Chapter 22 Prostate Cancer
Epidemiology and Risk Factors
Prostate carcinoma is the most frequently diagnosed visceral cancer and the second most common cause of cancer death among American men.1 African American and Jamaican men of African descent have the highest incidence rates in the world. Geographically, the disease is more common in North America and northwestern Europe than in Asia and South America.2 With the advent of a method to potentially detect the disease using PSA blood testing, the incidence of prostate cancer increased rapidly between 1982 and 1992 and has risen less dramatically since. Greater than 70% of cases are diagnosed in men older than age 65. Since 1995, the incidence has risen less sharply in men younger than 65 and leveled off in men older than 65 years old,2 suggesting that the impact of PSA on the detection of prostate cancer in the population has leveled off. The use of PSA for screening is currently controversial. Ethnicity can influence one’s chances of both getting and surviving the disease. In the United States, African American men have both a higher incidence of and more than twice the death rate from prostate cancer than whites. Fortunately, death rates from prostate cancer have been declining, particularly since the mid 1990s. Some of the decline may be attributed to earlier diagnosis and therapeutic intervention. Five-year survival is effectively 100% when disease is local or regional, but drops to 32% with distant metastases. For all stages, survival is 99% at 5 years, 93% at 10 years, and 79% at 15 years.2
Established risk factors include age, ethnicity, and family history. The latter is a contributor in only 5% to 10% of cases,2 and the risk increases with a greater number of first-degree relatives affected and younger age of diagnosis in those relatives. Furthermore, patients with a family history tend to be diagnosed with prostate cancer at an earlier age.3 Environmental factors, including diets high in saturated fat, may increase risk, and obesity may increase the risk of dying from the disease.4 An antioxidant, lycopene, may reduce risk. It is found in red and pink foods such as tomatoes.2
Anatomy and Histology
The prostate gland is bounded superiorly at its base by the urinary bladder and inferiorly by the urogenital membrane. It is encircled by the levator ani bilaterally, the symphysis pubis anteriorly, and the rectum posteriorly. In the model by McNeal and coworkers,5 the prostate gland is divided into four zones—the transition zone, the central zone, the peripheral zone, and the nonglandular anterior fibromuscular stroma (Figure 22-1)—that contain 5%, 20%, 70% to 80%, and 0% of glandular tissue and 25%, 5%, 70%, and 0% of prostate cancers, respectively. Cancers resolvable by conventional imaging are essentially within the peripheral zone. The transition zone is most susceptible to age-related benign prostatic hyperplasia (BPH). Normally, it composes only 5% to 10% of the total glandular tissue of the prostate, but in older men with BPH, it can compose the majority of prostate tissue. The transition zone cannot be separated from the central zone by imaging, and on images, the two together are often referred to as the central gland or zone. The central zone is cone shaped, with its widest portion at the base of the bladder and its apex at the verumontanum. The seminal vesicles are located posterior and superior to the prostate gland and give off the ejaculatory ducts, which traverse the central zone, providing a conduit for seminal fluid from the seminal vesicles to the urethra. The relative amount of peripheral zone to central zone increases from the base of the gland to the apex of the gland. Inferiorly, the peripheral zone comprises all of the prostatic glandular tissue at the apex, and superiorly, it invests the posterior portion of the central zone.
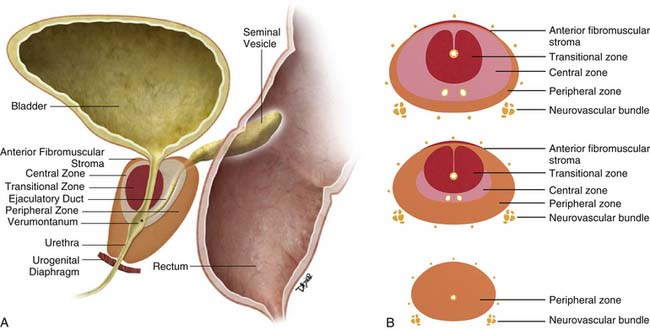
Figure 22-1 Prostate gland anatomy: A, Sagittal view. B, Axial view: top, base; middle, mid gland; bottom, apex.
