in the oropharynx can cause apnea because of the laryngeal chemoreflex. This reflex protects against aspiration by preventing inspiration during feeding.
4. Improper neck positioning can cause obstruction of the airway.
1. Spo2 and heart rate should be monitored, and alarm systems should remain active.
a. Bradycardia and desaturation often follow an apneic period.
b. Impedance apnea monitors are not used because they do not differentiate between normal breathing and the gasping efforts seen with airway obstruction.
c. High-risk neonates should be monitored for at least 10 to 12 days.
2. Reduce environmental stress
3. During apneic spells use the least invasive intervention that stimulates the infant to breathe.
a. Provide gentle tactile stimulation.
b. If stimulation is not immediately effective, use bag-mask ventilation.
(1) Use FIO2 of approximately 10% above that used before the episode to manage hypoxemia.
(2) Increasing the ambient FIO2 will decrease the frequency but prolong the duration of apneic periods.
c. If necessary use nasal or nasopharyngeal CPAP.
d. Mechanical ventilation may be needed for extremely premature or unstable neonates.
4. Manage disorders that cause secondary apnea.
5. Methylxanthines are sometimes used to manage apnea of prematurity.
VIII Chronic Disorders Associated with Prematurity
A Bronchopulmonary dysplasia (BPD)/chronic lung disease (CLD)
1. BPD is a chronic disorder in premature infants characterized by
2. Originally BPD described a classic progression of radiographic and functional changes in the lungs of survivors of RDS.
3. The BPD seen today is not as severe; with current treatment premature infants do not necessarily progress through all of the classic stages.
4. CLD is a term used to describe premature infants who require supplemental oxygen at 28 to 30 days of life or at 36 weeks’ postmenstrual age.
5. Etiology: The causes of BPD/CLD are iatrogenic.
(1) Historically believed to occur because of long-term exposure to high FIO2.
(2) However, BPD/CLD has been documented after short- and long-term exposure to high and low FIO2.
b. Lung injury associated with positive pressure ventilation.
a. Developmental immaturity of the lungs makes them more susceptible to damage.
b. Injury to alveolar epithelium and fibrin-producing hyaline membranes occurs when the neonate develops RDS.
c. Repair of the lung injury requires treatment with oxygen and positive pressure ventilation, two of the factors that contribute to lung injury and development of BPD.
d. A cycle of constant and recurring lung injury and repair is established.
(1) Develops during the first 3 days of life.
(2) Chest radiography shows ground glass (reticulogranular) appearance and air bronchograms.
(1) Develops during the first 3 to 10 days of life.
(2) Hyaline membrane formation
(3) Stages I and II correlate with development and resolution of RDS.
(4) Chest radiography shows granular opacity of the lung obscuring the cardiac borders.
(1) Develops during the first 10 to 20 days of life.
(2) Marks the transition into chronic phase.
(3) Chest radiography shows opaque lungs with multiple small cysts and visible cardiac borders.
(1) Develops after 28 days of life.
(2) Chest radiography shows larger, irregular cysts and areas of increased density.
(3) Marked respiratory distress, cyanosis, and diffuse rales.
(4) Hyperinflation, flattened diaphragm, and atelectasis may be evident.
(5) Cardiomegaly develops from cor pulmonale.
(6) Thickened alveolar walls and increased diffusion gradient
a. Initially the infant presents with the signs and symptoms of RDS.
b. In later stages signs include
(1) A medical history of prematurity, moderate to severe RDS, mechanical ventilation, and difficulty weaning from the ventilator.
(2) Abnormal breathing pattern: Tachypnea, retractions, and nasal flaring
(3) Poor tolerance of feeding and handling
(5) Crackles and decreased air entry
(6) Pulmonary hypertension with right-sided heart failure
(7) Children aged <2 years with CLD are frequently readmitted to the hospital with viral respiratory infections caused by respiratory syncytial virus (RSV).
