41 Regional Anesthesia
THE USE OF REGIONAL anesthesia techniques in children has increased dramatically in the past two decades.1–9 Regional anesthesia is most commonly used in conjunction with general anesthesia, although in certain circumstances regional anesthesia may be the sole technique. In addition to central neuraxial blocks, peripheral nerve blocks are now employed with increasing frequency, in part because of the introduction of high-resolution portable ultrasound imaging. Ultrasound-guided visualization of anatomic structures permits both greater precision of needle or catheter placement and confirmation that the drug has been deposited at the site of choice (see Chapter 42). The advent of ultrasound guidance has also facilitated the performance of several blocks, including truncal blocks, which could not be otherwise performed safely using landmark techniques, especially in children. Evidence from recent large-scale collaborative studies of regional blockade in children supports the contention that peripheral nerve blockade is assuming greater prominence in pediatric anesthesia, and data from the Pediatric Regional Anesthesia Network (PRAN) suggests that increased use of ultrasound guidance may be, at least in part, driving this trend.10 Supplementing a general anesthetic with a nerve block can result in a pain-free awakening and postoperative analgesia without the potentially deleterious side effects associated with parenteral opioids (see Chapter 43).11 This benefit may be of particular importance to neonates, former preterm infants, and in children with cystic fibrosis and other conditions.4 There is also evidence that suggests that regional anesthesia may improve pulmonary function in children who have undergone thoracic or upper abdominal surgery.12–15 Lastly, the greatly increased number of “same day surgery” cases in recent years has made the advantages of regional anesthesia, such as the rapid awakening, enhanced postoperative analgesia with no sedation or altered sensorium, and lack of opioid-induced nausea or vomiting, even more apparent. The safe and effective use of these techniques in children, however, requires an understanding of both the developmental anatomy of the region in which the block is placed and the developmental pharmacology of local anesthetics. This chapter will focus on landmark-guided techniques for those who do not have the advantage of ultrasound imaging; ultrasound techniques are described in Chapter 42.
Pharmacology and Pharmacokinetics of Local Anesthetics
There are two classes of clinically useful local anesthetics, the amino amides (amides) and the amino esters (esters) (Table 41-1). The amides are degraded in the liver by cytochrome P450 enzymes, whereas the esters are hydrolyzed primarily by plasma cholinesterases.16–20 These degradation pathways account for some of the differences in distribution and metabolism of local anesthetics, particularly in neonates when compared with adults.
Esters | Amides |
---|---|
Procaine | Lidocaine |
Tetracaine | Mepivacaine |
2-Chloroprocaine | Bupivacaine |
Levobupivacaine | |
Ropivacaine | |
Etidocaine |
Amides
Amide local anesthetics commonly used in children include lidocaine, bupivacaine (and its isomer levobupivacaine), and ropivacaine. The choice of agent most often depends on the desired speed of onset and duration of action of the block, but in small infants and children issues related to potential toxicity are also important. Compared with an adult, the neonatal liver has limited enzymatic activity to metabolize and biotransform drugs (E-Fig. 41-1; see also Chapter 6). The ability to oxidize and to reduce drugs, in particular, is immature.19–25 Clearance is immature in preterm neonates and matures rapidly through infancy. Neonates do not metabolize mepivacaine, with most of it excreted unchanged in the urine.26–32 Conjugation reactions are limited at birth and do not reach adult rates until approximately 3 to 6 months of age.21–2428
Older children also differ from adults with respect to the pharmacokinetics of local anesthetics. Children achieve peak plasma concentrations of amide local anesthetics more rapidly than adults after intercostal nerve blocks, but at similar times (approximately 30 minutes with lidocaine and bupivacaine) after caudal epidural administration.33–35 Ilioinguinal nerve blocks in children weighing less than 15 kg may produce plasma concentrations of bupivacaine in the toxic range if more than 1.25 mg/kg is administered.36
The nature of the epidural space in infants differs from that in the adult with increased vascularity, less fat, and a smaller absorptive surface for local anesthetics. Anatomical studies have shown that the epidural fat is spongy and gelatinous in appearance, with distinct spaces between individual fat globules.37 With increasing age, fat becomes more tightly packed and fibrous. The absorption half-time of epidural levobupivacaine decreases from 0.36 hours at 1 month postnatal age (PNA) to 0.14 hours at 6 months PNA. This, combined with reduced clearance (by the cytochrome P450, CYP3A4), causes a time to maximum plasma concentration (Tmax) to decrease from 2.2 hours at 1 month PNA to reach 80% of the mature value (0.75 hours) by 6 months PNA.38 The steady-state volume of distribution (Vdss) for amides in children is greater than that in adults, whereas their clearances (Cl) are similar.35,39,40 Because the elimination half-life (t1/2) is related to the volume of distribution and clearance,
a larger steady-state volume of distribution directly prolongs the elimination half-life. However it is clearance that determines steady-state concentrations with continuous amide infusion; reduced clearance in neonates implies that repeated doses and continuous infusions will cause an accumulation of local anesthetic (see E-Fig. 41-1).41–43 Thus infusion rates and local anesthetic concentrations must be reduced in this vulnerable age group when prolonged administration of amides are employed for postoperative analgesia.