The prostate gland is partially invested by a coalition of fibrous tissue, historically called the “capsule,” that is most apparent posteriorly and posterolaterally. The capsule is an important landmark for assessing extraprostatic tumor extension (EPE). The neurovascular bundles course posterolateral to the prostate capsule bilaterally at 5 and 7 o’clock (see Figure 22-1). At the apex and the base, the bundles send penetrating branches through the capsule, providing a route for EPE. The normal fat plane between the prostate gland and the neurovascular bundles seen on imaging is lost when there is neurovascular involvement by prostate carcinoma. Whereas perineural invasion by prostate cancer on biopsies does not seem to correlate with positive margins or poorer survival, it may be indicative of EPE.6,7 Identifying tumor involvement of the neurovascular bundles is important when planning for nerve-sparing prostatectomy. The neurovascular bundles run within the substance of the parietal pelvic fascia, also termed the lateral prostatic fascia. During nerve-sparing radical prostatectomy, this fascia is incised anterior to the bundles and reflected off the prostate capsule.
Histologically, 95% of prostate cancers are adenocarcinomas that develop from the acini of the prostatic ducts. Other histologic types include small cell carcinoma (the most common variant); mucinous, ductal, squamous, sarcomatoid, and transitional cell carcinomas; adenoid basal cell tumors; and malignant mesenchymal tumors.8
In the United States, histologic evaluation of prostate adenocarcinomas is performed using the Gleason grading system. Tumors are assigned a primary grade on the basis of the predominant pattern of differentiation and a secondary grade on the basis of the second most common pattern. The two numbers are added to produce a Gleason score. For example, a tumor described as “Gleason grade 3 + 4 or 4 + 3” will have a Gleason score of “7.”9 The biologic behavior of a Gleason score 4 + 3 is more aggressive than 3 + 4 regardless of the number of cores.10
Cancers with Gleason scores of 6 or lower are considered well-differentiated and are associated with a good prognosis. Those with a Gleason score of 8 to 10 have the worst prognosis and the highest risk for recurrence.11 Tumors with Gleason scores of 7 have a variable prognosis and an intermediate risk of recurrence. Serum PSA is not useful in uncommon high-grade tumors that do not produce PSA.11 Prostate cancer tends to be multifocal and the Gleason score at biopsy may differ from that obtained at surgery.12
Key Points Anatomy and Histology
• The prostate is divided into the central zone and the peripheral zone. By imaging, the peripheral zone is better evaluable for tumor.
• The base of the gland is near the bladder neck, and the apex is near the urogenital diaphragm.
• The neurovascular bundles are at 5 and 7 o’clock.
• Histology is defined by the Gleason score, which consists of two numbers added. The predominant type goes first, for example, 4 + 3 = 7 Gleason score with the predominant type being 4.
Clinical Presentation
At presentation, early prostate cancer is commonly asymptomatic. When present, local symptoms include difficulty starting or stopping urine flow; painful, weak, or interrupted urine flow; increased frequency; and hematuria. Most of these symptoms may be due to coexistent BPH. A large prostate cancer or a smaller prostate cancer in the transition zone affecting the urethra may cause similar symptoms. The rapid acuity of presentation usually prompts the consideration for cancer; in comparison, the onset is insidious and chronic with BPH. Back, pelvis, or thigh pain may indicate distant metastatic disease. Unfortunately, the symptoms are not specific to prostate cancer and can be mimicked by a variety of benign conditions.2 Prostate cancer may be suspected by elevated PSA, digital rectal examination (DRE), or imaging.
