14 Respiratory Alterations and Management
After reading this chapter, you should be able to:
• describe the pathophysiological mechanisms of acute respiratory failure (ARF) and key principles of patient management
• differentiate between hypoxaemic (type I) and hypercapnoeic (type II) respiratory failure
• outline the incidence of respiratory alterations in the Australasian critical care context
• discuss the aetiology, pathophysiology, clinical manifestations and management of common respiratory disorders managed in intensive care, specifically pneumonia, respiratory epidemics, asthma, chronic obstructive pulmonary disease (COPD), acute lung injury (ALI) and pneumothorax
• describe the evidence base for key components of nursing and collaborative practice involved in the management of patients with ARF in ICU
• outline the principles and immediate postoperative management for lung transplant recipients.
acute respiratory distress syndrome
hypoxaemic respiratory failure
Introduction
The most common reason that patients require admission to an intensive care unit (ICU) is for support of their respiratory system. Over the last decade, almost half of all patients admitted to ICU in Australia and New Zealand required mechanical ventilation;1 a statistic of 41% in 2008.2 Failure or inadequate function of the respiratory system occurs as a result of direct or indirect pathophysio-logical conditions. The process of mechanical ventilation may also injure a patient’s lungs, further impacting functioning of the respiratory system. Preventing or minimising ventilator-associated lung injury is therefore also a primary goal of patient care. Chapter 13 described the relevant anatomy and physiology and assessment and monitoring practices for a patient with life-threatening respiratory dysfunctions. This chapter describes the incidence, pathophysiology, clinical manifestations and management of common respiratory disorders that result in acute respiratory failure, specifically pneumonia (including discussion of respiratory epidemics), asthma, chronic obstructive pulmonary disease (COPD), acute lung injury (ALI), pneumothorax and lung transplantation. Discussion of oxygenation and ventilation strategies to support respiratory function during a critical illness is presented in Chapter 15.
Incidence of Respiratory Alterations
Respiratory diseases are common and affect significant numbers of the population in Australia, accounting for almost half of all hospital admissions.3 These diseases are also the most common illness responsible for emergency admission to hospital, the most common reason to visit a general practitioner and represent the most commonly reported long-term illnesses in children.4 Despite these findings, the incidence of respiratory alterations is difficult to quantify as the number of patients who require admission to hospital as a result of respiratory disease represent a small proportion of the total number affected. Further, patients who require admission to ICU as a result of respiratory disease represent only a fraction of all hospital admissions.5,6
Data presented in Table 14.17 illustrates the total number of patients (adults and children) admitted to hospital as a result of a range of respiratory diseases. While it is difficult to determine the number of patients in each diagnostic group who required admission to ICU as part of their management, ICU admissions account for around 4% of all overnight hospital admissions.5 Infective processes (influenza and pneumonia), COPD and asthma represent the three largest groups of hospital admissions. Conditions such as adult respiratory distress syndrome (ARDS), pneumothorax, pulmonary embolus and pulmonary oedema are relatively small. It should be noted, however, that these conditions often evolve throughout the course of an illness6 and may not therefore be included as the reason for admission. Common respiratory-related ICU presentations are discussed in the following sections.
TABLE 14.1 Incidence of respiratory alterations in Australia 2007–20087
Disorder | Hospital admissions | |
---|---|---|
n | % | |
Adult Respiratory Distress Syndrome | 202 | 0.06 |
Asthma | 37,641 | 10.40 |
COPD (acute exacerbation) | 56,249 | 15.54 |
Influenza and pneumonia | 70,232 | 19.41 |
Lung transplantation | 91 | 0.03 |
Pneumothorax | 3,177 | 0.88 |
Pulmonary embolus | 9,234 | 2.55 |
Pulmonary oedema | 902 | 0.25 |
Total | 177,728 | 49.11 |
Respiratory Failure
Respiratory failure occurs when there is a reduction in the body’s ability to maintain either oxygenation or ventilation, or both. It may occur acutely, as observed in pneumonia and ARDS or it may exist in chronic form, as observed in asthma and COPD. Respiratory failure, and the disorders that cause it, are responsible for a high proportion of death and disability throughout the world.6
Aetiology of Respiratory Failure
• decreased respiratory drive may be caused by brain trauma, drug overdose or anaesthesia/sedation
• decreased respiratory muscle strength may be caused by Guillain–Barré syndrome, poliomyelitis, myasthenia gravis or spinal cord injury
• decreased chest wall expansion may be caused by postoperative pain, rib fractures or a pneumothorax
• increased airway resistance may be caused by asthma or COPD
• increased metabolic oxygen requirements may be caused by severe sepsis
• decreased capacity for gas exchange may be caused by impairment in either ventilation (e.g. pulmonary oedema, pneumonia, acute lung injury, COPD) or pulmonary perfusion (e.g. pulmonary embolism), or a combination of the two.