1. Maintain adequate oxygenation and ventilation
2. Weaning from mechanical ventilation
3. Provide adequate nutrition to facilitate healing of damaged lung tissue.
4. Drug therapy is used to improve lung mechanics.
(1) Bronchodilators (β2 agonists) are commonly used to manage bronchospasm in infants with BPD/CLD.
(2) Inhaled steroids are used to decrease airway inflammation.
(3) Histamine inhibitors (Cromolyn) are used to reduce airway reactivity and inflammatory response.
b. Effectiveness of aerosolized medications
(1) β2 agonists act on bronchial smooth muscle, but this tissue is poorly developed in premature infants, thus limiting the effectiveness of these drugs.
(2) Small ETTs baffle the aerosol, preventing 90% of the drug from reaching the lungs.
(3) Drug delivery in intubated infants is poor from metered dose inhalers (MDI) and small volume nebulizers (SVNs).
c. Methylxanthines are used to promote weaning from low-level ventilatory support.
d. Diuretics can improve lung mechanics and facilitate weaning.
e. Antenatal and postnatal steroids are widely used and have been linked to decreased mortality and faster weaning.
f. Studies comparing the efficacy of inhaled versus systemic steroids have yielded conflicting results.
5. Unfortunately very small premature infants almost always have complications from BPD as children (Box 27-1).
IX Retinopathy of Prematurity (ROP)
A ROP is a common complication of prematurity.
1. Develops in approximately 85% of premature infants of <28 weeks’ gestation.
2. Develops in approximately 65% of premature infants weighing <1250 g.
1. ROP has long been associated with lengthy exposure to high FIO2, but this process is not yet fully understood.
2. Many other risk factors appear to contribute to the development of ROP.
a. Maternal hypertension during pregnancy caused by smoking, diabetes, or other causes
b. Prematurity, low birth weight, and multiple gestations
c. Anemia and blood transfusions
d. Apnea and bradycardia in the infant, along with cycles of hyperoxia and hypoxia
e. Cytokine release caused by sepsis in the infant
f. Intraventricular hemorrhage and/or seizures
h. Ethnicity: White infants are more likely to develop ROP than African-American infants.
1. Most blood vessels supplying the retina are developed by 32 weeks’ gestation, but those in the peripheral regions may not develop until 40 weeks.
2. Retinal vessels constrict in response to hyperoxia.
3. Some vessels dilate and revascularize their surrounding areas.
4. Other vessels constrict permanently and become necrotic in a process called vasoobliteration.
5. The remaining blood vessels begin to proliferate as they attempt to provide blood flow to the obliterated areas of the retina.
6. If the vessels continue to proliferate, the damage can progress from hemorrhage to retinal scarring, retinal detachment, and blindness.
7. Early changes are often seen in the temporal periphery of the retina, the region where vascularization develops in the last few weeks of gestation.
8. ROP can no longer occur when the vascular development of the retina is complete.
D Classification of the severity of ROP (Figure 27-5)
a. The retina is divided into zones composed of two concentric circles and a crescent-shaped area lateral to the nose.
b. Zone 1 is the interior, and zone 3 is the outermost region.
1. The most effective way to prevent ROP is to prevent prematurity.
2. Pulse oximetry and arterial blood gases must be monitored whenever oxygen is administered.
3. The recommended high alarm setting for preterm infants’ SpO2 is ≤96%. This decreases the risk of injury for ROP and BPD/CLD.
4. Maintain Pao2 of 65 to 90 mm Hg.
5. Reduce the time of exposure to high FIO2 levels (≥0.50).
6. Use oxygen blenders to provide specific FIO2 level during procedures (i.e., suctioning, apneic spells, and endotracheal intubation).
7. Early and frequent ophthalmic examination in premature infants, especially those weighing <1500 g.
8. Laser surgery or cryotherapy to manage retinal detachment has yielded good results.
X Congenital Heart Disease (Box 27-2)
A Approximately 1% of newborns are born with a heart defect.
1. The single most important factor is family history of congenital heart defects.
2. Approximately 8% of congenital defects are associated with specific chromosomal abnormalities (e.g., trisomy 21 or trisomy 13).
