Pulmonary Function Measurements
After reading this chapter, you will be able to:
• Describe how the normal range of human pulmonary function values are obtained
• Explain why dilution or washout measurements of functional residual capacity (FRC) in severe obstructive airways disease generally differ from values obtained by the plethysmographic method
• Explain why both restrictive and obstructive disease mechanisms reduce the vital capacity
• Explain why restrictive and obstructive diseases affect the FRC, the residual volume, and the work of breathing differently
• Differentiate between purely obstructive and restrictive patterns of pulmonary function tests
• Explain why large and small airways resistance affects certain spirometric tests in different ways
• Explain why the results of some spirometric tests can be improved with greater effort, but the results of others are independent of effort
• Describe the theory behind the test that detects grossly uneven ventilation of the lungs
• Explain why the relationship between maximum voluntary ventilation and ventilation attained during maximal exercise is different for individuals with severe obstructive pulmonary disease compared to healthy people
• Explain the basic theory behind tests that are especially sensitive to increases in small airways resistance
Static Lung Volumes
Static lung volumes and their spirometric measurement are discussed in Chapter 3. Normal values and interrelationships among volumes and capacities are illustrated in Figure 5-1. Anthropometric differences (differences in body type and size) affect normal values, creating ranges in which normal function is presumed. Normal ranges are derived from a statistical analysis of large populations of “normal” people (i.e., people who are healthy, have no history of lung disease, and have minimal exposure to risk factors such as smoking and environmental pollution). Physical characteristics that influence pulmonary function most are age, gender, height, ethnic origin, and body size or surface area.1 Normal values are predicted for an individual based on these physical characteristics. Function is generally classified as normal if values are within 20% of the predicted value (i.e., 80% to 120% of the predicted value). Table 5-1 displays the relationship between the percentage of the predicted normal value and the degree of functional impairment.
TABLE 5-1
Degree of Impairment | Percentage of Predicted | SD Above and Below Mean |
Normal | 80-120 | ±1 |
Mild | 65-79 | ±1-2 |
Moderate | 50-64 | ±2-3 |
Severe | 35-49 | > ±3 |
Extremely severe | <35 | — |
Modified from Wilkins RL, Stoller JK, Scanlan CL: Egan’s fundamentals of respiratory care, ed 8, St Louis, 2003, Mosby.
Theoretical Basis for Measurement
Because residual volume (RV) cannot be exhaled, it cannot be measured via direct spirometry. Therefore, no capacity containing RV can be directly measured. RV and capacities containing it are measured indirectly via one of the following methods: helium dilution, nitrogen washout, or body plethysmography. The dilution and washout techniques measure gas in the lung only if the gas communicates with unobstructed airways. These tests are started at the end of a normal expiration (i.e., the functional residual capacity [FRC] level). Washout and dilution tests measure the FRC, which is the logical starting point because it is highly reproducible and its achievement requires no patient effort—only ventilatory muscle relaxation. As shown in Figure 5-1, after FRC is determined, spirometric measurement of the expiratory reserve volume (ERV) allows the RV to be calculated (RV = FRC − ERV). Likewise, spirometric measurement of inspiratory capacity (IC) allows total lung capacity (TLC) to be calculated (FRC + IC = TLC). Alternatively, if RV is measured, TLC can be calculated as follows: RV + vital capacity (VC) = TLC.
Helium Dilution Method
The helium dilution method requires the person to rebreathe a helium gas mixture through a closed circuit from a spirometer of known volume (Figure 5-2). Helium is a foreign gas to the lung and is inert and insoluble in the blood. Therefore, the blood does not absorb helium during the test. The lung contains no helium initially (Figure 5-2, A), but rebreathing causes lung and spirometric gases to mix (Figure 5-2, B). A helium analyzer in the circuit continuously monitors helium concentration. As rebreathing progresses, lung gases dilute the helium in the spirometer. Consequently, helium concentration decreases in the spirometer and increases in the lung until equilibrium is reached (Figure 5-2, C). During the rebreathing process, the spirometric volume is kept constant through the addition of oxygen to the circuit to replace oxygen taken up by the blood. Likewise, a chemical in the circuit absorbs carbon dioxide entering the lung from the blood.
The principle of the closed-circuit helium dilution method is that the amount of helium in the lung spirometric system is the same at the end of the test as at the beginning. For example, after it is charged with helium, the spirometer’s volume may be 3000 mL and the helium concentration 10% (see Figure 5-2, A). In this example, the amount of helium present at the beginning of the test is 10% of 3000, or 300 mL (0.10 × 3000 = 300 mL). Because the lung contains no helium initially, 300 mL of helium must be present in the lung spirometer system at the end of the test after helium equilibrium is achieved. Consider an example in which the helium concentration at equilibrium is 5% (see Figure 5-2, C). The lung volume diluting the spirometer’s helium mixture is the FRC if the first inspiration of the helium mixture started from the resting FRC level. In principle, the FRC is calculated as follows:2