Importantly, respiratory failure can be an acute or chronic condition. While acute respiratory failure (ARF) is characterised by life-threatening alterations in function, the manifestations of chronic respiratory failure are more subtle and potentially more difficult to diagnose. Patients with chronic respiratory failure often experience acute exacerbations of their disease, also resulting in the need for intensive respiratory support.6
Pathophysiology
Respiratory failure occurs when the respiratory system fails to achieve one or both of its essential gas exchange functions: oxygenation or elimination of carbon dioxide, and can be described either as type I (primarily a failure of oxygenation) or type II (primarily a failure of ventilation).6
Type I Respiratory Failure
A patient with type I (‘hypoxaemic’) respiratory failure presents with a low PaO2 and a normal or low PaCO2. Hypoxaemic respiratory failure may be caused by a reduction in inspired oxygen pressure (e.g. such as extreme altitude), hypoventilation, impaired diffusion or ventilation-perfusion mismatch. Most major respiratory alterations cause this type of failure, usually as a result of hypoventilation due to alveolar collapse or consolidation, or a perfusion abnormality.6
When there is mismatch between ventilation and perfusion in the lungs, exchange of gases is impaired and hypoxaemia ensues (see Figure 14.1):6
• In some cases, there may be reduced ventilation to a certain area of lung tissue (e.g. pulmonary oedema, pneumonia, atelectasis, ARDS). A severe form of mismatch known as intrapulmonary shunting occurs when adequate perfusion exists but there are sections of lung tissue that are not ventilated. In these alveoli, the oxygen content is similar to that of the mixed venous blood and the CO2 is elevated.
• In other instances, ventilation may be adequate but perfusion is impaired (e.g. pulmonary embolus). In its severe form, this is known as dead space ventilation as the lungs continue to be ventilated but there is no perfusion, and therefore no gas exchange. In this situation, the alveolar oxygen content is similar to that of the inspired gas mixture and the CO2 is minimal6 (see Chapter 13 for further discussion).
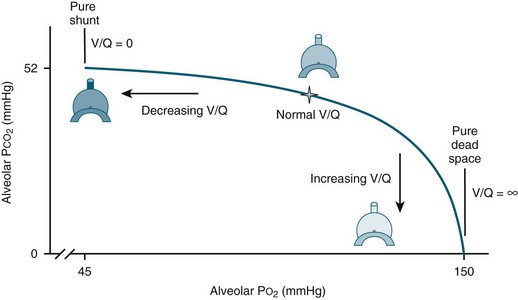
FIGURE 14.1 Ventilation-perfusion mismatches.6 Ventilation-perfusion (V/Q) ratio displays the normal balance (star) between alveolar ventilation and vascular perfusion allowing for proper oxygenation. When ventilation is reduced, the V/Q ratio decreases, in the most extreme case resulting in pure shunt, where V/Q = 0. When perfusion is reduced, the V/Q ratio increases, in the most extreme case resulting in pure dead space, where V/Q = infinite (∞). (published with permission)
Clinical Manifestations
Patient presentations in acute respiratory failure can be quite diverse and are dependent on the underlying pathophysiological mechanism (e.g. hypercapnoea and/or hypoxaemia), the specific aetiology and any comorbidities that may exist.6 Specific clinical manifestations for the clinical disorders discussed in this chapter are provided in each section. Dyspnoea is the most common symptom associated with ARF; this is often accompanied by an increased rate and reduced depth of breathing and the use of accessory muscles. Patients may also present with cyanosis, anxiety, confusion and/or sleepiness.4
A systematic approach to clinical assessment and management of patients with ARF is crucial, given the large number of possible causes. Clinical investigations to assess the cause of respiratory failure vary depending on the suspected underlying aetiology and the progression of disease. Continuous monitoring of oxygen saturation using pulse oximetry, arterial blood gas (ABG) analysis and chest radiograph assessment are used in almost all cases of respiratory failure.8 Other more specialised tests such as computed tomography (CT) of the chest and microbiological cultures may be used in specific circumstances.9 With ABG analysis, the measurement of PaO2, PaCO2, Alveolar–arterial (A–a) PO2 difference and the patient response to supplemental oxygen are key elements in determining the cause of ARF (see Chapter 13).
Independent Nursing Practice
The primary survey (airway, breathing and circulation) and immediate management form initial routine practice.10 Frequent assessment and monitoring of respiratory function, including a patient’s response to supplemental oxygen and/or ventilatory support, is the focus. Patient comfort and compliance with the ventilation mode, ABG analysis and pulse oximetry guide any titration of ventilation. The key goals of management are to treat the primary cause of respiratory failure, maintain adequate oxygenation and ventilation and prevent or minimise the potential complications of positive pressure mechanical ventilation.
