CHAPTER 36 PULMONARY CONTUSION AND FLAIL CHEST
Pulmonary contusion and flail chest are the two most common anatomic complications of major blunt chest trauma. Each will directly alter pulmonary physiology in a specific and unique fashion, and thus contribute to pulmonary dysfunction and failure after trauma. Pulmonary contusion was probably first described by Morgagni in the 18th century, but Laurent’s description in the Lancet in 18831 appears to be the first to recognize the possibility that plasticity of the chest wall, most notably in the young, can allow injury to the underlying lungs without disruption of the bony thorax. Conversely, flail chest is predominantly a disease of the elderly, with most patients being in the sixth decade of life and beyond and older patients having the worst outcomes.2–4 Pulmonary contusion and flail chest commonly coexist but their degree of association changes and they may exist entirely separately under specific circumstances in specific patient groups. Yet because of their close association, their effects on pulmonary pathophysiology are often confused. Such confusion can lead to misapplication of studies aimed at one entity or the other and eventually to inappropriate treatment.
INCIDENCE
Pulmonary hemorrhage and contusion were noted to be common at autopsy of patients dying from battlefield and blast injuries during World War I.5,6 Similar findings were noted in World War II,7,8 and the term “pulmonary concussion” appears to have been coined by Hadfield describing civilian injuries from bomb blasts sustained during the Battle of Britain.9 Reports in the 1960s first noted that pulmonary contusions occurred frequently after civilian motor vehicular trauma and were seen in up to 10% of thoracic injuries.10
Currently, the incidence of “pulmonary contusion” varies markedly depending on how aggressively it is sought and diagnosed. In some large series, about 15% of major blunt injuries11 are found to have a pulmonary contusion. Using closer computed tomography (CT) evaluation, up to 25% of patients with chest trauma may be noted to have some form of contusion.12 Yet a 10-year registry review of approximately 20,000 blunt trauma patients seen at the New Jersey State Trauma Center in Newark performed in preparation for this review showed that only 2.6% of all patients arriving at our Level I trauma center were diagnosed as having a pulmonary contusion.
So the “denominator” patient population examined will clearly affect the disease incidence reported in administrative databases, as will the tendency to identify and report less severe injuries. Yet our experience over the last several years indeed shows that the diagnosis of pulmonary contusion is increasing alongside our increased use of CT diagnosis for chest trauma (Figure 1).
The relative frequency of flail chest as compared with pulmonary contusion will also vary depending upon the denominator population. Pediatric reviews find that the majority of major thoracic trauma presents with pulmonary contusions whereas flail chest is very rare, even where multiple fractures exist.13,14 In contrast, in a large contemporary descriptive series examining adult blunt chest trauma, flail chest was diagnosed in about half of all patients with significant pulmonary contusions.11 Moreover, the diagnosis of flail chest is often missed or delayed in sicker patients that require mechanical ventilation.4 This results from the synchronous expansion of the lungs and “splinting” of the chest wall by positive intrathoracic pressure. Thus, it is clear that the proportions change and flail chest becomes increasingly common with advancing age and brittleness of the thoracic cage. Consequently, the frail elderly frequently sustain a flail chest with relatively minor chest trauma and little or no pulmonary contusion. Similarly, flail chest has been reported in newborns with osteogenesis imperfecta.15
Physical Mechanisms of Injury
The overwhelming majority of significant blunt chest trauma in civilian life occurs as a result of motor vehicle crashes and motor vehicle versus pedestrian injuries. Falls are another common cause of pulmonary contusion and flail chest. Thoracic compression injuries are not as common as vehicular trauma and falls, and although they may produce similar syndromes, the slower speed of impact makes contusion less likely than flail chest. Rather, these patients may manifest traumatic asphyxia. In military practice, blast injuries from high explosives can occur both in air and underwater. These produce specific and recognizable diffuse contusion patterns resulting from the concentration of energy at interfaces between denser tissues and tissues that contain gas, like the lung and bowel. Although these injuries have been rare in civilian life for the last 60 years, the advent of international terrorism as a mode of political action within the last 10 years has led to a resurgence of such injuries in civilian life—first in the Middle East, and now in the West.16
All blunt injuries result from the physical transfer of energy to the patient, but because of the rigidity of the bony thorax, all pulmonary contusions and most flail chest injuries are high-energy injuries, with the primary exception being occasional chest wall injuries in the frail elderly. Thus, they are seen primarily in motor vehicular trauma, perhaps most classically where unrestrained drivers strike the steering column. Pedestrian trauma and falls from a distance are frequent causes. Interpersonal violence leading to blows with blunt objects or kicking are occasional causes of pulmonary contusion. Flail chest however, is rare in our experience, first because assaults are most common in young adults and second because biomechanically they are unlikely to result in segmental injuries of multiple contiguous ribs. The physician should also bear in mind that rib fractures in infants and small children occur most commonly as a result of child abuse, and that any rib fracture in a child is a marker for severe trauma.17
The transfer of energy typically leads directly to hemorrhage into the lung. Pulmonary lacerations are uncommon but can occur (Figure 2) and in our experience are seen with increasing frequency when routine CT imaging is used. On rare occasions, tangential gunshot injuries will cause contusions of the underlying pulmonary parenchyma without actually entering and lacerating the lung. These injuries are usually very limited in their extent and cause little or no physiologic effects. Another potential mechanism of pulmonary dysfunction after trauma is the activation of pulmonary vascular endothelium by percussive cellular deformation per se. This phenomenon is much better documented in cerebrovascular endothelial beds,18,19 but it is likely to exist in the pulmonary bed as well (see Figure 2).
