Pulmonary Function Testing
A The gas in the respiratory system is divided into four lung volumes and four lung capacities.
a. Residual volume (RV): Volume of gas remaining in the lung after a maximal exhalation.
b. Expiratory reserve volume (ERV): Volume of gas that can be exhaled after a normal exhalation.
c. Tidal volume (VT or TV): Volume of gas inspired during a normal inspiration.
d. Inspiratory reserve volume (IRV): Volume of gas that can be inspired after a normal inspiration.
2. Lung capacities are composed of two or more lung volumes.
a. Total lung capacity (TLC): Volume of gas contained in the lung at maximum inspiration (RV + ERV + VT + IRV).
b. Inspiratory capacity (IC): Maximum volume of gas that can be inhaled after a normal exhalation (VT + IRV).
c. Vital capacity (VC): Maximum volume of gas that can be exhaled after a maximal inspiration (ERV + VT + IRV).
d. Functional residual capacity (FRC): Volume of gas that remains in the lung after a normal exhalation (RV + ERV).
B Normal lung volumes and capacities for a healthy young male with 1.7 m2 body surface area (165 lb, 6 ft tall, 25 years old) are:
2. VC: 4.80 L, approximately 80% of TLC
3. IC: 3.60 L, approximately 60% of TLC
4. FRC: 2.40 L, approximately 40% of TLC
5. RV: 1.20 L, approximately 20% of TLC
6. ERV: 1.20 L, approximately 20% of TLC
7. VT: 0.5 L, approximately 6.3 ml/kg of ideal body weight (10% of TLC)
C All lung volumes and capacities depicted in Figure 19-1 can be measured by direct spirometry except:
II Spirometry Refers to Simple, Widely Used Tests That Measure VC and Its Subdivisions (Adapted from AARC Clinical Practice Guidelines)
A Slow VC measurement (see Figure 19-1)
1. The subject breathes normally for several breaths, followed by a maximal inspiration and a maximal exhalation.
2. The resting expiratory level must be stable to obtain valid test results.
3. Large TVs or an irregular pattern will result in inaccurate measurements of IC and ERV. These values are used to calculate RV and TLC.
B Forced vital capacity (FVC) and its subdivisions are the most widely used PFTs. The graphic representation of this simple maneuver provides information for the determination of many useful variables or calculations.
1. The subject breathes normally for several breaths, then inspires maximally and exhales as forcefully and fully as possible.
2. FVC and VC should be within 200 ml of each other in healthy patients.
3. Decreased FVC is a nonspecific finding; any disorder that affects the elasticity of the lungs can decrease FVC.
a. With severe airway obstruction, such as emphysema, or small airway collapse, gas is trapped in the alveoli, reducing FVC.
b. Restrictive lung diseases, such as pulmonary fibrosis or pneumonia, can reduce FVC.
4. FEV1 is the forced expiratory volume that can be exhaled in 1 second.
a. FEVT for other time intervals (T) can easily be determined from the FVC curve.
b. FEV1 is reported as volume, although it is essentially a measurement of flow (volume/time).
c. A decrease in FEV1 indicates obstructive changes in small airways.
d. Large airway obstruction from a tumor or a foreign body causes a decrease in FEV1, which continues through the entire FVC.
5. FEVT% is the ratio of the FEV for any given time interval (T) to the FVC (Figure 19-2).
a. The percentage of the FVC that can be expired in 1 second, or FEV1%, is calculated below:
< ?xml:namespace prefix = "mml" />

b. FEV1% tends to decrease with age, as the elasticity of the lung decreases.
c. Normal values for young adults
d. A decrease in FEV1% is the most important indicator of obstructive disease.
6. FEF25%-75% is the average expiratory flow rate of the middle 50% of the FVC (Figure 19-3).
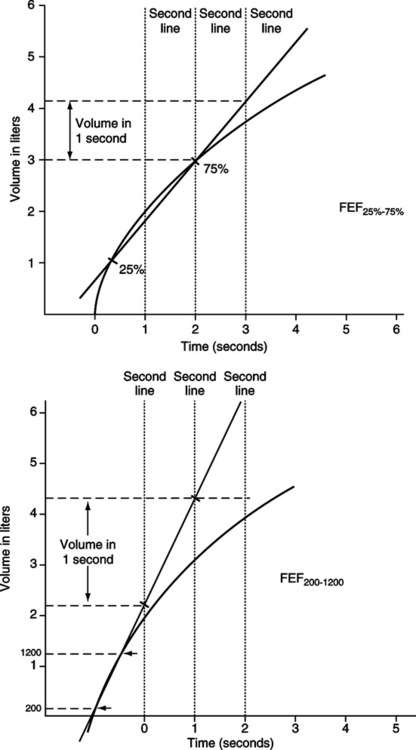
a. Reflects flow from medium and small airways.
b. Smoking causes changes in flow from medium airways before there is evidence of obstruction in small airways.
7. FEF200-1200 is the average expiratory flow rate between the first 200 ml and 1200 ml of exhaled volume during an FVC maneuver (see Figure 19-3).
8. The FEF200-1200 value in L/sec is multiplied by 60 to convert it to L/min.
9. Diagnosis of obstructive disease using PFT results
a. FEF25%-75% for young, healthy adults is 4 to 5 L/sec, but results vary considerably.
b. FVC, FEV1, and FEV1% should be considered together to determine the presence and severity of an obstructive disorder.
c. Other measurements, such as FEF25%-75%, are useful to confirm the diagnosis but should not be used as a primary indicator.
C Maximum voluntary ventilation (MVV)
1. Calculates the maximum volume of gas that a patient can ventilate in 1 minute.
2. The patient is directed to breathe rapidly and deeply for 12 to 15 seconds.
3. The total volume inspired or expired during the testing interval is measured.
4. The volume is extrapolated to 1 minute. For example, if the patient breathed 40 L in 15 seconds:

5. MVV must be corrected to body temperature and pressure saturated (BTPS).
6. Normal MVV for young healthy men is 150 to 200 L/min; slightly lower values are normal for women.
7. Decreased values indicate increased airway resistance or obstruction, decreased lung or thoracic compliance, or ventilatory muscle weakness.
D Peak expiratory flow (PEF) rate
1. The highest expiratory flow rate occurs during the early part of exhalation.
2. When PEF is reported with other spirometry values, it is expressed as L/sec. When it is performed alone using a peak flowmeter, it is expressed as L/min.
3. Peak flows normally are equal to 400 to 600 L/min for young, healthy men and 300 to 500 L/min for young, healthy women.
4. Decreased peak flows indicate airway obstruction in large airways.
E Flow-volume (F-V) curves (Figure 19-4)
1. An F-V loop graphically depicts flow rate plotted against volume change during an FVC maneuver.
2. To perform this maneuver, the patient maximally inspires, followed by a single forced exhaled vital capacity (FEVC) and forced inspired vital capacity (FIVC).
3. A tidal F-V loop superimposed on a VC loop allows easy determination of many flow and volume variables.
4. Computer-generated graphics allow easy manipulation and comparison of F-V loops.
a. Most computerized spirometers indicate time increments on the curve so that FEV1 can be read from the loop.
b. Successive F-V loops can be superimposed on each other to demonstrate reproducibility or response to bronchodilators (Figure 19-5).
c. Superimposed loops may show decreasing flows with successive efforts. FVC maneuvers can induce bronchospasm in patients with reactive airways.
d. Some pulmonary disorders can be identified from specific, reproducible changes in the shape of the F-V loop (Figure 19-6).
e. Normal or predicted loops can be easily compared with suspected abnormal loops.
III Useful Guidelines for Spirometry Equipment and for Evaluating and Reporting Test Results
A The American Thoracic Society sets standards for spirometry equipment.
1. Volume measurement range of 0.5 to 8 L.
2. Flow measuring range of 0 to 14 L/sec.
3. Capable of accumulating volume for 15 seconds.
4. The maximum acceptable error for volume measurements is ±3% or ±0.050 L, whichever is greater.
5. Resistance and backpressure must be <1.5 cm H2O/L/sec over the standard flow range.
B Criteria to evaluate accuracy of spirometry results
1. Spirometry measurements depend on patient effort. Practitioners should be careful to ensure reproducibility before test values are reported.
2. Evaluation of the volume-time tracing
a. The curve should be smooth and show at least 6 seconds of forced effort.
b. A plateau lasting at least 1 second should be evident. There should be <30 ml of volume change during the plateau.
c. The test can be acceptable when the FVC maneuver is stopped at <6 seconds for a clinical reason, such as excessive coughing or dizziness.
d. Patients with severe airway obstruction may continue to exhale at low flow rates for >15 seconds. Stopping the maneuver at 15 seconds will not significantly change the results.
3. Evaluation of the start-of-test
a. The beginning of the maneuver should be abrupt and distinct.
b. Time zero should be calculated by back extrapolation of each FVC curve (i.e., a straight line drawn through the steepest part of the curve is extended until it intersects the x-axis. The point of intersection is time zero.)
c. The volume exhaled at the back-extrapolated time zero should be <5% of the FVC or <0.150 L, whichever is greater (Figure 19-7).
a. A minimum of three acceptable maneuvers should be recorded.
b. A maximum of eight attempts should be performed to show reproducible efforts.
c. If two acceptable maneuvers cannot be recorded after eight attempts, the test should not be reported.
d. The largest and second largest FVC and FEV1 measurements from acceptable curves should be within 200 ml. A maximum of 5% difference has also been used as the reproducibility criterion.
C Reporting of spirometry results
1. Lung volumes are always corrected to BTPS.
2. The largest FVC and FEV1 from acceptable curves are reported even if the two values are from different curves.
3. Flows that depend on the FVC are taken from the single best maneuver.
4. The maneuver with the largest sum of FVC and FEV1 is considered the best effort.
IV Tests Used to Measure Lung Volumes and Gas Distribution (Adapted from the AARC Clinical Practice Guidelines)
A Volumes of gas and lung capacities that can be exhaled from the lung (i.e., VT, VC, IRV, ERV, and IC) can be measured directly with spirometry.
B Lung volumes and capacities that cannot be exhaled (i.e., FRC, TLC, and RV) are determined using indirect methods.
C The FRC value is measured using indirect methods and used to calculate TLC and RV.
D Computed tomography (CT) scans and magnetic resonance imaging (MRI) provide a direct view of gas distribution in the lungs and can be used to determine FRC, TLC, and RV.
E The multiple-breath nitrogen washout study uses an open circuit method to determine FRC (Figure 19-8).
1. Because nitrogen makes up approximately 80% of FRC when the subject is breathing room air, the volume of nitrogen in the total exhaled gas will equal approximately 80% of the FRC.
2. The patient breathes 100% oxygen through a valve-mouthpiece system for 7 minutes or until the alveolar concentration of nitrogen decreases to approximately 1%.
3. Measurements are started at end expiration.
4. A rapid response nitrogen (N2) analyzer and a spirometer measure breath-by-breath N2 concentration and exhaled volume. Values are summed to provide the total volume of N2 washed out.
5. Corrections must be made for the 30 to 40 ml of N2 that are washed out of the blood and tissue during each minute of the test.
6. FRC is calculated using the equations below.

7. ERV obtained from a slow VC maneuver is used to calculate RV and TLC
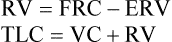