Maintaining Oxygenation and Ventilation
The therapeutic aim is to titrate the fraction/percentage of inspired oxygen (FiO2) to achieve a PaO2 of 65–70 mmHg and to maintain minute ventilation to achieve PaCO2 within normal limits where possible.6 Oxygen is not a drug, therefore it does not require prescription for use. Nursing staff in ICU are therefore commonly responsible for titration of oxygen therapy to maintain a specific PaO2 or SpO2, and the alteration of respiratory rate and/or tidal volume to maintain a specified PaCO2. One concern that often arises, particularly with patients who require high concentrations of oxygen, is the risk of oxygen toxicity. The link between prolonged periods of oxygen concentrations approaching 100% and oxidant injuries in airways and lung parenchyma has been established, although mostly from animal research. Although it remains unclear how these data apply to human populations, most consensus groups have argued that FiO2 values less than 0.4 are safe for prolonged periods of time and that FiO2 values of greater than 0.8 should be avoided if possible6 (see Chapter 15 for further discussion of oxygenation).
Ventilator-associated lung injury is also a concern when managing patients with acute respiratory failure. A lung can be injured when it is stretched excessively as a result of tidal volume settings that generate high pressures, often referred to as barotrauma or volutrauma. The most common injury is that of alveolar rupture and/or air in the pleural space (pneumothorax).6 An approach known as ‘lung protective ventilation’ aims to minimise overdistension of the alveoli through careful monitoring of tidal volumes and airway pressures. This method should be considered for all ventilated patients. The approach may result in tolerance of higher PaCO2 than normal in patients presenting with acute lung injury or ARDS (see Chapter 15 for further discussion).
Development of ventilator-associated respiratory muscle weakness has been reported as a significant issue when the respiratory muscles are rendered inactive through adjustment of ventilator settings and administration of pharmacotherapy. While it is not yet possible to provide precise recommendations for interventions to avoid this, clinicians are advised to select ventilator settings that provide for some respiratory muscle use.11
Prevention or minimisation of complications associated with positive pressure mechanical ventilation remains a major focus of nursing practice. These complications may relate to the patient–ventilator interface (artificial airway and ventilator circuitry), infectious complications such as ventilator-associated pneumonia (VAP) or complications associated with sedation and/or immobility. Some common complications and the appropriate management strategies are briefly outlined in Table 14.26,12–14 and discussed further in Chapter 15.
TABLE 14.2 Complications of mechanical ventilation and associated management strategies
Patient–ventilator interface complications | |
Airway dislodgement/disconnection | Endotracheal tube (ETT) or tracheostomy tube is secured to optimise ventilation and prevent airway dislodgement or accidental extubation. |
Circuit leaks | Cuff pressure assessmentCircuit checksExhaled tidal volume measurement |
Airway injury from inadequate heat/humidity | Maintain humidification of the airway using either a heat-moisture exchanger or a water-bath humidifier. |
Obstructions from secretions | Assess the need for suctioning regularly and suction as required. |
Tracheal injury from the artificial airway | Assessment of airway placement and cuff pressure (minimal occlusion method) |
Infectious complications | |
Ventilator-associated pneumonia (VAP) |
Collaborative Practice
A patient with ARF requires extensive multidisciplinary collaboration between nurses, physiotherapists, specialist medical staff, speech and occupational therapists, dietitians, social workers, radiologists and radiographers. Patients may require additional oxygen delivery through an adequate haemoglobin level for oxygen transportation and a cardiac output sufficient to supply oxygenated blood to the tissues.6 At times this may require blood transfusion and/or the use of vasoactive medications (see Chapters 11 and 20).
Chest physiotherapy is a routine activity for managing patients with ARF. This involves positioning, manual hyperinflation, percussion and vibration and suctioning. The evidence base for these techniques is limited, however, with a systematic review not demonstrating an improvement in mortality.12 Guidelines for physiotherapy assessment have enabled identification of patient characteristics for treatments to be prescribed and modified on an individual basis.13 Table 14.36,13,15 outlines a number of collaborative practice issues for patients with respiratory failure, particularly those who may require prolonged mechanical ventilation.