Prostate carcinoma is often suspected when an abnormal DRE is noted by physical examination or the serum PSA is elevated. Interobserver agreement among urologists is only fair for detecting malignancy by DRE and DRE is not accurate for staging.13,14 For serum PSA, no specific cutoff point simultaneously provides optimal sensitivity and specificity; instead, it provides a spectrum of risk at all ranges.15 Although a serum PSA level greater than 4 ng/mL has traditionally been considered abnormal, almost 27% of biopsy-proven prostate cancers present with “normal” PSA, and 70% to 80% of patients with “elevated” PSA (>4 ng/mL) do not have prostate carcinoma.16,17 The chance of prostate carcinoma increases with increasing PSA.18
There is controversy regarding using PSA to screen for prostate cancer. If performed after a discussion between the patient and the physician of the risks and benefits of screening, the American Cancer Society (ACS) guidelines for early detection of prostate cancer include annual screening by DRE and serum PSA of men age 50 or older with a 10-year life expectancy. Evaluation may begin at age 40 to 45 for high-risk individuals, such as African Americans and/or patients with a first-degree relative diagnosed with the disease at a young age.2 Although the role of early detection with PSA has been questioned, recent findings suggest that early detection and active treatment were important in the recent lowering of death rates, with the greatest survival impact on younger (age < 65 yr) men.1,19 However, PSA screening has also been cited as a cause of overdiagnosis.20,21 To improve upon traditional serum PSA, derivative tests based on PSA have been and are being developed, for example, PSA density, PSA velocity, age-specific reference range, PSA isoforms, and percent-free PSA.22 Non–PSA-based tests may also improve the diagnostic accuracy of markers, for example, urinary PCA3,23 a noncoding mRNA rarely expressed in benign prostate tissue but overexpressed in the majority of prostate cancer. Incidental findings (e.g., an enlarged prostate, abnormal T2 signal on MRI, or gross features of local invasion, pelvic lymphadenopathy, or sclerotic bone metastasis) on imaging obtained for another reason may initiate workup for prostate cancer.
Key Points Clinical Presentation
• The patient is often asymptomatic or may have nonspecific symptoms such as related to voiding for local disease or bone pain for metastatic disease.
• Controversy exists regarding PSA for routine screening. Derivative and new tests are being developed.
• PSA is useful for monitoring patients diagnosed with prostate cancer.
Patterns of Tumor Spread
Prostate cancer tends to spread by local extension (Figure 22-2). EPE tends to be seen at 5 and 7 o’clock, where the neurovascular bundles send penetrating branches through the capsule. This also provides a route for neurovascular bundle involvement. Further lateral growth may involve the levator ani and then the pelvic sidewalls. Tumor may also spread superiorly to involve the seminal vesicles—either by direct extension or via the ejaculatory ducts—or the bladder, by direct extension or via the urethra. Superior and inferior growth may also involve the two sphincters for micturation, superiorly at the base of the bladder and inferiorly at the urogenital diaphragm. Rarely, the tumor may grow inferiorly along the urethra beyond the membranous urethra. Posteriorly and uncommonly, the tumor may involve the rectum.
Metastases to regional lymph nodes (Figure 22-3) occurs most commonly along the obturator chain (medial to external iliac area) but also occurs along other regional nodes such as along the internal iliac, external iliac, and presacral chains.24 Lymph node metastases may also occur along the common iliac and retroperitoneum chains. Skip metastases to the retroperitoneum may occur in the absence of pelvic lymphatic metastasis. After lymph node resection or radiation therapy to the pelvis, recurrence may occur particularly in retroperitoneal nodes outside the treated field. Hematogenous metastases (see Figure 22-3) tend to occur primarily to the skeleton and are usually blastic. Lytic lesions may be seen with advanced disease. Occasionally, spinal metastases may cause cord compression. Other visceral metastases are associated with advanced disease and are rare; if found, such metastases may suggest less differentiated forms of prostate cancer or another primary cancer.
Key Points Tumor Spread
• EPE most commonly occurs at 5 and 7 o’clock; therefore, it can involve the neurovascular bundles.
• Seminal vesicles or other adjacent organs such as bladder and rectum are less commonly involved.
• Lymphatic metastases are usually to intrapelvic lymph nodes, but skip metastases to retroperitoneal lymph nodes may occur.
• Visceral metastases are most commonly to bone and are most commonly sclerotic.
Staging
The primary goals of staging (Table 22-1) are, in general, to allow for risk stratification and allocation to reasonable treatment strategies. Specifically, this involves distinguishing patients with organ-confined, locally invasive, or metastatic disease and assessing risk of treatment failure. Risk-stratification guides therapy and integrates PSA; tumor-node-metastasis (TNM) clinical staging; and biopsy data including Gleason score, number of cores positive, and amount of cancer in each core (Table 22-2).