Mechanisms of Physiologic Injury
Studies done toward the end of World War I suggested that blast injury predominantly resulted in pulmonary hemorrhage,5 and it was felt that pulmonary failure reflected the effects of blood filling the air spaces. Whereas this effect undoubtedly contributes to the increased pulmonary shunting (Qs/Qt) seen after injury, many other pathophysiologic processes are at work. There is now little doubt that the majority of pulmonary dysfunction seen after chest trauma results from secondary injury processes rather than direct injury to the lung. It is most convenient to divide the various pathophysiologic influences on pulmonary function into those that result in increased Qs/Qt and hypoxemia, and those that alter the work of breathing and can lead to ventilatory failure. Either can result from chest wall or parenchymal pathology. It is important to note that associated intrapleural collections of air and blood may also impact mechanical chest wall function and pulmonary aeration as well as systemic hemodynamic performance, although these considerations are outside the scope of this review.
Shunting and Hypoxemia
Systemic shock and ischemia/reperfusion (I/R) are well-known activators of immune system attacks on the lung, causing shunting and hypoxia. This is perhaps most clearly evident in lung transplantation,20 but is also seen in systemic I/R21 as well as intestinal I/R.22 All will activate the innate immune system and cause systemic inflammatory response syndrome (SIRS), which contributes to acute lung injury (ALI) and pulmonary dysfunction after chest trauma. Inadequately treated pain resulting from chest wall injury and splinting is a common cause of splinting and hypoventilation. The resultant atelectasis is a common cause of increased Qs/Qt and hypoxemia after trauma. The use of mechanical ventilation, although necessary, can result in ventilator-induced lung injury (VILI) through a number of mechanisms.23 Immunologic injury can be induced by leukocytes in the presence activating cytokines, resulting in increased lung water and decreased diffusion capacity of the lung (DL). Thus, secondary immune attack on the “primed” lung can be initiated by pneumonia, shock, injudicious ventilation strategies, or the release of cytokines into the circulation, as may happen in long-bone fixation.24,25
Increased Work of Breathing and Ventilatory Failure
In the presence of a flail chest, CO2 retention has commonly been attributed to the pendelluft phenomenon, where to-and-fro flow of gas has been postulated to exist between the two hemithoraces in the presence of a unilateral flail segment. This concept is intuitively appealing, and the re-breathing of airway gas does create a pathologic dead space. Yet direct application of this concept to clinical chest injury is probably simplistic. In practice, elevated shunt fractions and hypoxemia are more common in flail chest and in trauma in general than is hypercarbia. Moreover, pendelluft occurs in acute lung injury even without chest wall instability. This results from the heterogeneous viscoelastic properties of the injured lung itself, which leads to gas movements between lung segments of differing compliance.26 Clearly though, flail segments do make ventilation both painful and increasingly inefficient.
Inflammatory Lung Injury
Deteriorating pulmonary function after chest trauma is commonly related to systemic inflammation after injury. Acute lung injury (ALI) and adult respiratory distress syndrome (ARDS) are terms widely used to reflect the increasing severity of secondary lung injury after trauma. Such injury is widely believed to result from polymorphonuclear neutrophil (PMN)–endothelial cell (EC) interactions that injure pulmonary capillary endothelial membranes, causing interstitial and alveolar edema, and resulting in diminished compliance and gas diffusion. ALI/ARDS are usually defined as a diagnosis of exclusion where hypoxemia exists in the absence of other discrete causes of pulmonary failure such as pneumonia or congestive heart failure. In fact, ALI/ARDS probably exists in all major chest trauma to some extent. Although management of ALI/ARDS is to date supportive, an understanding of the pathogenesis is important because the lung should be understood to be “primed” for secondary insults after chest trauma and at risk for marked deterioration in the event of secondary insults like shock and sepsis. There is increased risk of pneumonia after chest trauma, and pneumonia, of course, can act both as a primary cause of pulmonary dysfunction and as a trigger for “second-hit” organ failure. A special problem is that chest trauma is often accompanied by long-bone fractures and patients with chest injuries are clearly at special risk for pulmonary deterioration after fracture fixation.27 Fractures are reservoirs for inflammatory mediators in the early post-injury period which can be mobilized to the bloodstream by operation and potentially contribute to ALI/ARDS.24,26,28,29 Prospective studies will be needed to determine whether orthopedic management of these patients should be tailored to the protection of lung function.