TABLE 14.3 Collaborative practices for patients with respiratory failure
Long-term patient management | Best practice |
---|---|
Timing of tracheostomy insertion | Where mechanical ventilation is expected to be 10 days or more, tracheostomy should be performed as soon as identified. Early tracheostomy is associated with less nosocomial pneumonia, reduced ventilation time and shorter ICU stay. |
Weaning protocols | Specific plan is patient dependent; better outcomes are achieved when there is an agreed and well communicated weaning plan (see Chapter 15) |
Nutrition | Consider adequate nutrition for physiological needs – important to not overfeed as this increases CO2 production and need to have balance of vitamins and minerals |
Swallow assessment | Assess for dysphagia |
Mobilisation | Sitting out of bed, mobilising (see Chapter 4) |
Communication | Communication aids, speaking valves |
Activities | Activity plan/routine, entertainment (TV/Films), visitors, outings |
Sleep | Clustering cares, reducing stimuli to promote sleep (see Chapter 7) |
Family support | Importance of providing physical, emotional and/or spiritual support to family members (see Chapter 8) |
Tracheostomy follow-up | Outreach team: follow-up care by nurses experienced in tracheostomy care can prevent complications and improve outcomes |
End-of-life decisions in ARF | see Chapter 5 |
Medications
Medications commonly prescribed in respiratory failure include inhalation steroids and bronchodilators, intravenous steroids and bronchodilators, antibiotic therapy, analgesia and sedation to maintain patient–ventilator synchrony, but may also involve nitric oxide, glucocorticoid or surfactant administration. A patient’s condition, comorbidities and the above-mentioned pharmacological therapy may also be supported with inotropic and other resuscitation therapies (see Chapter 11). As the use of medications will vary depending on the underlying cause of respiratory failure, these are discussed in each section respectively.
Special Considerations
Respiratory failure in patients who are pregnant, elderly or have comorbidities require specific attention to avoid clinical deterioration. Respiratory physiology and the respiratory tract itself are altered during pregnancy; this may result in exacerbation of preexisting respiratory disease or increased susceptibility to disease (see Chapter 26). Upper airway mucosal oedema may increase the likelihood of upper respiratory tract infection. Lung function and lung volume are also altered, compensated by an increase in respiratory drive and minute ventilation. The impact of these alterations on chronic conditions such as asthma/COPD and acute illness are explored in the subsequent sections. The impact on the fetus of infection, hypoxia and drug therapy is an important consideration.6
The elderly have ageing organs and systems and other comorbidities that may exacerbate their respiratory dysfunction. Drug metabolism and excretion is slowed, complicating drug dosing and response.16 Metabolism of anaesthetic agents is slower due to the diminished physio-logy of ageing organs. Common comorbidities may also be present, including obesity, heart disease, diabetes, and renal impairment or muscle wasting. Pneumonia is a common presentation in the elderly and is often exacerbated by chronic lung conditions.6
Post-anaesthesia Respiratory Support
Short-term respiratory support may be required after major surgery, in cases of extended anaesthesia, preexisting comorbidities and/or diminished physical reserve (e.g. elderly, patients with obstructive sleep apnoea). Most patients requiring ventilation in the early postoperative period have had cardiothoracic surgery, and so much of the available research relates to this patient group (see also Chapter 12).
Preoperative assessment and management is a key factor in preventing respiratory complications. This involves optimising physical condition and nutritional status, planning the timing of surgery to reduce the likelihood of preexisting respiratory infection and patient education regarding the importance of respiratory support, including postoperative mobilisation and physiotherapy. Patients with suspected or confirmed chronic conditions require a thorough diagnostic work-up prior to surgery to determine the best management strategy in the postoperative period.17
The key focus in management of postoperative ventilation is to limit ventilation time, as prolonged ventilation time is associated with poor outcome. Once a patient has reached normothermia, is haemodynamically stable, responsive and has adequate analgesia, weaning of ventilation is commenced. Rapid and/or nurse-led weaning protocols are often implemented to minimise delays in the weaning process. Anaesthetic care in these patients includes use of short-acting or regional anaesthesia (e.g. epidural analgesia) to minimise respiratory depression.18
Pneumonia
Pathophysiology
The normal human lung is sterile, unlike the gastrointestinal tract and upper respiratory tract which have resident bacteria. A number of defence mechanisms exist to prevent microorganisms entering the lungs, such as particle filtration in the nostrils, sneezing and coughing to expel irritants and mucus production to trap dust and infectious organisms and move particles out of the respiratory system. Infection occurs when one or more of these defences are not functioning adequately or when an individual encounters a large amount of microorganisms at once and the defences are overwhelmed.6 An invading pathogen provokes an immune response in the lungs, resulting in the following pathophysiological processes:
• alteration in alveolar capillary permeability that leads to an increase in protein-rich fluid in the alveoli; this impacts on gas exchange and causes the patient to breathe faster in an effort to increase oxygen uptake and remove CO2
• mucous production increases and mucous plugs may develop which block off areas of the lung, further reducing capacity for gas exchange
• consolidation occurs in the alveoli, filling with fluid and debris; this occurs particularly with bacterial pneumonia where debris accumulates from the large number of white blood cells involved in the immune response.6
Aetiology
Pneumonia is caused by a variety of microorganisms, including bacteria, viruses, fungi and parasites. In many cases, the causative organism may not be known and current practice in many cases is to initiate antimicrobial treatment as soon as possible, based on symptoms and patient history, rather than waiting for microorganism culture results.19 The true incidence of pneumonia is not well known as many patients do not require hospitalisation. Different ages and characteristics of the patient are often associated with different causative organisms. Viral pneumonias, especially influenza, are most common in young children, while adults are more likely to have pneumonia caused by bacteria such as Streptococcus pneumoniae and Haemophilus influenzae. Pneumonia is a particular concern among elderly adults as they experience an increase in the frequency and severity of pneumonia.6
Table 14.47 outlines the principal diagnoses of patients hospitalised with pneumonia in Australia during 2007–2008. This information reflects the high proportion of viral pneumonia and the large number of cases where the causative organism may not be known.