With the values from the previous example, the following can be calculated:





Nitrogen Washout Method

In the open-circuit nitrogen washout procedure (Figure 5-3), the person breathes through a one-way valve, inspiring 100% oxygen and expiring into a large gas-collecting spirometer previously flushed with 100% oxygen. Each inspiration is nitrogen-free, and each expiration eliminates some of the nitrogen in the lung. Normally, less than 7 minutes is required to eliminate most of the nitrogen in the lung, but patients with poorly ventilated areas may require longer washout times. The test usually ends when the expired nitrogen is less than 2%. The amount of nitrogen exhaled is calculated by analyzing the spirometer’s nitrogen concentration (FN2) and multiplying it by the Vs (Nitrogen [mL] = FN2 × Vs). If 35 L (35,000 mL) of expired gas is collected during the test and its nitrogen content is 6%, the amount of nitrogen exhaled is calculated as follows: 35,000 × 0.06 = 2100 mL. As the following indicates, 2100 mL is 80% of the lung volume:

The measured FRC must be corrected to reflect the volume it occupies under BTPS conditions.
Body Plethysmographic Method
The body plethysmograph is an airtight chamber, or “body box,” in which the patient sits while breathing through a mouthpiece (Figure 5-4). Sensitive pressure transducers measure mouthpiece and box pressures. Box calibration is carried out with an empty, sealed box; a known volume of gas is injected into the box with a large calibration syringe, and the change in box pressure is noted. This calibration procedure allows subsequent box pressure changes to be converted to volume changes.
At the end of expiration when gas flow ceases, mouthpiece pressure equals alveolar pressure. The lung volume at this point (the FRC) is unknown. An electrically controlled shutter occludes the mouthpiece precisely at the end expiratory point. The subsequent inspiratory effort cannot move gas. However, the respiratory muscles enlarge the thorax, decompressing the gas in the lung, decreasing its pressure (see Figure 5-4). In the absence of gas flow, intrapulmonary pressure equals mouthpiece pressure. At the same time, the enlarging thorax compresses air inside the box around the patient, increasing the box pressure. The change in box pressure is converted to the change in volume from the initial calibration procedure. The variables in Boyle’s law are assigned as follows: P1 equals initial mouth pressure (atmospheric pressure), V1 equals FRC (unknown), P2 equals final mouth pressure (measured during inspiratory effort through the occluded mouthpiece), and V2 equals FRC plus the change in lung volume (ΔV). The ΔV is known. In principle, the FRC is calculated as follows:
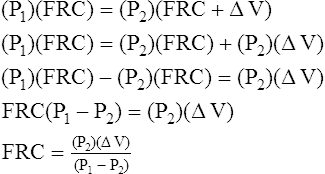
Significance of Changes in Functional Residual Capacity and Residual Volume
Physical factors that change FRC and RV are summarized in Box 5-1. Changes in lung recoil affect FRC because FRC is determined by the balance point between lung and thoracic recoil forces. An abnormally increased FRC represents hyperinflation, which may be caused by a loss of elastic recoil or partial airway obstruction. Partial airway obstruction caused by bronchospasm is generally reversed by bronchodilator drugs, and the associated increase in FRC is reversible. Increased FRC caused by a permanent loss of elastic recoil is irreversible. In severe emphysema, this loss of lung elasticity is associated with passive airway compression and collapse during expiration, causing air trapping. Bronchodilator drugs are not useful in these circumstances. (See the discussion on air trapping and auto-PEEP in Chapter 3).