Extravascular Lung Water
Before the routine clinical use of pulmonary artery (PA) catheters, it was widely believed that fluid overload and subsequent increases in extravascular lung water were the primary cause of pulmonary dysfunction after trauma. In contrast, modern concepts emphasize that hypovolemia, hypoperfusion, and reperfusion can lead to inflammatory organ injury. Also, impaired right-to-left blood flow leads to preferential perfusion of the dependent (West Zone III) lung segments that are poorly ventilated, thus also increasing shunt. Chest injury may be associated with myocardial dysfunction as well, but this is typically right ventricular in nature, and resolves quickly.30 Shock and resuscitation do in fact lead to some expansion of extravascular water, but pulmonary lymphatics protect the lung from interstitial overload remarkably well.31 We therefore stress maintaining euvolemia and circulatory adequacy in patients with chest injuries. In patients with underlying cardiac, renal, or hepatic disease, however, extravascular lung-water accumulation may be a significant issue. These patients may require inotropes, diuretics, or oncotic support.
DIAGNOSIS
Physical Examination
The diagnosis of flail chest is best made by visual inspection or palpation of asymmetric chest wall movement in the spontaneously breathing patient. Palpation is often the more sensitive test. It is rapid and informative but is often overlooked. Mobile segments of chest wall and sternum can often be palpated even when not visible on inspection. Clinical flail chest is associated with worse outcomes and greater need for intubation than pulmonary contusion alone.32 Spontaneously breathing patients are often best examined by placing both hands on the two hemithoraces and palpating the symmetry of chest wall motion. Crepitance is also a common finding and point tenderness over the costochondral junctions may point to dislocations or cartilaginous fractures that are not visible on radiographs. Auscultation of the chest is usually suboptimal in trauma, and will play little role in the diagnosis of pulmonary contusion and flail chest except to diminish concern for lesions (such as pneumothoraces) that may deteriorate acutely.
Chest X-Rays
Chest x-ray (CXR) and computed tomography (CT) of the chest play key roles in the diagnosis of chest trauma. The initial anteroposterior/supine chest x-rays that are typically done in seriously injured patients may show pulmonary contusion or suggest flail chest (Figure 3).
But CXRs are of low sensitivity and will miss many very important intrathoracic lesions. Although rib fractures may also be apparent, plain x-rays show many fewer fractures than CTs. Thus, in addition to underestimating pain and disability, CXRs will rarely suggest whether rib fracture patterns are likely to be mechanically unstable. So, although the initial CXR remains crucial in the early diagnosis of immediately life-threatening lesions, it often fails to diagnose pulmonary contusions, hemothoraces, pneumothoraces, and lung lacerations that may require specific interventions. This is especially true where anterior pneumothoraces and posterior fluid collections coexist (Figure 4).
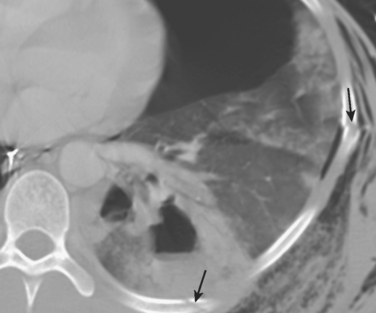
Figure 4 Initial chest computed tomography of same patient as in Figure 3. Multiple segmental rib fractures (arrows) were not seen on CXR. A posterior contusion-laceration and an anterior pneumothorax are present despite the chest tube laterally and the apparent expansion of the lung on CXR. This reflects the superimposition of air and fluid densities. CXR, Chest x-ray.
Chest Computed Tomography
Chest CT typically reveals many more rib fractures than CXR, and the distribution of the injuries may sometimes suggest the likelihood of their causing chest wall musculoskeletal dysfunction (Figure 5). Significant flail segments most commonly occur in the setting of segmental fractures of three or more contiguous ribs. CT scans will sometimes demonstrate contiguous rib fractures in a pattern that suggests geographic instability or a dysfunctional area of the chest wall where physical exam is unrevealing.