3. Environmental factors include maternal viral infections (rubella) and maternal drug or toxic substance ingestion (e.g., seizure medications, alcohol).
C Newborns with severe cardiac defects generally have one or more of the following signs and symptoms.
D CHF is a syndrome characterized by decreased cardiac output and decreased tissue perfusion.
1. Results from the inability of the myocardium to fulfill the body’s metabolic needs.
a. Tachycardia as the heart attempts to increase cardiac output.
b. Enlargement of the heart from one or both of the following
(1) Dilation of the chambers from excess volume
(2) Hypertrophy from increased myocardial work to overcome pressure gradients
c. Tachypnea is the initial sign, followed by other signs of respiratory distress.
d. Poor perfusion and mottling of extremities
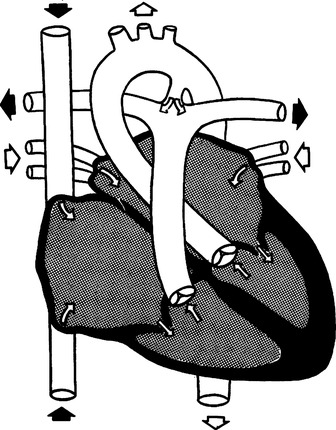
1. Cyanosis is not present because the shunt is from left to right.
2. The ductus arteriosus fails to close at birth.
3. A pressure gradient exists between the aorta and the pulmonary artery; therefore, left ventricular output flows through the patent ductus into the pulmonary artery.
4. Right ventricular work increases, resulting in CHF.
5. PDA is common in preterm infants with RDS.
(1) Indomethacin, a prostaglandin inhibitor that causes constriction of the ductus arteriosus
(2) Indomethacin is much less effective if administered after 7 days of life.
b. Surgical ligation or clipping of the ductus arteriosus carries little risk when performed by an experienced cardiac surgical team.
F Atrial septal defect (ASD) (Figure 27-7)
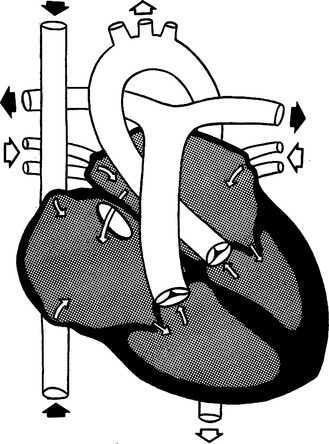
1. Cyanosis is not present because the shunt is from left to right.
2. When the foramen ovale fails to close or when the atrial septum does not develop normally, the result is an ASD.
a. Ostium primum occurs low in the atrial wall and usually involves the mitral and tricuspid valves.
b. Ostium secundum is an isolated defect occurring high in the atrial wall.
4. Because of the higher pressure in the left atrium, blood flows from the left atrium into the right atrium through the ASD.
5. Work of the right side of the heart often increases, and CHF develops.
G Ventricular septal defect (VSD) (Figure 27-8)