Bupivacaine
Bupivacaine is highly bound to plasma proteins, particularly to α1-acid glycoprotein. It is a racemic mixture of the levorotary and dextrorotary enantiomers; the l-isoform is the bioactive one with regard to clinical effect, and the d-isoform contributes more to toxicity. Levobupivacaine, the l-enantiomer of bupivacaine, retains similar efficacy and duration of blockade as the racemic formulation (demonstrated in both an ovine model and in adult volunteers), yet carries up to a 30% reduced risk of cardiac and central nervous system (CNS) toxicities.44,45 Although levobupivacaine’s beneficial toxicity profile has resulted in its widespread use, it is currently unavailable in the United States.46
Several new experimental preparations of bupivacaine (for example, the recently approved liposome extended-release formulation) have the prospect to dramatically prolong analgesia with a reduced potential for toxicity.47–49 Bioerodible encapsulated microspheres of bupivacaine administered for peripheral neural blockade50 release local anesthetic over many hours to several days, depending on the formulation of the microsphere, thus producing very prolonged analgesia.51 The addition of dexamethasone to the microspheres prolongs the block up to 13-fold, and plasma bupivacaine concentrations in animal studies were far below the toxic threshhold.52 No adverse local reactions were noted. Several different preparations have been developed and studied, including synthetic bioerodible microspheres, protein-lipid-sugar spheres, or lipospheres.53–55 The first such preparation (Exparel, Pacira Pharmaceutical, Inc., Parsippany, N.J.) has recently been approved for use in patients 18 years of age or older by the FDA for surgical incision pain; toxicology studies suggest a low risk because of the slow rate of drug release.47,56–58 If this and other formulations become available for use in children, they may be particularly useful for those who cannot have an indwelling catheter for a regional anesthetic, but still require prolonged neural blockade for analgesia. Potential applications include intercostal blockade for children with rib fractures, postoperative analgesia for ambulatory surgery, and children in whom an indwelling epidural catheter poses an excessive risk of infection.59
Ropivacaine
Like levobupivacaine, ropivacaine is an l-enantiomer that has reduced risks of cardiac and neurologic toxicities compared with bupivacaine.60 The lethal dose in 50% of animals (LD50) appears to be greater than that of bupivacaine. Rats of different maturity exhibit a threefold greater tolerance to equipotent doses of ropivacaine than of bupivacaine, when administered for a femoral nerve block.61 Ropivacaine is also reputed to produce a less dense motor block at equianalgesic potency to other local anesthetics, although the data are conflicting in this regard. Some studies report a greater sparing of motor function compared with bupivacaine, whereas others have report no difference in motor and sensory block. Ropivacaine produces a denser blockade of the Aδ and C fibers than bupivacaine when low concentrations are used, lending mechanistic credence to the idea of differential blockade.62 Much of the infant animal data, however, do not support the existence of a greater sensorimotor differential block than that after bupivacaine. The few clinical studies in infants and children currently available do not report a detectable motor-sensory differential, in contrast to the data in adults.61,63 Several clinical studies in infants and children report a prolonged duration of analgesia with ropivacaine, despite using a solution of reduced potency.63–65 Although there are still only limited data available in children, the decreased potential for toxicity makes ropivacaine an attractive agent in this age group. Most clinical studies have used a 0.2% solution (2 mg/mL) with a volume of drug that was similar to that of bupivacaine but that depended on the type of block and size of the child. We use concentrations of 0.1% for infusions with opioid for continuous postoperative analgesia.
Lidocaine
Because lidocaine has a relatively short duration of action compared with bupivacaine and ropivacaine, it is not commonly used for single injection blocks in pediatric regional anesthesia, where a prolonged effect for postoperative analgesia is usually a priority. However, it can be used effectively in continuous blocks where the drug is continuously infused via a catheter, although here too, ropivacaine, levobupivacaine and bupivacaine are far more commonly employed. In vitro laboratory experiments have suggested that lidocaine might have greater potential for neurotoxicity in the developing nervous system than other local anesthetics, although the clinical implications of these findings remain unclear and unproved.66
Esters
The pharmacokinetics of the ester local anesthetics are also affected by the quantitative and qualitative difference in plasma proteins. Plasma pseudocholinesterase activity in neonatal umbilical blood is decreased compared with adults.67 The impact of this reduced activity on clearance is less certain. Other drugs that depend on plasma cholinesterase have mature clearance at birth (e.g., remifentanil). Limited data suggest that 2,3-chloroprocaine may be safe for neonatal regional techniques, and that potentially toxic accumulation does not occur after several hours of use with a 1.5% concentration.68,69
Another enzymatic system with decreased activity in neonates is methemoglobin reductase, which is responsible for maintaining hemoglobin in a reduced valence state where it is capable of binding and transporting oxygen. Hepatic metabolism of prilocaine yields o-toluidine, which can produce methemoglobinemia, thereby rendering red blood cells less capable of carrying oxygen.70 The decreased activity of methemoglobin reductase and the increased susceptibility of fetal hemoglobin to oxidization make prilocaine an unsuitable local anesthetic for use in neonates. Although prilocaine is no longer available for use in the United States as an injected local anesthetic, it is one of the components of EMLA cream (Eutectic Mixture of Local Anesthetics, AstraZeneca, Wilmington, Del.), a commonly employed transdermal local anesthetic. The total dose and surface area for EMLA application is therefore limited in neonates because methemoglobinemia has been reported; however, the dose must be carefully chosen even in infants and toddlers.71 