Contusions are often seen as infiltrative lesions that underlie fractures and are nonanatomic in their distribution on chest CT. But dependent infiltrates on the CT may reflect processes like aspiration, atelectasis, and later, pneumonia. These can be difficult to tell apart from contusions. In contrast, nonanatomic and antidependent distributions of infiltrative lung lesions on a chest CT may be pathognomonic for pulmonary contusion (Figure 6). A pleural-based “blast-wave” pattern seen on CT is pathognomonic (see Figure 1). Early chest CT can also aid in the evaluation of pulmonary contusions by determining their extent and allowing prediction of respiratory deterioration.12
More heavily muscled areas of the chest are less likely to sustain flail injuries. Moreover, such areas can remain mechanically stable despite the presence of fractures. Thus, typically, visible flail segments are anterior or inferolateral in the chest. But symmetric bilateral multiple fractures in the anterior chest will also commonly cause flail injuries (Figure 7), especially in elderly persons striking a steering wheel. This mechanism can lead to a flail sternum, or if in the axillary lines, a flail of the entire anterior chest wall en cuirasse (i.e., like a shield).
Physiologic Studies
In truly isolated flail chest injuries, there is little initial hypoxemia. Rather, these patients present early with rapid shallow respiration, and they may develop hypercarbia as ventilation fails. More often, though, they do have some element of pulmonary contusion, or they develop hypoxemia over time because of atelectasis or poor clearance of secretions. Ventilated patients may show little pulmonary dysfunction resulting from the flail chest component of their injury as long as their pressure or volume support is sufficient to splint the chest wall and cause it to move in synchrony.
ANATOMIC LOCATION OF INJURY AND INJURY GRADING
The American Association for the Surgery of Trauma-Organ Injury Scale (AAST-OIS) for chest trauma as reviewed by Moore and colleagues33 is shown in Tables 1 and 2. It should be noted that the scores for chest wall and pulmonary injury are separated, but injuries may overlap and interact. For instance, the association of a flail chest with pulmonary contusion results in worse outcomes and greater need for intubation than pulmonary contusion only.32 Conversely, many of the small pulmonary contusions now found on CT scan alone may be of little predictive value, or their significance may be limited to situations where patients are expected to undergo long-bone fixation.34 Thus, these scales must clearly be in evolution at the time of CT diagnosis of intrathoracic injury (see Tables 1 and 2).
MANAGEMENT
Immediate Management
Before the early 1980s, the major controversy in early management of pulmonary contusion/flail chest was whether early endotracheal intubation should be emphasized or whether attempts should be made to avoid intubation. This controversy reflected the early perception that patients who were intubated had a worse prognosis. Richardson and colleagues35 were the first to show that, rather than being a causal relationship, this difference in outcomes reflected worse overall injuries in the intubated than the nonintubated group. Over time, the established approach (as reflected in ATLS and other algorithms) became early elective use of endotracheal intubation in patients presenting with any hypoxemia. Flail chest injuries in particular are associated with a tendency to early ventilatory failure requiring emergency intubation resulting from unrecognized high work of breathing.32 This approach is controversial, however, and other workers have suggested aggressive attempts to avoid intubation.36,37 Analysis of the available data, however, suggests that there are no true prospective series available comparing early intubation to “intubation on demand” in equivalent groups, and that in all reports the intubated patients were simply sicker. Such sicker patients simply require intubation more often. Thus, in our judgment arguments that elective intubation per se leads to worse outcomes are unsupported. Our approach has been the use of early rapid-sequence intubation and ventilation to facilitate diagnosis and management wherever there are severe injuries, with early extubation of patients who will tolerate it. Patients who have progressive deterioration of respiratory function despite intubation will require prolonged ventilation and intensive care unit (ICU) management.
Intensive Care Unit Management
Pulmonary contusion decreases parenchymal compliance and increases QS/QT. Flail chest causes chest wall dysfunction, pain, and inefficient mechanical ventilation. These two injuries contribute synergistically to hypoventilation and ventilatory failure. Pain and impaired coughing contribute to atelectasis and mucus plugging, and decreased chest wall expansion under flail segments decreases functional residual capacity (FRC).38 Associated systemic injury, shock, and pulmonary infections contribute to secondary pulmonary parenchymal injury. All will contribute to shunting and hypoxemia. Thus, management of flail chest–pulmonary contusion is one of the most important and challenging aspects of intensive care in trauma.
General Principles of Ventilator Management
The management of flail chest/pulmonary contusion consists in great measure of the support of failing pulmonary function by the use of a ventilator. In the case of minor injuries, supplemental oxygen can be given by mask as needed. True continuous positive airway pressure (CPAP) delivered by a tight-fitting mask has been used to improve oxygenation and support the functional residual capacity, but the natural history of significant flail chest/pulmonary contusion is the gradual worsening of pulmonary function over the first few days. Thus, in our experience, CPAP is most commonly a marker for the later need for emergent intubation with its attendant problems. Moreover, because CPAP predisposes to gastric distention and thus to aspiration, we have found that using CPAP as a bridge to delay intubation in major chest trauma is usually unwise and may risk significant complications. Because endotracheal intubation will be required at some point in most patients with significant injuries, intubation should be considered early, before deterioration, and when considered is usually warranted.