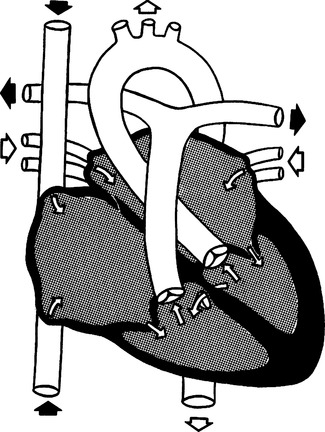
1. Cyanosis is rarely present because the shunt is from left to right.
2. Defects vary considerably in size and may occur in conjunction with other cardiac defects.
3. VSD (either alone or associated with other anomalies) account for 50% of congenital heart defects.
4. Two factors influence the effect of the VSD on circulation.
H Aortic stenosis (Figure 27-9)
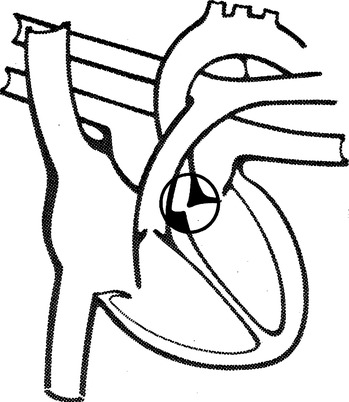
1. Cyanosis is not generally present.
2. Flow from the left ventricle into the aorta is partially obstructed.
3. The most common obstruction is a defect in the aortic valve where the leaflets of the valve are fused.
4. A pressure gradient of ≥50 mm Hg between the left ventricle and the ascending aorta indicates aortic stenosis.
5. Left ventricular hypertrophy and CHF develop as a result of the increased resistance.
I Pulmonary stenosis (Figure 27-10)
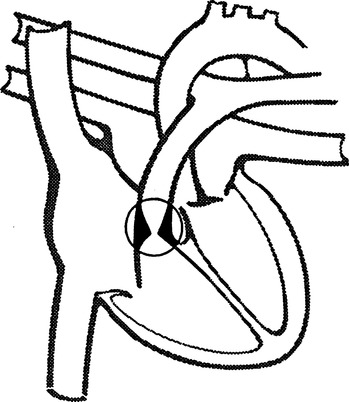
1. Cyanosis is not present if this is the only cardiac lesion present.
2. Blood flow into the pulmonary artery is obstructed, most often caused by a defect in the pulmonary valve.
J Coarctation of the aorta (Figure 27-11)
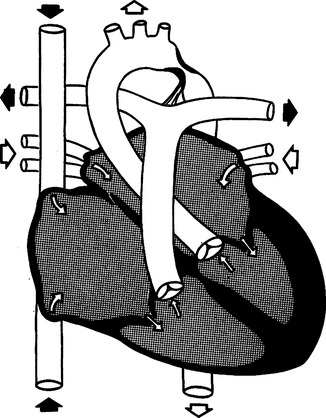
1. Cyanosis is not generally present.
2. Characterized by a constriction in the lumen of the aorta.
3. The most common location is near the ductus arteriosus at the junction of the transverse aortic arch and descending aorta.
4. Therefore most of the cardiac output is to the head and upper extremities.
5. If coarctation is present in this location, systolic blood pressure is ≥15 mm Hg higher in the upper extremities than in the lower extremities.
K Tetralogy of Fallot (Figure 27-12)
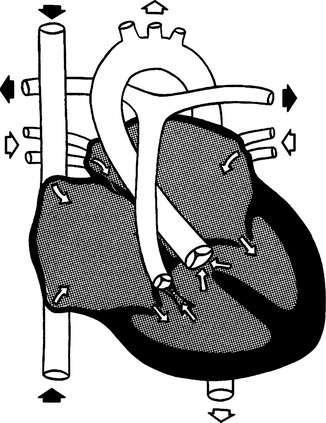
1. Cyanosis is present because of right-to-left shunt.
2. Tetralogy of Fallot is the most common cyanotic anomaly.
5. Infants have occasional hypercyanotic episodes with severe cyanosis, tachypnea, irritability, flaccidity, and sometimes loss of consciousness.
a. Hypercyanotic episodes are managed with propranolol or sometimes morphine.
b. Surgery in the small neonate involves building a shunt between the aorta and pulmonary artery to increase pulmonary blood flow.
c. Total repair involves closure of the VSD and correction of the outflow obstruction at the pulmonary artery.
L Truncus arteriosus (Figure 27-13)
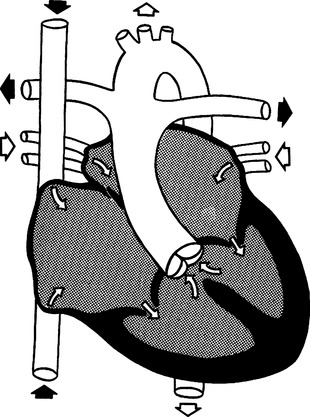
1. Cyanosis is present because of right-to-left shunt.
2. Characterized by a common artery (truncus) originating from both ventricles and overriding a VSD.
3. The truncus carries a mixture of oxygenated and unoxygenated blood and is the origin for the aorta, coronary arteries, and both branches of the pulmonary arteries.