TABLE 14.4 Principal diagnoses of patients hospitalised with pneumonia in Australia during 2007–2008
Principal Diagnosis | Hospitalisations | |
---|---|---|
n | % | |
Pneumonia due to identified influenza virus | 1668 | 2.4 |
Influenza, virus not identified | 1429 | 2.0 |
Viral pneumonia, not elsewhere classified | 1899 | 2.7 |
Pneumonia due to Streptococcus pneumoniae | 1331 | 1.9 |
Pneumonia due to Haemophilus influenzae | 1029 | 1.5 |
Bacterial pneumonia, not elsewhere classified | 3184 | 4.5 |
Pneumonia due to other infectious organisms, not elsewhere classified | 292 | 0.4 |
Pneumonia, organism unspecified | 59,389 | 84.6 |
Total | 70,232 | 100.0 |
Community-acquired Pneumonia
Clinical assessment, especially patient history, is important in distinguishing the aetiology and likely causative organism in patients with community-acquired pneumonia (CAP). Specific information regarding exposure to animals, travel history, nursing home residency and any occupational or unusual exposure may provide the key to diagnosis.9 Personal habits such as smoking and alcohol consumption increase the risk of developing pneumonia and should be explored. Many patients admitted to hospital or ICU with CAP have comorbidities, suggesting that those who are chronically ill have an increased risk of developing ARF. The most common chronic illnesses involved are respiratory disease (including smoking history, COPD/asthma), congestive cardiac failure and diabetes mellitus.6,20 Table 14.5 outlines aspects of the clinical history associated with particular causative organisms in CAP.6,9,21
TABLE 14.5 Clinical history/comorbidities associated with particular causative organisms in CAP
Condition | Causative organisms |
---|---|
Individual factors | |
Alcoholism | Streptococcus pneumoniae (including penicillin-resistant), anaerobes, gram-negative bacilli (possibly Klebsiella pneumoniae), tuberculosis |
Poor dental hygiene | Anaerobes |
Elderly | group B streptococci, Moraxella catarrhalis, H. influenzae, L. pneumophila, gram-negative bacilli, C. pneumoniae and polymicrobial infections |
Smoking (past or present) | S. pneumoniae, Haemophilus influenzae, Moraxella catarrhalis, Aspergillus spp. |
IV Drug use | S. aureus, anerobes, M. tuberculosis, S. pneumoniae |
Comorbidities | |
COPD | S. pneumoniae, Haemophilus influenzae, Moraxella catarrhalis, Aspergillus spp. |
Post influenza pneumonia | S. pneumoniae, S. aureus, H. influenzae |
Structural disease of lung (e.g., bronchiectasis, cystic fibrosis) | P. aeruginosa, P. cepacia or Staphylococcus aureus |
Sickle cell disease, asplenia | Pneumococccus, H. influenzae |
Previous antibiotic treatment and severe pulmonary comorbidity, (e.g. bronchiectasis, cystic fibrosis, and severe COPD) | P. aeruginosa |
Malnutrition related diseases | Gram-negative bacilli |
Environmental exposure | |
Air conditioning | Legionella pneumophila |
Residence in nursing home | S. pneumoniae, gram-negative bacilli, H. influenzae, S. aureus, Chlamydia pneumoniae; consider M. tuberculosis. Consider anaerobes, but less common. |
Homeless population | S. pneumoniae, S. aureus, H. influenzae, Cryptococcus gattii: caused by inhalation of spores while sleeping, associated with red gum trees (Australia, Southeast Asia, South America) |
Suspected bioterrorism | Anthrax, tularaemia, plague |
Animal exposure | |
Bat exposure | Histoplasma capsulatum |
Bird exposure | Chlamydia psittaci, Cryptococcus neoformans, H. capsulatum |
Rabbit exposure | Francisella tularensis |
Exposure to farm animals or parturient cats | Coxiella burnetii (Q fever) |
Travel history | |
Travel to southwestern USA | Coccidioidomycosis; hantavirus in selected areas |
Travel to southeast Asia | Severe acute respiratory syndrome (coronavirus), Mycobacterium tuberculosis, melioidosis |
Residence or travel to rural tropics | Melioidosis (Burkholderia pseudomallei) |
Travel to area of known epidemic | Avian influenza (H5N1), Swine influenza (H1N1) and SARS (coronavirus) |
The Australian CAP study collaboration20 examined episodes of CAP in which all patients underwent detailed assessment for bacterial and viral pathogens. Aetiology was identified in 46% of episodes, with the most frequent causes being Streptococcus pneumoniae (14%), Mycoplasma pneumoniae (9%) and respiratory viruses (15%). Mechanical ventilation or vasopressor support was required in 11% of cases.