Ventilatory Support
Ventilating chest trauma patients can be different from ventilating other patients. Intubated patients with lesser injuries may require some support for air exchange, but generally should be allowed to spontaneously ventilate to whatever degree is possible. We find that pressure support ventilation (PSV) mode is very satisfactory for such spontaneously breathing patients. But care should be taken using PSV in the presence of significant flails. In these cases, the negative pressures that trigger the ventilator may destabilize the chest wall39 and should be minimized. Such motion may also be painful and delay stabilization. We often prefer to begin treatment using a volume mode like synchronized intermittent mandatory ventilation (SIMV), keeping minute ventilation high enough to raise arterial pH slightly above 7.4, thus suppressing spontaneous ventilation without undue sedation. The flail segments are allowed to stabilize over about a week, with thicker chest walls often stabilizing more quickly. Patients are then ventilated using PSV and weaned progressively.
Chest trauma, pulmonary contusion, and ARDS can cause the chest to be noncompliant. In such cases, relatively high airway pressures are needed to sustain the traditional high tidal volumes (10–15 ml/kg) used before the ARDS-Net studies. High airway pressures (≤35 cm H2O) should be avoided where possible, and using lower tidal volumes (6–8 ml/g) will decrease peak airway pressures. Limiting airway pressures is especially important in cases of bronchopleural fistulas. Also, “normal” PaCO2 values are not needed in sedated patients, and permissive hypercapnia is often useful in the treatment of hypoxemia after chest trauma.40,41
Oxygenation Support
Patients with significant chest trauma all manifest some degree of hypoxemia. This may be managed initially by increasing FIO2, but prolonged high FIO2 can be harmful in itself. The longer-term management of hypoxemia therefore entails measures to increase mean airway pressure to maintain and improve oxygenation. This may include PEEP or reversed inspiratory/expiratory (I/E) times that can be delivered by any of several ventilator strategies. These interventions can recruit alveoli and diminish alveolar and interstitial water. The reversal of I/E time is limited by the need to excrete CO2 and stacking or auto-PEEP at high I/E ratios. Traditional high tidal volume ventilation (10–15 ml/kg) does not contribute to pulmonary expansion, nor does it improve oxygenation in the majority of cases. Rather, it has been known for many years to lead to unequal ventilation, alveolar overdistension, and VILI.42 We began using low tidal volume ventilation in the early 1990s (then known as “the kinder, gentler vent breath”). Since that time, low tidal volume ventilation has been shown to improve the survival of general ICU patients in prospective studies,43 and we extend these principles to chest trauma in most cases.
Tracheobronchial toilet in the intubated chest trauma patient should have a high priority because of the frequent coexistence of early particulate aspiration, blood casts or lobar collapse resulting from retained secretions. Both macroaspiration at the time of injury and the continued microaspiration that accompanies endotracheal intubation can increase secretions and allow airway colonization by oropharyngeal flora. Excessive secretions will also lead to lobar collapse, shunting and hypoxemia, diminished compliance, and post-obstructive airway infections. Blood may accumulate in the airway after pulmonary contusion and form bronchial casts. Intubated patients cannot cough and are completely dependent upon suctioning for airway toilet. N-acetylcysteine may be used as a mucolytic if secretions are thick, but it causes bronchospasm and should be used with bronchodilators. Also, prolonged N-acetylcysteine therapy can cause bronchorrhea. Chest physiotherapy is helpful, but the percussion of injured ribs is often painful. Thus, removal of secretions or particulates frequently requires bronchoscopy. The removal of blood casts may require retrieval with snares or morcellation (Figure 8).
Pain Management
The control of chest wall pain is a key consideration in management of chest injuries. Immobility of the chest wall due to splinting resulting from pain is often thought a major contributor to the development of pneumonia after rib fractures. This has never been proven and recent studies suggest that pneumonia in trauma patients, in fact, reflects the suppression of innate immunity.44,45 Nonetheless, good pain control is an important contribution to patient care in general, and probably improves pulmonary toilet. Chest wall pain can be treated with appropriate systemic analgesic regimens or with intercostal, intrapleural, subpleural, or paravertebral blocks. Epidural blocks are probably the optimal form of analgesia where possible and not contraindicated.46,47 There is no scientific support for the historic practice of using chest taping or strapping to relieve pain.