4. Pulmonary blood flow varies, depending on the location of the branching of the pulmonary arteries off the truncus and the degree of pulmonary hypertension.
5. Pulmonary blood pressure is usually equal to systemic blood pressure.
6. Right and left ventricular hypertrophy and CHF commonly occur.
M Complete transposition of the great vessels (Figure 27-14)
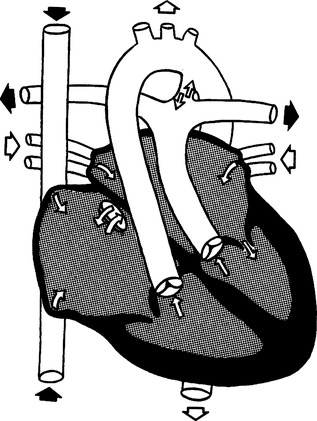
1. Cyanosis is present because of right-to-left shunting.
2. Characterized by reversed position of aorta and pulmonary arteries.
a. The aorta originates from the right ventricle and pumps unoxygenated blood to the body.
b. The pulmonary artery originates from the left ventricle and pumps oxygenated blood back to the lungs.
c. Thus two separate circulations occur, and mixing can only occur if there are other lesions present.
3. Venous and arterial blood may mix through an ASD, VSD, or PDA (Table 27-4).
TABLE 27-4
Cardiac Defect | Anatomic Characteristics | Direction of Shunt | Presence of CHF | Presence of Cyanosis | Treatment |
Patent ductus arteriosus (PDA) | Failure of ductus arteriosus to close at birth | Left-to-right | Present | Acyanotic | Medical: Indomethacin |
Surgical: Ligation of PDA | |||||
Atrial septal defect (ASD) | Failure of foramen ovale to close or malformation in atrial septal wall | Left-to-right | Present | Acyanotic | Surgical closure |
Ventricular septal defect (VSD) | Malformation in ventricular septal wall | Left-to-right | Present, if large VSD | Acyanotic | Large VSD requires surgical closure |
Aortic stenosis | Most common type an aortic valve with fused leaflets | No shunt, if isolated lesion | Present | Acyanotic | Surgical correction is aortic valvulotomy |
Pulmonary stenosis | Most common type is defect in the pulmonary valve with fused leaflets | No shunt, if isolated lesion | Present | Acyanotic | Pulmonary valvulotomy |
Coarctation of the aorta | Constriction in lumen of aorta near ductus arteriosus | No shunt | Sometimes | Acyanotic | Surgical resection of the stricture |
Tetralogy of Fallot | VSD with overriding aorta, outflow obstruction of RV, RV hypertrophy | Right-to-left | Rare | Cyanotic | Surgery to create shunt from aorta to PA, VSD closure, correction of outflow obstruction |
Truncus arteriosus | Common artery (truncus) overriding a VSD | Right-to-left | Present | Cyanotic | VSD closure, separation of pulmonary arteries from truncus, reattachment to RV |
Complete transposition of the great vessels | Aorta originates from RV; PA originates from LV, with ASD, VSD, and/or PDA | Right-to-left | Not present | Cyanotic | Most common surgical correction is arterial switch procedure |
Total anomalous pulmonary venous return (TAPVR) | Pulmonary veins return oxygenated blood to the RA, ASD | Right-to-left | Not present | Cyanotic | Surgical connection of pulmonary veins to LA, ASD closure |
Tricuspid atresia | Agenesis of tricuspid valve, small RV, large LV | Right-to-left | Sometimes | Cyanotic | Surgical connection of PA to RA, ASD and VSD closure |
4. Profound cyanosis may occur if the ventricular septum is intact or the ductus arteriosus begins to close.
5. In this situation oxygen therapy has a limited benefit because the only oxygenated blood reaching systemic circulation is that which is shunted through the VSD, ASD, or PDA.