Diagnosis of CAP
Routine screening of patients with suspected pneumonia continues to rely on microscopy and culture of lower respiratory tract specimens, blood cultures, detection of antigens in urine and serology. Methods for detection of antigens are now widely available for several pneumonia pathogens, particularly S. pneumoniae, Legionella and some respiratory viruses.22 Culture of respiratory secretions may be limited due to difficulty in obtaining sputum samples. For this reason, nasopharyngeal aspirates or swabs may be taken as part of the routine screening for CAP.23
Severity assessment scoring
International guidelines recommend a severity-based approach to management of CAP. CURB65, CRB65 and the Pneumonia Severity Index (PSI) are the most widely recommended systems that produce scores and assess severity based on patient demographics, risk factors, comorbidities, clinical presentation and laboratory results.6 Recent evaluation found no significant differences between these systems in their ability to predict mortality.24 The Australian CAP Collaboration team devised and validated the SMART-COP scoring system for predicting the need for intensive respiratory or vasopressor support in patients with CAP. The acronym relates to the factors: low Systolic blood pressure, Multilobar chest radiography involvement, low Albumin level, high Respiratory rate, Tachycardia, Confusion, poor Oxygenation and low arterial pH.25
Hospital-acquired and Ventilator-associated Pneumonia
Hospital-acquired or nosocomial pneumonia is defined as pneumonia occurring more than 48 hours after hospital admission.9 It is the second-most common nosocomial infection and the leading cause of death from infection acquired in-hospital. Ventilator-associated pneumonia (VAP) is a nosocomial pneumonia in patients who are mechanically ventilated. The incidence of VAP is reported at 10–30% among patients who require mechanical ventilation for greater than 48 hours.26
Critically ill ventilated patients commonly experience chest colonisation as a result of translocation of bacteria from the mouth to the lungs via the endotracheal tube (ETT). This may lead to clinical signs of infection, or the patient may remain colonised without an infective process. The patient’s severity of disease, physiological reserve and comorbidity influence the development of infection.6 Most cases (58%) of VAP are associated with infection involving gram-negative bacilli such as Pseudomonas aeruginosa and Acinetobacter spp. A high number of cases (20%) are associated with gram-positive Staphylococcus aureus. Many cases of VAP are associated with multiple organisms.6 As in CAP, the presence of comorbidities and other risk factors influence the causative organism.
Diagnosis and treatment of VAP
VAP can be difficult to diagnose, as clinical features can be non-specific and other conditions may cause infiltrates on chest X-ray (CXR). However, it is often suspected when there are new infiltrates observed on CXR or when clinical signs of infection begin to develop, e.g. new onset of pyrexia, raised white blood cell counts, purulent sputum and a difficulty in maintaining adequate oxygenation.6 Specific risk factors associated with increased mortality in VAP have been identified over the last decade. The most widely-recognised risk factor is the provision of appropriate antibiotic treatment, which has reduced mortality and the rate of complications. Timeliness of antibiotic admini-stration is an independent risk factor for mortality; mortality was increased where administration of antibiotics was delayed for more than 24 hours after diagnosis.26 When VAP is suspected there are two treatment strategies, although a systematic review did not demonstrate any differences in mortality, length of ICU stay or length of ventilation period:19
• Clinical Strategy: involves treatment of patients with new antibiotics, based on patient risk factors and the local microbiologic and resistance patterns. Therapy is adjusted based on culture results and the patient’s response to treatment.
• Invasive Strategy: involves collection and quantitative analysis of respiratory secretions from samples obtained by bronchoalveolar lavage (BAL) to confirm the diagnosis and causative organism. Antibiotic therapy is then guided by specific protocols.