Other local anesthetics, particularly topical agents, such as benzocaine, are potentially dangerous in infants because of the risk of methemoglobinemia by this same mechanism.72 EMLA should only be applied to normal intact skin in appropriate doses73 (less than 5 kg, 1 g applied to a maximum of approximately 10 cm2 surface area; 10 to 20 kg, 10 g applied to a maximum of approximately 100 cm2 surface area; greater than 20 kg, 20 g applied to a maximum of approximately 200 cm2 surface area. The dose must be reduced if EMLA is applied to mucosal surfaces (such as the glans of the penis) compared with intact skin. The duration of action is 1 to 2 hours after the cream is removed. Adverse reactions include skin blanching, erythema, itching, rash, and methemoglobinemia. EMLA should be used with caution and with strict attention to the amount of cream and surface area of application in children younger than 1 month of age. Infants receiving drugs that may induce methemoglobinemia, such as phenytoin, phenobarbital, and sulfonamides may be at increased risk, and caution is warranted. It is contraindicated in children with congenital or idiopathic methemoglobinemia. Topical amethocaine (Ametop) has a more rapid onset of analgesia and increased depth of penetration through the skin and produces minimal vasoconstriction of the skin compared with EMLA.74 Iontophoresis of lidocaine can also produce excellent transdermal skin analgesia with much faster onset of action (10 minutes) and greater depth of skin penetration than EMLA.74
Toxicity of Local Anesthetics
With the exception of uncommon effects, such as producing methemoglobinemia, the major toxic effects of local anesthetics are on the cardiovascular system and the CNS. Local anesthetics readily cross the blood-brain barrier to cause alterations in CNS function. A consistent sequence of symptoms can be observed as plasma local-anesthetic concentrations progressively increase, although this may not be readily apparent in infants and small children. Because of the smaller threshold for cardiac toxicity with bupivacaine, cardiac and CNS toxicity may occur virtually simultaneously in infants and children, or cardiac toxicity may even precede CNS toxicity. During the intraoperative use of bupivacaine, the risk of cardiac toxicity may be increased by the concomitant use of inhalational anesthetics and the CNS effects of the general anesthetic may obscure the signs of CNS toxicity until devastating cardiovascular effects are apparent.75
In awake adults, the earliest symptom of local-anesthetic toxicity is circumoral paresthesia, which is because of the high tissue concentrations of local anesthetic rather than CNS effects. The development of circumoral paresthesia is followed by the prodromal CNS symptoms of lightheadedness and dizziness, which progress to both visual and auditory disturbances, such as difficulty in focusing and tinnitus. Objective signs of CNS toxicity during this time are shivering, slurred speech, and muscle twitching. As the plasma concentration of local anesthetic continues to increase, CNS excitation occurs, resulting in generalized seizures. Further increases in the local anesthetic concentration depresses the CNS, with respiratory depression leading to a respiratory arrest. In adults, cardiovascular toxicity usually follows CNS toxicity. In this case, the systemic blood pressure decreases because the peripheral vasculature dilates, and because of direct myocardial depression, leading to a progressive bradycardia. These effects culminate in a cardiac arrest. In large doses, bupivacaine produces ventricular dysrhythmias, including ventricular tachycardia, peaked T waves, and ST segment changes suggestive of myocardial ischemia, especially when epinephrine-containing solutions are used. Bupivacaine has a particularly strong affinity for the fast sodium channels, as well as the calcium and slow potassium channels in the myocardium. These effects explain why it is so difficult to resuscitate children from a toxic dose of bupivacaine.76–78 Stereoselectivity of the sodium channel in the open state, however, has not been demonstrated. There is also evidence that the slow or “flicker” potassium channels may play a significant role in bupivacaine toxicity.79
With an intravascular injection of bupivacaine with epinephrine, characteristic changes on the electrocardiogram (ECG) may be seen without any observable symptoms of CNS toxicity. Figure 41-1 shows an ECG tracing obtained during an IV injection of bupivacaine with and without epinephrine. Even a small IV dose of 1 to 2 µg/kg of epinephrine in a 1 : 200,000 solution with 0.25% bupivacaine produces peaked T waves with elevated ST segments, particularly in the lateral chest leads.80–82 As opposed to the serious risk of ischemia and dysrhythmias with larger unintended doses of bupivacaine with epinephrine, small test doses result in only brief, transient changes in the ECG and may therefore be useful in the detection of an intravascular catheter or needle placement. Tachycardia is not a reliable indicator of an intravascular injection of bupivacaine, occurring in only 73% of intravascular injections during general anesthesia. When the ECG effects of bupivacaine, with and without epinephrine, and epinephrine alone were compared in children, the most reliable ECG changes (peaked T waves at 1 minute), and an increase in arterial pressure and heart rate, required the presence of epinephrine.83 It should be noted, however, that these changes in the T waves depended on age, diminishing in responsiveness beyond 8 years of age. These data suggest that careful observation of the ECG during test-dose administration may be a sensitive indicator of unintended intravascular injection of bupivacaine in the child anesthetized with an inhalational anesthetic.84