6. This amount is determined by the size, position, and number of communications where shunting can occur.
7. The most common surgical repair is the arterial switch procedure.
N Total anomalous pulmonary venous return (TAPVR) (Figure 27-15)
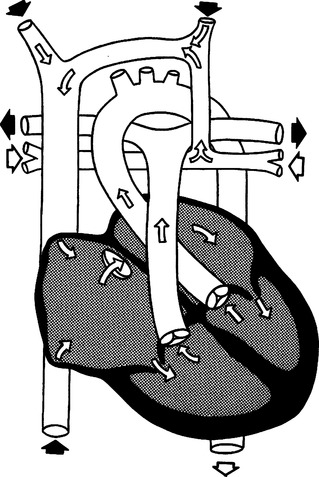
1. Cyanosis and right-to-left shunting are present.
2. Characterized by all pulmonary veins returning oxygenated blood to the right atrium.
3. Several types of TAPVR are classified according to route of pulmonary venous return to the right atrium.
a. Supracardiac TAPVR is the most common and involves return through the superior vena cava.
b. Infracardiac TAPVR involves return through the portal venous system.
c. Cardiac TAPVR is characterized by direct communication between the pulmonary veins and the right atrium or through the coronary sinus.
4. To sustain life, there must be a passage between the right and left atria (ASD) so that right-to-left shunting can occur.
5. Surgical management consists of reimplantation of anomalous pulmonary veins from the right atrium to the left atrium and closure of the ASD.
O Tricuspid atresia (Figure 27-16)
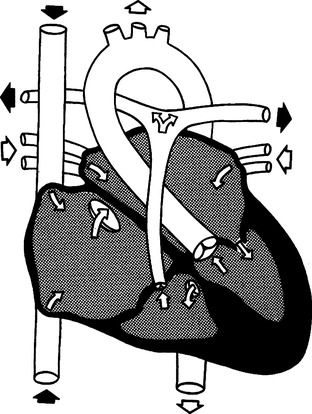
1. Cyanosis is present in varying degrees because of right-to-left shunt.
a. Failure of the tricuspid valve to form, resulting in lack of direct communication between the right atrium and right ventricle.
3. Right-to-left shunting occurs through an ASD.
4. A VSD is sometimes present.
5. Transposition of the great arteries is also present in approximately 30% of the cases.
6. Surgical management of the neonate consists of creating a shunt between the aorta and the pulmonary artery to increase pulmonary blood flow.
7. Definitive repair involves connecting the pulmonary artery to the right atrium and closure of ASD and VSD if present.
XI Other Congenital Anomalies with Effects on the Respiratory System
1. Characterized by blockage, to varying degrees, of the posterior opening into the nasopharynx by a membranous tissue, usually with bony implants.
2. Occurs in approximately 1 in 7000 live births.
3. Can be an isolated anomaly or part of a syndrome with multiple anomalies.
5. Computerized tomography scan usually confirms diagnosis of choanal atresia.
B Esophageal atresia (EA) (Figure 27-17)
1. Characterized by an esophagus that ends in a blind pouch before joining the stomach and usually occurs in conjunction with a tracheoesophageal fistula (TEF).
2. There are several types; the most common involves atresia of the upper esophagus with the lower esophagus connected to the trachea or mainstem bronchi.
a. Most infants with EA have a history of maternal polyhydramnios or an abnormally high level of amniotic fluid.
b. Excessive amounts of oral secretions, choking, and respiratory distress soon after birth
c. Inability to swallow feedings
d. A gastric sump tube coiled in the blind pouch of the proximal esophagus is commonly seen on radiographs.
e. Air in the stomach and intestines seen on radiographs if TEF is present.