Tracheostomy
As with endotracheal intubation, it is often apparent early in patients with severe injuries that tracheostomy will be critical. Also, later deterioration often makes tracheostomy hazardous. Thus, experienced clinicians will often look for an early window of opportunity to move airway access from the endotracheal tube to a tracheostomy in sicker patients. Early tracheostomy (generally defined as at <7 days) improves access to the tracheobronchial tree for toilet and allows for better oropharyngeal hygiene. While controversy persists, this approach has been suggested to result in fewer episodes of pneumonia and more rapid weaning from mechanical ventilation.48,49 Last, current studies have shown convincingly that low volume/low pressure ventilation is less injurious to the lung than higher ventilator volumes and pressures. Tracheostomy diminishes the anatomic dead space, and reliably lowers peak airway pressures at equivalent levels of alveolar ventilation. This is especially helpful in patients with refractory pulmonary failure who require aggressive ventilator settings to support oxygenation.
Operative Stabilization of Flail Chest
Most physiologically significant flail chest injuries are satisfactorily managed by selective intubation and mechanical ventilation. Nonetheless, several groups have advocated the operative management of flail chest injuries.50,51 Evidence that rib fracture stabilization is useful in major chest trauma is scanty. The existing series are highly selected, and in all published series the patients with the most severe pulmonary dysfunction were not considered surgical candidates. Voggenreiter and colleagues52 showed that patients who had flail chests and also had significant pulmonary contusions did not benefit from operation. Thus, chest wall fixation permitted early extubation only in a highly selected group of patients that had ventilatory insufficiency without pulmonary contusion, and presumably therefore without hypoxemia. Consequently, fixation can stabilize flail segments, but the significant physiologic deficits in chest trauma patients relate to underlying contusions and acute lung injury. In summary, the literature currently supports the concept that operative chest wall stabilization will not benefit the vast majority of patients with flail chest. Moreover, it has significant potential for causing mischief in sick patients. The net risk-to-benefit ratio may therefore be most favorable in the subset of patients with large unstable flail segments who already must undergo a thoracic procedure (such as a decortication) for related injuries.
MORTALITY
Most deaths after pulmonary contusion/flail chest result from associated injuries like head trauma, but major chest wall trauma can be an independent cause of death. In these instances, death is usually due to ARDS, respiratory failure, sepsis, and multiple organ failure. So these outcomes are covariant with other conditions that predispose to SIRS, respiratory failure, and MOF. These may include associated injuries, increasing ISS or APACHE scores, and increasing numbers of blood transfusions.3,53 Where pulmonary contusions are visible on the admitting chest x-ray of a patient with a flail chest, the need for mechanical ventilation is far higher and mortality is more than doubled when compared with either condition alone.54,55 The number of ventilator days, ICU days, and overall length of stay, as well as pneumonia and mortality, are all clearly higher in older patients with rib fractures.56–58 Similarly, the mortality of patients with a flail chest increases with age.59 Last, ventilator-associated pneumonia is an independent risk factor for death in chest trauma,60 although pneumonia itself may simply be a marker for greater systemic trauma.44,61 In summary, flail chest and pulmonary contusion are highly morbid and may contribute significantly to mortality in multisystem trauma or in patients with underlying comorbidities. Nonetheless, with good ICU treatment death from flail chest and pulmonary contusion alone should be uncommon.
CONCLUSION



1 Laurent EA. Rupture of both lungs without external injury. Lancet. 1883;2:25.
2 Athanassiadi K, Gerazounis M, Theakos N. Management of 150 flail chest injuries: analysis of risk factors affecting outcome. Eur J Cardiothorac Surg. 2004;26(2):373-376.
3 Freedland M, Wilson RF, Bender JS, Levison MA. The management of flail chest injury: factors affecting outcome. J Trauma. 1990;30(12):1460-1468.
4 Landercasper J, Cogbill TH, Strutt PJ. Delayed diagnosis of flail chest. Crit Care Med. 1990;18(6):611-613.
5 Hooker DR. Physiological effects of air concussion. Am J Physiol. 1924;67:219.
6 Lockwood AL. Surgical experiences in the last war. BMJ. 1940;1:356.
7 Savage O. Pulmonary concussion (“blast”) in non-thoracic battle wounds. Lancet. 1945:424.
8 Burford TH, Burbank B. Traumatic wet lung. J Thorac Surg. 1945;14:415.
9 Hadfield G, Christie RV. A case of pulmonary concussion (“blast”) due to high explosive. BMJ. 1941;1:77.
10 Demuth WEJr, Smith JM. Pulmonary contusion. Am J Surg. 1965;109:819-823.
11 Galan G, Penalver JC, Paris F, et al. Blunt chest injuries in 1696 patients. Eur J Cardiothorac Surg. 1992;6(6):284-287.