Plasma protein binding is the most important pharmacologic factor that determines the toxicity of local anesthetics, particularly for amides, because it is the free (unbound) fraction of the drug that produces toxicity. Reduced plasma protein concentrations cause more drug to remain in the unbound active form with greater potential for toxicity (see Chapter 6). Plasma concentrations of α1-acid glycoprotein are less in neonates than in older children, and adults, producing a clinically crucial greater free fraction of amide local anesthetics.41,85–89 Current data suggest that the plasma concentration of free drug may be 30% greater in infants younger than 6 months of age and even greater in preterm infants than in adolescents90 as a result of reduced clearance and reduced α1-acid glycoprotein concentrations. α1-Acid glycoprotein is an acute phase reactant whose concentration increases after surgery. Concentrations of α1-acid glycoprotein in infants are less in those undergoing elective rather than emergency surgery.91 This increased concentration of α1-acid glycoprotein increases the total plasma concentrations for low to intermediate extraction drugs, such as bupivacaine. The unbound concentration, however, will not change because the clearance of the unbound drug is affected only by the intrinsic metabolizing capacity of the liver (i.e., clearance).92,93
Plasma concentrations of lidocaine that depress the cardiovascular and respiratory systems in human neonates are about half those that cause similar toxicity in adults.94 In contrast, 2-day-old guinea pigs were less susceptible to the toxic effects of bupivacaine than 2-week or 2-month-old guinea pigs, even though the blood concentrations in the 2-day-olds were greater.75 Data regarding toxicity in infant versus adolescent versus adult rats for both bupivacaine and ropivacaine are similar.61 Young dogs, however, have a decreased threshold to both seizure and cardiac toxicity caused by excessive doses of bupivacaine.95 Because the responses to toxic concentrations of local anesthetics vary among species, it is difficult to know which animal model and thus which study results are most applicable to human neonates.96 No data exist in humans regarding age-dependent differences in the toxic threshold of bupivacaine at a given blood concentration. Seizures and cardiovascular collapse have been reported in human infants at the same blood concentrations of bupivacaine as in adults. Whereas data from some animal studies suggest that the greater volume of distribution of amides in younger children may protect against bupivacaine toxicity, retrospective analyses of large databases of infants who have received epidural infusions indicate that these findings may not be applicable to the human infant, particularly during continuous infusions or with repeated dosing. Current data on the pharmacokinetic and pharmacodynamic differences associated with early infancy suggest that caution should be exercised when using local anesthetics in infants. Several reports document that infants and children may develop systemic toxicity, including dysrhythmias, seizures, and cardiovascular depression from the accumulation of bupivacaine administered via epidural infusions.41,97–100 Meticulous attention must be paid to the total dose of local anesthetic administered, the rate of administration, the site of injection, and the use of vasoconstrictors to diminish the rate of uptake of the local anesthetic. This is particularly important when a continuous regional anesthetic technique is used postoperatively or when repeated doses of local anesthetic are administered during prolonged surgery.
We recommend that both the bolus and infusion doses of bupivacaine and lidocaine be reduced by approximately 30% for infants younger than 6 months of age to decrease the risk for toxicity. This would mean that the maximum rate of bupivacaine administration should not exceed 0.2 to 0.3 mg/kg/hr.42 Whereas these recommendations are particularly applicable to continuous infusions of bupivacaine for postoperative analgesia, the same caveats apply to large single injections, repeated injections, and continuous infusions of local anesthetics during prolonged surgeries.
The l-stereoisomer (or enantiomer) of racemic mixtures of local anesthetics is associated with less cardiac and CNS toxicity compared with the d-enantiomer in the mixture.78 Both ropivacaine and levobupivacaine, which are l-enantiomers, the latter the l-enantiomer of bupivacaine, induce less cardiac toxicity than the racemic mixture of bupivacaine in adults and experimental animals.79 This may be due in part, to the reduced affinity of the l-enantiomers for cardiac and CNS binding sites.60 The inclusion of levobupivacaine and ropivacaine into the clinical armamentarium may decrease the toxicity of local anesthetics.
Prevention of Toxicity
There are few data that correlate the anesthetic block, blood concentration of local anesthetic, and dose in infants and children. Most dosing guidelines have been extrapolated from studies in adults. Table 41-2 lists the maximum recommended doses of local anesthetics, as well as their approximate durations of action. To avoid overdose and the possibility of toxic effects, it is prudent to remain within these guidelines until studies in children clarify the pharmacokinetics and pharmacodynamics of local anesthetics for specific nerve blocks. As discussed earlier, all of these doses should be reduced by approximately 30% in infants younger than 6 months of age.
TABLE 41-2 Maximum Recommended Doses and Duration of Action of Commonly Used Local Anesthetics
Local Anesthetic | Maximum Dose (mg/kg)* | Duration of Action (minutes)† |
---|---|---|
Procaine | 10 | 60-90 |
2-Chloroprocaine | 20 | 30-60 |
Tetracaine | 1.5 | 180-600 |
Lidocaine | 7 | 90-200 |
Mepivacaine | 7 | 120-240 |
Bupivacaine | 2.5 | 180-600 |
Ropivacaine | 3 | 120-240 |
*These are maximum doses of local anesthetics. Doses of amides should be decreased by 30% in infants younger than 6 months of age. When lidocaine is being administered intravascularly (e.g., during intravenous regional anesthesia), the dose should be decreased to 3 to 5 mg/kg; there is no need to administer long-acting local anesthetic agents for intravenous regional anesthesia, and such a practice is potentially dangerous.
†Duration of action is dependent on concentration, total dose, site of administration, and the child’s age.
Toxic reactions from local anesthetics are a function of (1) the total dose administered, (2) the site of administration, (3) the rate of uptake, (4) pharmacologic alterations in toxic threshold, (5) the technique of administration, (6) the rate of degradation, metabolism, and excretion of local anesthetic, and (7) the acid-base status of the child.101–106 Thus recommendations (including those of the authors) of a specific dose limit for a given drug are both overly simplistic and potentially misleading.107 Because of the multiplicity of factors that drive the final concentration of unbound local anesthetic, and in the absence of definitive and comprehensive data, conservative dosing remains the most prudent course.