C Congenital diaphragmatic hernia (CDH) (Figure 27-18)
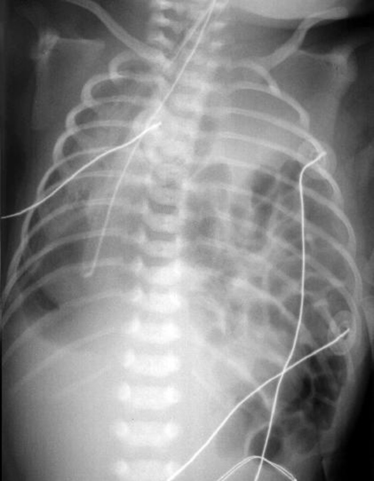
1. Characterized by incomplete development of diaphragm, the presence of abdominal organs in the thoracic cavity, and small, underdeveloped lung(s).
2. CDH occurs in 1 of every 4000 live births.
3. At least 90% of occurrences of CDH involve the left side, allowing the intestines to migrate into the chest.
4. The primary factors that influence outcome are
5. Antenatal diagnosis is confirmed by ultrasound.
6. Early diagnosis allows the mother to plan for delivery at a high-risk perinatal center.
a. Orogastric tube placement to collapse stomach and prevent bowel distention from causing further compression of the lung.
b. Intubation and mechanical ventilation are frequently necessary to stabilize respiratory distress.
c. If the pulmonary dysfunction is severe, other therapies include
d. Early surgical repair is sometimes performed if the CDH causes little or no pulmonary dysfunction.
XII Nonrespiratory Disorders of Preterm Neonates
A Intraventricular hemorrhage (IVH)
1. Significant cause of brain injury in premature infants, particularly those weighing <1500 g or born at <32 weeks’ gestation.
a. Occurs early in life: Nearly 90% of cases occur during the first 3 days, and 50% occur during the first 24 hours postpartum.
3. Etiology is multifactorial, but all risk factors relate in some way to an alteration in the infant’s cerebral blood flow.
a. Vaginal delivery, breech presentation, and birth asphyxia
b. Severe respiratory distress, pneumothorax
a. The most common location of bleeding is in the germinal matrix, a highly vascular area of the brain that gives rise to neurons during the development of the nervous system.
b. Preterm neonates are vulnerable to fluctuations in hemodynamics because they lack a supporting network to protect the fragile blood vessels in the germinal matrix.
c. The network of fibers that support these capillary beds develops during the period between 24 and 32 weeks’ gestation.
d. Hemodynamic instability is common in premature neonates. Appropriate systemic blood pressure ranges are not well established.
5. Clinical presentation of IVH varies from subtle to catastrophic.
a. Twenty-five percent to 50% of cases are “silent” and can only be diagnosed using cranial ultrasound.
b. Common signs may be present.
c. IVH is classified from grade 0 to grade III or grade IV depending on the system used, with higher numbers corresponding to increasing severity of bleeding.
6. Management involves supportive care to prevent extension of the IVH.
B Necrotizing enterocolitis (NEC) is an inflammatory bowel process resulting in injury to the intestinal mucosa.
a. NEC occurs in 1 in 1000 live births and in approximately 5% of premature infants of <1500 g.
b. Prematurity is the primary risk factor and is the only risk factor in 20% of all cases.
c. Associated risk factors include hypoxia and gastrointestinal infection.
d. NEC is seen in 5% of term infants and has been associated with congenital heart disease, polycythemia, and birth asphyxia.
a. The premature neonate develops inflammation and ischemia in the intestinal mucosa.
b. Tissue damage extends to the muscular and subserosal layers of the bowel, causing areas of hemorrhage, necrosis, and accumulation of gas.
c. The classic sign of NEC is a radiograph showing pneumatosis or air trapped in the walls of the intestine.
a. If the infant is stable, supportive care consists of bowel rest, fluid resuscitation, and antibiotics.
b. Up to one third of infants with NEC develop an intestinal stricture that requires surgery.
c. Close monitoring for signs of intestinal gangrene and intestinal perforation
d. Signs of gangrene without intestinal perforation include
e. Intestinal perforation and pneumoperitoneum require immediate surgical intervention.