Clinical Manifestations
Symptoms for pneumonia are both respiratory and systemic. Common characteristics include fever, sweats, rigours, cough, sputum production, pleuritic chest pain, dyspnoea, tachypnoea, pleural rub, inspiratory crackles on auscultation, plus radiological evidence of infiltrates or consolidation. Cough is the most common finding and is present in up to 80% of all patients with CAP.6,9
Collaborative Practice
Early recognition of pneumonia and timely administration of antibiotic therapy are key aspects for patient management. The most important aspect of management in VAP is prevention and this is a key emphasis in the care of all mechanically-ventilated patients. One approach in encouraging the implementation of VAP prevention was the combination of four aspects of patient management into one evidence based guideline, known as the Ventilator Care Bundle: elevating the head of bed to 30–45 degrees, daily sedation vacation and assessment of readiness to extubate, peptic ulcer disease (PUD) prophylaxis and deep vein thrombosis (DVT) prophylaxis.27 Effectiveness of this strategy and implementation issues have been further evaluated, with some additional perspectives offered. While it is apparent that daily spontaneous awakening and breathing trials are associated with early liberation from mechanical ventilation and VAP reduction, the strategies included for DVT and PUD prophylaxis do not directly affect VAP reduction. Semi-recumbent positioning has been associated with a significant reduction in VAP but is difficult to maintain in ventilated patients.14 It has been suggested that other methods to reduce VAP, such as oral care and hygiene, chlorhexidine in the posterior pharynx and specialised endotracheal tubes (continuous aspiration of sub-glottic secretions, silver-coated), should be considered for inclusion in a revised Ventilator Bundle more specifically aimed at VAP prevention.14
Development of VAP is attributed in part to aspiration of oral secretions that are colonised by a variety of bacteria. Maintenance of oral hygiene is therefore a key element in the care of mechanically-ventilated patients.6 Oral mucosa and dental plaque may also be colonised with bacteria and the use of an oral antiseptic solution (e.g. Chlorhexidine) may further reduce the risk of developing VAP.28
Supportive ventilation is a key focus for managing patients with pneumonia. In some instances this may include increased oxygen delivery and positive end expiratory pressure (PEEP) to maintain oxygenation and prevent alveolar collapse. Chest physiotherapy assists in the prevention of VAP29 and remains a key component of management of all ventilated patients. However, its contribution towards improving mortality in patients with pneumonia is unclear.30 Upright positioning and early mobilisation are important elements of both prevention and management of pneumonia. The effectiveness of additional strategies, such as use of beds with a continuous lateral rotation or a vibration function to assist in the removal of secretions is yet to be shown.31 See Chapter 15 for further discussion.
Medications
Antibiotic administration is fundamental to a patient’s clinical progress. As noted earlier, the importance of accurate and timely administration of antibiotics directly impacts on patient outcome. In particular, the first dose of antibiotics is required as soon as possible after the diagnosis of pneumonia has been made. Studies where the first dose of antibiotic therapy was delayed showed an increase in mortality.32 Antibiotic cover for pneumonia depends on the causative organism and sensitivity to drugs (see Table 14.66). Review of antibiotic prescribing practices in Australia and New Zealand has shown that prescription of antibiotics in pneumonia is consistent with current guidelines.33
Type of infection | Preferred agent(s) |
---|---|
Community-acquired pneumonia | |
Streptococcus pneumoniae | PCN-susceptible: Penicillin G, amoxicillin, clindamycin, doxycycline, telithromycinPCN-resistant: cefotaxime, ceftriaxone, vancomycin, and fluoroquinolone |
Mycoplasma | Doxycycline, macrolide |
Chlamydophila pneumoniae | Doxycycline, macrolide |
Legionella | Azithromycin, fluoroquinolone (including ciprofloxacin), erythromycin (rifampicin) |
Haemophilus influenzae | Second- or third-generation cephalosporin, clarithromycin, doxycycline, β-lactam/β-lactamase inhibitor, trimethoprim/sulfamethoxazole, azithromycin, telithromycin |
Moraxella catarrhalis | Second- or third-generation cephalosporin, trimethoprim-sulfamethoxazole, macrolide doxycycline, β-lactam–β-lactamase inhibitor |
Neisseria meningitidis | Penicillin |
Streptococci (other than S. pneumoniae) | Penicillin, first-generation cephalosporin |
Anaerobes | Clindamycin, β-lactam–β-lactamase inhibitor, β-lactam plus metronidazole |
Staphylococcus aureus Methicillin-susceptible Methicillin-resistant |
Oxacillin, nafcillin, cefazolin; all rifampin or gentamicin Vancomycin, rifampicin or gentamicin |
Klebsiella pneumoniae and other Enterobacteriaceae (excluding Enterobacter spp.) | Third-generation cephalosporin or cefepime (all aminoglycoside) carbapenem |
Hospital-acquired infections | |
Enterobacter spp. | Carbapenem, β-lactam–β-lactamase inhibitor, cefepime, fluoroquinolone; all + aminoglycoside in seriously ill patients |
Pseudomonas aeruginosa | Anti-pseudomonal β-lactam + aminoglycoside, carbapenem + aminoglycoside |
Acinetobacter | Aminoglycoside + piperacillin or a carbapenem |
Special Considerations