Site of Injection
Injection of local anesthetics into very vascular areas leads to greater blood concentrations than the same dose injected into less vascular areas. The order of uptake (i.e., maximum blood concentration) of local anesthetics (in order from greatest to least) with regional blocks in adults is (1) intercostal nerve blocks, (2) caudal blocks, (3) epidural blocks, and (4) brachial plexus and femoral-sciatic nerve blocks.108 An easy way to remember this is by the mnemonic ICE Block:
Studies are required to determine whether this order holds true for children. Blood concentrations of bupivacaine twice those measured in older children have been reported in children weighing less than 15 kg after ilioinguinal nerve block for herniorrhaphy using only 1.25 mg/kg of 0.5% bupivacaine without epinephrine, which is half the usual recommended maximal dose.36 However, the fascia iliaca block in older children produced blood concentrations of bupivacaine that were within the acceptable safe range.109 Local infiltration of the wound in herniorrhaphy has not been associated with increased blood concentrations of local anesthetics,110 but scalp infiltration during neurosurgery may produce relatively greater blood concentrations.111 As would be expected, spinal anesthesia results in very small blood concentrations, even in neonates.89
Rate of Uptake
The rate of uptake of a local anesthetic depends on the vascularity of the site of injection. Increased perfusion increases uptake, whereas decreased perfusion decreases uptake.112 The rate of uptake in children is usually more rapid than in adults. In general, the addition of a vasoconstrictor to the local anesthetic reduces the rate of uptake and prolongs the duration of the block. In adults, the dose of epinephrine is usually limited when used in conjunction with potent anesthetic agents because of the risk of inducing cardiac arrhythmias. If epinephrine is combined with the ester anesthetics, there is no increased risk of arrhythmias. For example, in adults anesthetized with halothane, the maximum recommended dose of epinephrine is 1.0 to 1.5 µg/kg. In children, however, larger doses of epinephrine may be safe.113–115 We have used as much as 10 µg/kg of epinephrine in children, with a maximum dose of 250 µg, during halothane anesthesia without evidence of ventricular irritability, and these doses are likely to be even more safe with currently used inhalational anesthetics, which do not sensitize the myocardium to the arrhythmogenic effects of epinephrine. An epinephrine concentration of 1 : 100,000 should not be exceeded, and 1 : 200,000 or less is generally used. A quick reference for converting local anesthetic concentrations and the amount of epinephrine in various dilutions is presented in Tables 41-3 and 41-4. Epinephrine is contraindicated in blocks in which vasoconstriction of an end-artery could lead to tissue necrosis, such as for digital and penile blocks, although this historical practice has recently been challenged, setting the stage for prospective studies.116–118
Epinephrine Dilution | µg/mL |
---|---|
1 : 100,000 | 10 |
1 : 200,000 | 5 |
1 : 400,000 | 2.5 |
1 : 800,000 | 1.25 |
TABLE 41-4 Local Anesthetic Concentration and Its Conversion to mg/mL
Concentration (percent) | mg/mL |
---|---|
3 | 30 |
2.5 | 25 |
2 | 20 |
1 | 10 |
0.5 | 5 |
0.25 | 2.5 |
0.125 | 1.25 |
Alteration in Toxic Threshold
Medications, such as diazepam or midazolam, that increase the seizure threshold (i.e., the threshold for CNS toxicity) can be valuable adjuncts to regional anesthesia. Premedication with diazepam (0.15 to 0.3 mg/kg) decreases a child’s anxiety but also offers some protection from the toxic CNS effects of a local anesthetic overdose.119 Although diazepam is no longer in common clinical use in children in the perioperative period, evidence in animal models and considerable clinical experience in humans suggests that midazolam is also effective in terminating seizure activity.120 Animal data, however, suggest that the concomitant use of diazepam and bupivacaine decreases the elimination of bupivacaine from serum and cardiac tissue in mice.121 This effect is not a result of changes in protein binding.122 It is not known if this is true for all benzodiazepines or if this is also the case in humans. Although premedication with a benzodiazepine prevents manifestations of CNS toxicity, the threshold for cardiovascular toxicity is unchanged.123 Thus after premedication with a benzodiazepine, cardiovascular collapse can occur without warning because the symptoms of CNS toxicity may be blunted. Because most regional anesthesia administrations that are performed in children are placed after induction of general anesthesia (with the exception of some blocks in former preterm infants), this may be a moot point in most circumstances.
Technique of Administration
Whenever regional anesthesia is performed, the operator must be prepared for an adverse reaction and resuscitation supplies, including drugs, suction, and airway equipment, must be immediately available. The needle or catheter must always be inspected for blood as soon as it is positioned, but before injecting the local anesthetic, to determine if the tip is within an artery or vein. It is preferable to observe the needle or catheter for passive blood flow rather than to actively aspirate for blood because the blood vessels, such as the epidural venous plexus, are thin walled and collapse readily when negative pressure is applied. As a result, the inability to aspirate blood is not absolute proof that the needle or catheter is not in a blood vessel. For this reason, a small volume of local anesthetic with a marker for intravascular injection, such as epinephrine in a concentration of 1 : 200,000, is administered first, while the ECG is observed for 30 to 60 seconds. Data from awake adults indicate that the heart rate will increase within 1 minute of intravascular administration.124 When the drugs are administered during general anesthesia, however, the efficacy of the test dose to detect an intravascular injection may be greatly reduced. Heart rate increases in only 73% of children after an IV injection of 0.5 µg/kg of epinephrine during halothane anesthesia, suggesting that this marker of an intravascular injection is not completely reliable.125 Administration of atropine several minutes before the test dose increased the rate of positive responders to 92%, suggesting that vagal tone and the anesthetic’s blunting of the sympathetic reflexes are responsible for the reduced sensitivity to the test dose. Test doses during isoflurane anesthesia appear to have the same limitations.126 With sevoflurane, positive results were obtained in 100% of children, if the threshold for a positive response was an increase in heart rate of 10 beats per minute and a dose in excess of 0.5 µg/kg of epinephrine was used; positive results were obtained in 85% if 0.25 µg/kg of epinephrine was used.127 In all children, a change in the T-wave amplitude was a reliable indicator of intravascular injection with both doses of epinephrine (see Fig. 41-1). All children in that study were pretreated with atropine. It is not known if increasing the dose of epinephrine to 1.0 µg/kg or increasing the concentration of epinephrine in the test dose solution to 1 : 100,000 during general anesthesia would increase the sensitivity of the heart rate response test without atropine. Systolic blood pressure increased by more than 10% within 60 seconds of the test dose injection, suggesting that an increase in blood pressure may be a more sensitive indicator of intravascular injection than heart rate during inhalation anesthesia. ST-segment and T-wave changes also appear to be sensitive indicators of intravascular injection of local anesthetic. Observation of the ECG yields a highly sensitive indicator of an intravascular injection of bupivacaine with epinephrine. These ECG changes were present in 97% of infants and children who received an IV dose of bupivacaine and epinephrine.81 These investigators did not confirm the efficacy of pretreatment with atropine on the heart rate. Isoproterenol (added to the local anesthetic rather than epinephrine), 0.075 to 0.1 µg/kg, increases the heart rate in 90% to 100% of children during halothane anesthesia.128,129 It also increases the heart rate in adults during isoflurane and sevoflurane anesthesia, but this has not been extensively studied in children.130–132 During total intravenous anesthesia (TIVA) with propofol and remifentanil, however, quite different results have been reported. A prospective study of children who received an IV injection of bupivacaine with epinephrine as a simulated positive test dose during TIVA, found that T-wave alterations were inconsistent and could not be relied on as an indicator of intravascular injection.107 Only blood pressure (particularly diastolic) was a consistent and reliable marker of intravascular injection, increasing more than 10% in all subjects during TIVA.