12 Miller PR, Croce MA, Bee TK, et al. ARDS after pulmonary contusion: accurate measurement of contusion volume identifies high-risk patients. J Trauma. 2001;51(2):223-228. discussion 229–230
13 Balci AE, Kazez A, Eren S, et al. Blunt thoracic trauma in children: review of 137 cases. Eur J Cardiothorac Surg. 2004;26(2):387-392.
14 Reinberg O, Mir A, Genton N. Characteristics of thoracic injuries in children. Chir Pediatr. 1990;31(3):139-145.
15 Cardenas N, Manrique TA, Catlin EA. Flail chest in the newborn. A complication of osteogenesis imperfecta. Clin Pediatr (Phila). 1988;27(3):161-162.
16 Argyros GJ. Management of primary blast injury. Toxicology. 1997;121(1):105-115.
17 Garcia VF, Gotschall CS, Eichelberger MR, et al. Rib fractures in children: a marker of severe trauma. J Trauma. 1990;30(6):695-700.
18 Orfeo T, Doherty JM, Adey G, et al. Sublethal percussion trauma in vitro causes a persisting derangement in the nonthrombogenic properties of brain endothelial cells. J Trauma. 1994;37(3):347-357.
19 Gourin CG, Shackford SR. Influence of percussion trauma on expression of intercellular adhesion molecule-1 (ICAM-1) by human cerebral microvascular endothelium. J Trauma. 1996;41(1):129-135.
20 de Perrot M, Liu M, Waddell TK, et al. Ischemia-reperfusion-induced lung injury. Am J Respir Crit Care Med. 2003;167(4):490-511.
21 Brackett DJ, McCay PB. Free radicals in the pathophysiology of pulmonary injury and disease. Adv Exp Med Biol. 1993;366:147-163.
22 Turnage RH, Guice KS, Oldham KT. Pulmonary microvascular injury following intestinal reperfusion. New Horiz. 1994;2(4):463-475.
23 Dos Santos CC, Slutsky AS. Invited review: mechanisms of ventilator-induced lung injury: a perspective. J Appl Physiol. 2000;89(4):1645-1655.
24 Hauser CJ, Zhou X, Joshi P, et al. The immune microenvironment of human fracture/soft-tissue hematomas and its relationship to systemic immunity. J Trauma. 1997;42(5):895-903.
25 Pape HC, van Griensven M, Rice J, et al. Major secondary surgery in blunt trauma patients and perioperative cytokine liberation: determination of the clinical relevance of biochemical markers. J Trauma. 2001;50(6):989-1000.
26 Pelosi P, Cereda M, Foti G, et al. Alterations of lung and chest wall mechanics in patients with acute lung injury: effects of positive end-expiratory pressure. Am J Respir Crit Care Med. 1995;152(2):531-537.
27 Shorr RM, Crittenden M, Indeck M, et al. Blunt thoracic trauma. Analysis of 515 patients. Ann Surg. 1987;206(2):200-205.
28 Hauser CJ, Desai N, Fekete Z, et al. Priming of neutrophil [Ca2]i signaling and oxidative burst by human fracture fluids. J Trauma. 1999;47(5):854-858.
29 Pape H-C, Aufmkolk M, Paffrath T, et al. Primary intramedullary femur fixation in multiple trauma patients with associated lung contusion—a cause of posttraumatic ARDS. J Trauma. 1993;33(4):540-548.
30 Harley DP, Mena I, Narahara KA, et al. Traumatic myocardial dysfunction. J Thorac Cardiovasc Surg. 1984;87(3):386-393.
31 Erdmann AJ3rd, Vaughan TR, Brigham KL, et al. Effect of increased vascular pressure on lung fluid balance in unanesthetized sheep. Circ Res. 1975;37(3):271-284.
32 Velmahos GC, Vassiliu P, Chan LS, et al. Influence of flail chest on outcome among patients with severe thoracic cage trauma. Int Surg. 2002;87(4):240-244.
33 Moore EE, Cogbill TH, Malangoni MA, et al. Organ injury scaling. Surg Clin North Am. 1995;75(2):293-303.
34 Pape H-C, Regal G, Dwenger A, et al. Influences of different methods of intrameduallary femoral nailing on lung function in patients with multiple trauma. J Trauma. 1993;35(5):709-716.
35 Richardson JD, Adams L, Flint LM. Selective management of flail chest and pulmonary contusion. Ann Surg. 1982;196(4):481-487.
36 Bolliger CT, Van Eeden SF. Treatment of multiple rib fractures. Randomized controlled trial comparing ventilatory with nonventilatory management. Chest. 1990;97(4):943-948.
37 Vidhani K, Kause J, Parr M. Should we follow ATLS(R) guidelines for the management of traumatic pulmonary contusion: the role of non-invasive ventilatory support. Resuscitation. 2002;52(3):265-268.