Pneumonia is a leading cause of maternal and fetal morbidity and mortality. It also increases the likelihood of the complications of pneumonia, including requirement for mechanical ventilation. Bacterial pneumonia is the most common type experienced in pregnancy although diagnosis is often delayed as a result of the reluctance to obtain a chest X-ray. Management is similar to a non-pregnant patient with antibiotic therapy adjusted to consider the impact on the fetus.6
CAP is a major cause of morbidity and mortality in elderly patients. Streptococcus pneumoniae is the most common causative organism, with an increase in drug-resistance being reported more widely in the over-65 age group. Treatment of elderly patients with pneumonia is similar to younger patients, with emphasis on supportive care, prevention of sepsis and management of preexisting chronic conditions. Immunisation with pneumococcal and influenza vaccines is beneficial in the prevention of pneumonia in elderly patients.34
Respiratory Pandemics
Serious outbreaks of respiratory infections that spread rapidly on a global scale are termed pandemics. Their spread is so rapid because the infection is usually associated with emergence of a new virus where the majority of the population has no immunity. These infections are characterised by extremely rapid ‘transmission with concurrent outbreaks throughout the globe; the occurrence of disease outside the usual seasonality, including during the summer months; high attack rates in all age groups, with high levels of mortality particularly in healthy young adults; and multiple waves of disease immediately before and after the main outbreak’.35 Several severe respiratory infections have progressed to become pandemics in recent years; these have been associated with the Coronavirus and Influenza viruses. Prediction of the interval between pandemics is difficult, but occurrence is likely to continue and therefore requires that the health care community be well prepared.
Severe Acute Respiratory Syndrome
In 2002–03 an outbreak of a novel Coronavirus occurred in China and rapidly spread throughout the world. The infection was highly virulent with over 8000 cases reported and a mortality rate of 11%. The infection was called Severe Acute Respiratory Syndrome (SARS) due to the severity of the disease, characterised by diffuse alveolar infiltrates, resulting in about 20% of patients requiring respiratory support. The SARS outbreak provoked a rapid and intense public health response coordinated by the World Health Organization (WHO), resulting in a cessation of disease transmission within ten months.35
Influenza Pandemics
A feature of the influenza virus that explains why it continues to be associated with epidemic and pandemic disease is its high frequency of antigenic variation. This occurs in two of the external glycoproteins and is referred to as antigenic drift or antigenic shift, depending on the extent of the variation. The result of this is that new viruses are introduced into the population, and due to the absence of immunity to the virus, a pandemic of influenza results.6
Pandemics of influenza were observed a number of times in the twentieth century, and were believed to have involved viruses circulating in humans that originated from influenza A viruses in birds. The ‘Spanish influenza’ pandemic of 1918–19 resulted in the death of over 50 million people worldwide and remains unprecedented in its severity.35
The first reported infection of humans with avian influenza viruses occurred in Hong Kong in 1997, with six recorded fatalities. The increased virulence of this disease was observed in the acuity of those affected by the outbreak of the highly pathogenic avian influenza virus (H5N1) in 2004–2005.35 Most patients presented with non-specific symptoms of fever, cough and shortness of breath. In many patients this progressed rapidly to ARF requiring ventilation and other supportive measures. The majority of people affected (90%) were less than 40 years of age with case fatality rates highest in the 10–19-year-old age group.36
The most recent influenza pandemic declared by WHO occurred in 2009 when a novel H1N1 influenza A virus emerged in Mexico and the USA. This virus contained genes from avian, human and swine influenza virus and affected millions of people worldwide.37 Patients typically presented with nonspecific flu-like symptoms, however in a quarter of patients this was accompanied by diarrhoea and vomiting. The disease spread globally with millions of cases reported and resulted in over 16,000 deaths by March 2010.38
Australia and New Zealand communities had a high proportion of cases of H1N1 influenza-A infection, with 856 patients being admitted to ICU; 15 times the incidence of influenza A in other recent years. Infants (aged 0–1 years) and adults aged 25–64 years were at particular risk; others at increased risk were pregnant women, adults with a BMI over 35 and indigenous Australian and New Zealand populations. Australian and New Zealand Intensive Care Society (ANZICS) investigators prepared a report based on the Australian and New Zealand experience to assist those in the northern hemisphere to better prepare for their winter influenza season.39
The emergence of novel swine-origin influenza A virus was not anticipated and it is unlikely, given the limitations of current knowledge, that future pandemics can be predicted. The threat of pandemic disease from avian influenza remains high with the rapid evolution of H5N1 viruses; however the direction this will take is unpredictable. Priorities for prevention and management of future influenza pandemics therefore involve development of an international surveillance and response network for early detection and containment of the disease, local preparation for controlling the spread of the infection and further development of vaccines and antiviral agents.38