Even though no test dose regimen is completely infallible, it appears most prudent to use an epinephrine-containing test dose before administering the therapeutic dose of local anesthetic, particularly if the block is administered in an anatomic location near a blood vessel.127 The test dose should be repeated before subsequent bolus injections through a catheter. If the drug injection is visualized in real time using ultrasound, test dosing may be less critical, as the actual drug deposition can be visualized during the injection, although this, too, may not be infallible. If the child is receiving a general inhalation anesthetic, blood pressure and the ST-segment configuration, in addition to the heart rate, should be carefully and frequently observed after injection of the test dose.84 Pretreatment with atropine may increase the rate of detecting an unintended intravascular injection. In addition, the rate of injection may also be a factor in the development of toxicity. If injection is partially or completely intravascular, a slow injection may not exceed the toxic threshold, whereas a rapid injection could. Thus slow, incremental injection of the therapeutic blocking dose of local anesthetic (over several minutes), even though repeated injections within a brief period might also result in toxic reactions, may further increase the safety of regional blockade.
Treatment of Toxic Reactions
Treatment of toxic reactions to local anesthetic overdose requires knowledge of the signs and symptoms previously described. The signs of local-anesthetic toxicity, with the exception of the catastrophic cardiovascular events, are all masked by general anesthesia. Indeed, inhaled anesthetics may actually raise the threshold for seizures and thereby delay the detection of toxicity until cardiovascular collapse occurs. Even in the nonanesthetized child, the progression from prodromal signs to cardiovascular collapse may be very rapid and the initial resuscitative therapy in some cases may need to be directed at reestablishing circulation and normal cardiac rhythm, including the timely institution of chest compressions, while definitive treatments are being readied. As always, initial management should consist of establishing and maintaining a patent airway and providing supplemental oxygen. The timely administration of a CNS depressant that alters the seizure threshold may prevent seizures. Midazolam (0.05 to 0.2 mg/kg IV), thiopental (2 to 3 mg/kg IV), or propofol (1 to 3 mg/kg IV) effectively prevent or terminate seizure activity, however the latter two agents are also potent myocardial depressants and should be used with caution. If seizure activity is present and the airway is not secured, the use of succinylcholine or other relaxant may facilitate tracheal intubation but does not prevent seizure activity. It should be remembered, however, that the acute morbidity from seizures is the result of airway complications (hypoxia and aspiration) and that securing the airway takes precedence over the actual control of the electrical activity of the seizure. CNS excitability is exacerbated in the presence of hypercarbia; it is, therefore, important to mildly hyperventilate children who have seizures. None of these interventions should in any way supplant or delay the administration of lipid emulsion to directly treat the toxic levels of local anesthetic, and help should be sought to carry out these treatments simultaneously. Indeed, two case reports suggest that lipid emulsion may reverse the CNS symptoms of local-anesthetic toxicity in the absence of cardiovascular collapse, and might be a preferable first line therapy.133,134
Advances in the treatment of local-anesthetic toxicity have dramatically altered the therapeutic interventions that should be initiated in the event of cardiovascular collapse after a large intravascular injection of an amide local anesthetic. IV lipid emulsion has been shown to be effective for resuscitation of cardiac arrest caused by both bupivacaine and ropivacaine toxicity. In dogs, successful resuscitation after cardiac arrest and 10 minutes of external cardiac massage was demonstrated after lipid administration.135 Although 100% of the dogs that received an infusion of 20% lipid emulsion were successfully resuscitated, none of the controls that received a saline infusion survived. A clear relationship between the tissue concentration and the response has also been established.136 These animal studies have been corroborated by several anecdotal reports of rescue from cardiac arrest in humans that followed intravascular injections of all of the amide local anesthetics in common use.137–140 The mechanism of action of lipid emulsions in bupivacaine toxicity is not entirely understood, but studies in isolated rat heart preparations concluded that lipid treatment promotes the elution of bupivacaine from the myocardium and accelerates the recovery from bupivacaine-induced asystole.141 This “lipid sink” hypothesis suggests a novel mechanism of action as compared with more conventional antidysrhythmic drugs, and the treatment appears to be more effective as well. An inverse relationship between the concentration of lipid emulsion and the myocardial bupivacaine concentration (greater lipid concentrations were more effective at decreasing the bupivacaine concentration in the myocardium) suggests that this lipid sink hypothesis is correct.136 In support of this theory, in vitro evidence indicates that the lipid solubility of the local anesthetic determines, in part, its responsiveness to lipid resuscitation.142 Although long-chain lipids are recommended for resuscitation after the pH is normalized, recent evidence suggests that a lipid formulation that contains a mixture of medium- and long-chain lipids is superior to long-chain lipid to extract local anesthetic, and that the binding efficiency of the former is independent of blood pH.143