38 Gyhra A, Torres P, Pino J, et al. Experimental flail chest: ventilatory function with fixation of flail segment in internal and external position. J Trauma. 1996;40(6):977-979.
39 Cappello M, Legrand A, De Troyer A. Determinants of rib motion in flail chest. Am J Respir Crit Care Med. 1999;159(3):886-891.
40 Eisner MD, Thompson T, Hudson LD, et al. Efficacy of low tidal volume ventilation in patients with different clinical risk factors for acute lung injury and the acute respiratory distress syndrome. Am J Respir Crit Care Med. 2001;164(2):231-236.
41 Bigatello LM, Patroniti N, Sangalli F. Permissive hypercapnia. Curr Opin Crit Care. 2001;7(1):34-40.
42 Slutsky AS. Lung injury caused by mechanical ventilation. Chest. 1999;116(1):9S-15S.
43 ARDS-Network. Ventilation with lower tidal volumes as compared with traditional tidal volumes for acute lung injury and the acute respiratory distress syndrome. The Acute Respiratory Distress Syndrome Network. N Engl J Med. 2000;342(18):1301-1308.
44 Tarlowe MH, Duffy A, Kannan KB, et al. Prospective study of neutrophil chemokine responses in trauma patients at risk for pneumonia. Am J Respir Crit Care Med. 2004;23:23.
45 Perl M, Gebhard F, Bruckner UB, et al. Pulmonary contusion causes impairment of macrophage and lymphocyte immune functions and increases mortality associated with a subsequent septic challenge. Crit Care Med. 2005;33(6):1351-1358.
46 Mackersie RC, Karagianes TG, Hoyt DB, et al. Prospective evaluation of epidural and intravenous administration of fentanyl for pain control and restoration of ventilatory function following multiple rib fractures. J Trauma. 1991;31(4):443-449. discussion 449–451
47 Mandabach MG. Intrathecal and epidural analgesia. Crit Care Clin. 1999;15(1):105-118. vii
48 Moller MG, Slaikeu JD, Bonelli P, et al. Early tracheostomy versus late tracheostomy in the surgical intensive care unit. Am J Surg. 2005;189(3):293-296.
49 Griffiths J, Barber VS, Morgan L, et al. Systematic review and metaanalysis of studies of the timing of tracheostomy in adult patients undergoing artificial ventilation. BMJ. 2005;330(7502):1243. Epub May 18, 2005
50 Lardinois D, Kreuger T, Dusmet M, et al. Pulmonary function testing after operative stabilisation of the chest wall for flail chest. Eur J Cardiothorac Surg. 2001;20(3):496-501.
51 Ahmed Z, Mohyuddin Z. Management of flail chest injury: internal fixation versus endotracheal intubation and ventilation. J Thorac Cardiovasc Surg. 1995;110(6):1676-1680.
52 Voggenreiter G, Neudeck F, Aufmkolk M, et al. Operative chest wall stabilization in flail chest—outcomes of patients with or without pulmonary contusion. J Am Coll Surg. 1998;187(2):130-138.
53 Gaillard M, Herve C, Mandin L, et al. Mortality prognostic factors in chest injury. J Trauma. 1990;30(1):93-96.
54 Johnson JA, Cogbill TH, Winga ER. Determinants of outcome after pulmonary contusion. J Trauma. 1986;26(8):695-697.
55 Clark GC, Schecter WP, Trunkey DD. Variables affecting outcome in blunt chest trauma: flail chest vs. pulmonary contusion. J Trauma. 1988;28(3):298-304.
56 Holcomb JB, McMullin NR, Kozar RA, et al. Morbidity from rib fractures increases after age 45. J Am Coll Surg. 2003;196(4):549-555.
57 Taylor MD, Tracy JK, Meyer W, et al. Trauma in the elderly: intensive care unit resource use and outcome. J Trauma. 2002;53(3):407-414.
58 Bulger EM, Arneson MA, Mock CN, et al. Rib fractures in the elderly. J Trauma. 2000;48(6):1040-1046. discussion 1046–1047
59 Albaugh G, Kann B, Puc MM, et al. Age-adjusted outcomes in traumatic flail chest injuries in the elderly. Am Surg. 2000;66(10):978-981.
60 Magnotti LJ, Croce MA, Fabian TC. Is ventilator-associated pneumonia in trauma patients an epiphenomenon or a cause of death? Surg Infect (Larchmt). 2004;5(3):237-242.
61 Tarlowe MH, Kannan KB, Itagaki K, et al. Inflammatory chemoreceptor cross-talk suppresses leukotriene b(4) receptor 1-mediated neutrophil calcium mobilization and chemotaxis after trauma. J Immunol. 2003;171(4):2066-2073.