In a rat study of cardiac arrest, excessive epinephrine during the initial arrest phase may actually impair resuscitation with lipid emulsion and prevent a sustained response to treatment, and whereas the initial return to cardiac function may be quicker after epinephrine, sustained recovery was better after the lipid emulsion when compared with the high-dose (greater than 10 µg/kg) epinephrine group. This contrasts with a pig model of severe bupivacaine toxicity (50% reduction in arterial pressure) in which initial treatment with epinephrine more rapidly restored vital signs than did Intralipid (lipid emulsion) administration.144 The same investigators also demonstrated that during bupivacaine-induced cardiac arrest in a pig model, survival after epinephrine alone or the combination of epinephrine and Intralipid was greater than after Intralipid alone or in combination with vasopressin.144
The anecdotal human reports and experimental literature suggests that 1.5 mL/kg of 20% lipid emulsion should be administered over 1 minute and repeated every 3 to 5 minutes, up to a maximum of 3 mL/kg, followed by a maintenance infusion rate of 0.25 mL/kg/min until the circulation is restored.145 Several pediatric events report success with this intervention, with a range of doses similar to those reported in adults.138,146,147 Therefore, although the dose of lipids in children remains speculative and has not been subject to controlled investigation, the adult guidelines appear to be effective in children. There is a growing consensus that 20% lipid emulsions should be immediately available in any location where regional anesthesia is performed, to permit rapid treatment of cardiac toxicity. Although propofol is compounded in a lipid emulsion, it is not recommended as a substitute for Intralipid for resuscitation from local-anesthetic toxicity.
Because the initial stage of cardiovascular toxicity consists of peripheral vasodilation, supportive treatment should include IV fluid loading (10 to 20 mL/kg of isotonic crystalloid) and, if necessary, titration of a peripheral vasoconstrictor, such as phenylephrine (initial rate of 0.1 µg/kg/min), to maintain vascular tone and systemic blood pressure at acceptable limits. As toxicity progresses to cardiovascular collapse, profound decreases in myocardial contractility occur, followed by dysrhythmias. In dogs, echocardiography showed that decreased systolic function always preceded the development of dysrhythmias. Before lipid emulsion treatment was described, norepinephrine was shown to be more effective than epinephrine, dopamine, isoproterenol, or amrinone to treat bupivacaine cardiac toxicity in rats.148,149 In infants, a report suggested that phenytoin (5 mg/kg administered by a slow IV infusion) treated bupivacaine cardiac toxicity.150 Many toxic reactions are self-limited because the local anesthetic redistributes throughout the body and plasma concentrations rapidly decrease. Excretion of local anesthetic is hastened by hydration and alkalization of the urine by IV administration of sodium bicarbonate.151,152 Cardiopulmonary bypass has also been used to successfully resuscitate an adult with bupivacaine-induced cardiac toxicity.153 All current data, however, strongly suggest that, in addition to the standard CPR algorithm, lipid infusion is the most successful treatment, and immediate administration of this agent should be the next first line of therapy.
Hypersensitivity to Local Anesthetics
Hypersensitivity reactions to local anesthetics are rare.154–156 Ester local anesthetics are metabolized to p-aminobenzoic acid, which is usually responsible for allergic reactions in this group. However, these agents may cause allergic phenomena in children who are sensitive to sulfonamides, sulfites, or thiazide diuretics.157,158 Among the amide local anesthetics, only one case of a true allergic reaction has been documented. These drugs may contain the preservative, methylparaben, which can produce allergic reactions in those sensitive to p-aminobenzoic acid.158,159 When in doubt, local anesthetic allergy must be ruled out. Detailed protocols are described elsewhere.160
Equipment
Use of Ultrasound
Recently there has been great interest in the use of ultrasound-guided peripheral nerve blocks in children.161 The availability of high-resolution portable ultrasound machines has become increasingly commonplace and ultrasound-guided treatment will likely soon become the new standard of care for many peripheral nerve blocks. Although this requires sophisticated expensive equipment and the acquisition of new skills, it is likely to have a larger role in pediatric regional blockade because most blocks are performed while the child is anesthetized. Several manufacturers make ultrasound machines that are about the size of a laptop computer and have been designed for ease of use by the anesthesiologist. The cost-effectiveness of acquiring these devices is justified because they serve a dual purpose for placing invasive central lines. Direct visualization of the nerve may facilitate correct placement of the local anesthetic and may also help reduce the total dose of local anesthetic needed for successful blockade. It is imperative to use an ultrasound machine that is capable of scanning superficially because most of the nerves in children are usually less than a few millimeters from the skin. A more in-depth discussion of ultrasound guidance for peripheral nerve blocks can be found in Chapter 42. Because ultrasound might still not be available to every practitioner, a complete discussion of landmark and nerve-stimulator guided peripheral blockade is included in this chapter.
Use of a Nerve Stimulator
The use of a peripheral nerve stimulator is an alternative safe and effective method to locate the nerve to be blocked. Despite the increasing use of ultrasound guidance for placement of peripheral nerve blocks, some anesthesiologists still use nerve stimulation to verify the position of the tip of the needle when ultrasound is not available, or in combination with ultrasound, particularly when imaging is suboptimal. A nerve stimulator is not a substitute for anatomic knowledge, but is a useful adjunct that allows the performance of the block in an unconscious or uncooperative heavily sedated child. It avoids the need to seek sensory paresthesias or to rely purely on anatomic landmarks. The tiny amount of current flowing from the uninsulated needle tip stimulates the nerve and produces a motor response when the needle is in close proximity to the nerve. The nerve stimulator is attached to the child as shown in E-Figure 41-2. The cathode (negative pole) cable is attached to the low output terminal of the nerve stimulator at one end and to the proximal (uninsulated) shaft of a Teflon-insulated needle via a sterile alligator clip, or to the plug-in lead of a specially designed block needle at the other end (the child). The anode (positive lead) cable is attached to the high-output terminal of the stimulator at the one end and to the child, distant to the block site, via an ECG electrode at the other end.162,163 The needle is advanced in the appropriate anatomic direction, and when it appears to be in the correct position, the nerve stimulator is adjusted to approximately 0.5 mA with repetitive single pulse output at 1-second intervals. Local muscle contraction should be minimal at this setting, although direct muscle stimulation can occur and must be distinguished from neural stimulation. The area innervated by the nerves to be blocked is observed for the appropriate muscle contractions. As the uninsulated needle tip approaches the nerve, the muscle contractions will increase in intensity and become less strong as the needle tip moves away from the nerve. One should be able to decrease the current to approximately 0.2 mA with continued elicitation of easily perceptible muscle contraction to be sure that the needle tip is correctly positioned. It should be noted that the injection of even a very small volume of local anesthetic will ablate or dramatically attenuate responses produced by the low current of the nerve stimulator, so the needle position should be optimized before injection (Video: Poppliteal Fossa Block of Sciatic Nerve with Nerve Stimulator). The responses to stimulation of the radial, median, ulnar, and musculocutaneous nerves are shown in Figure 41-2.
Nerve stimulation has been used to verify the dermatomal level to which the tip of an insulated epidural catheter has been threaded. A low-dose current applied to a fine wire within the lumen of the epidural catheter stimulates motor paresthesias in the muscles of the abdomen and thorax and the most cephalad responses represent the dermatomal level corresponding to the tip of the catheter.164,165
Specific Procedures
Central Neuraxial Blockade
Anatomic and Physiologic Considerations
Several anatomic and physiologic differences between adults and children affect the performance of regional anesthetic techniques. The conus medullaris (the terminus of the spinal cord) in neonates and infants is located at the L3 vertebral level, which is more caudal than in adults. It does not reach the adult level at L1 until approximately 1 year of age (E-Fig. 41-3) owing to the difference in the rates of growth between the spinal cord and the bony vertebral column. Thus lumbar puncture for subarachnoid block in neonates and infants should be performed at the L4-5 or L5-S1 interspace to avoid needle injury to the spinal cord. The vertebral laminae are poorly calcified at this age, so a midline approach is preferable to a paramedian one in which the needle is “walked off” the laminae. Another anatomic difference is noted in the sacrum. In neonates, the sacrum is narrower and flatter than in adults (see E-Fig. 41-3). The approach to the subarachnoid space from the caudal canal is much more direct in neonates than in adults, making dural puncture more likely, so the needle must not be advanced deeply in neonates.166 The presence of a deep sacral dimple may be associated with spina bifida occulta, greatly increasing the probability of dural puncture. Thus a caudal block may be contraindicated in these children.
The distance from the skin to the subarachnoid space, which is very small in neonates (approximately 1.4 cm) and increases progressively with age (Fig. 41-3).167 The ligamenta flava are much thinner and less dense in infants and children than in adults, which makes the engagement of the epidural needle more difficult to detect and unintended dural puncture during epidural catheter placement a greater risk for the infrequent operator. Cerebrospinal fluid (CSF) volume as a percentage of body weight is greater in infants and young children than in adults (E-Fig. 41-4), although these studies are limited.168–172 This finding may account in part for the comparatively larger doses of local anesthetics required for surgical anesthesia with subarachnoid block in infants and young children. The CSF turnover rate is also greater in infants and children, accounting in part for the much briefer duration of subarachnoid block with any given agent compared with adults. These anatomic differences necessitate meticulous attention to detail to achieve successful and uncomplicated spinal or epidural anesthesia.
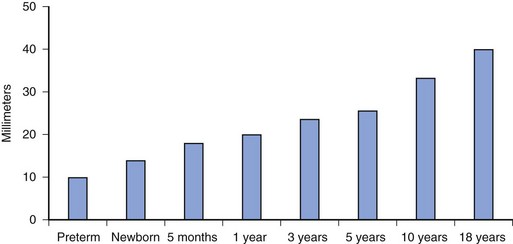
FIGURE 41-3 Distance from the skin to the subarachnoid space as a function of age.
(Data from Bonadio WA, Smith DS, Metrou M, et al. Estimating lumbar puncture depth in children. N Engl J Med 1988;319:952-3; Kosaka Y, Sato K, Kawaguchi R. [Distance from the skin to the epidural space in children.] Masui 1974;23:874-5; Lau HP. [The distance from the skin to the epidural space in a Chinese patient population.] Ma Tsui Hsueh Tsa Chi 1989;27:261-4.)