Table 22-1 Tumor-Node-Metastasis Staging System for Prostate Cancer
Primary Tumor (T) | |
Clinical | |
Tx | Primary tumor cannot be assessed |
T0 | No evidence of primary tumor |
T1 | Clinically inapparent tumor neither palpable nor visible by imaging |
T1a | Tumor incidental histologic finding in ≤ 5% of tissue resected |
T1b | Tumor incidental histologic finding in > 5% of tissue resected |
T1c | Tumor identified by needle biopsy (e.g., because of elevated PSA)* |
——— For evaluating intraprostatic disease, imaging becomes more useful ——— | |
(Primarily MRI) | |
T2 | Tumor confined within the prostate |
T2a | Tumor involves one half of one lobe or less |
T2b | Tumor involves more than one half of one lobe, but not both lobes |
T2c | Tumor invades both lobes |
——— For evaluating local extension, imaging becomes more useful ——— | |
(Primarily MRI and sometimes CT) | |
T3 | Tumor extends through the prostate capsule† |
T3a | Extracapsular extension (unilateral or bilateral) |
T3b | Tumor invades seminal vesicle(s) |
T4 | Tumor is fixed or invades adjacent structures other than seminal vesicles: bladder neck, external sphincter, rectum, levator muscles, or pelvic wall |
Pathologic (pT) | |
pT2 | Organ confined‡ |
pT2a | Unilateral, involving one half of one lobe or less |
pT2b | Unilateral, involving more than one half of one lobe, but not both lobes |
pT2c | Bilateral disease |
pT3 | Extraprostatic extension |
pT3a | Extraprostatic extension or microscopic invasion into the bladder neck§ |
pT3b | Seminal vesicle invasion |
pT4 | Invasion of bladder (gross), rectum, levator muscles, and/or pelvic sidewall |
Regional Lymph Nodes (N) | |
Clinical | |
Nx | Regional lymph nodes were not assessed |
N0 | No regional lymph node metastasis |
——– For staging and guiding lymph node biopsies, imaging becomes more useful ——– | |
(Primarily CT and MRI) | |
N1 | Metastasis in regional node(s) |
Pathologic | |
PNx | Regional lymph nodes not sampled |
pN0 | No positive regional nodes |
pN1 | Metastases in regional node(s) |
Distant Metastasis (M)![]() |
|
Mx | Distant metastasis cannot be assessed (not evaluated by any modality) |
M0 | No distant metastasis |
——— For staging metastatic disease, imaging becomes more useful ——— | |
(Multiple modalities, radiographs, CT, bone scan, MRI) | |
M1 | |
M1a | Nonregional lymph node(s) |
M1b | Bone(s) |
M1c | Other site(s) with or without bone disease |
CT, computed tomography; MRI, magnetic resonance imaging; PSA, prostate-specific antigen.
* Tumor found in one or both lobes by needle biopsy, but not palpable or reliably visible by imaging, is classified as T1c.
† Invasion into the prostatic apex or into (but not beyond) the prostate capsule is classified not as T3 but as T2.
‡ There is no pathologic T1 classification.
§ Positive surgical margins should be indicated by an R1 descriptor (residual microscopic disease).
When more than one site of metastasis is present, the most advanced category is used. pM1c is the most advanced.
Adapted from Edge SB, Byrd DR, Compton CC, et al, eds. AJCC Cancer Staging Manual. 7th ed. New York: Springer; 2010:525.
Local Staging (T)
Although local tumor staging (see Figure 22-2) is based upon findings from DRE, imaging can be beneficial. Stage T1 describes incidental detection of cancer. For example, stages T1a and T1b may be noted in specimens from transurethral resection of the prostate (TURP) performed for BPH. Stage T1c describes prostate cancer detected by elevated PSA, but not palpable by DRE. Cancer palpable by DRE or imaged on transrectal ultrasonography (TRUS) but confined within the prostatic capsule is considered T2. Cancer extending beyond the capsule is considered T3. This latter group includes EPE, periprostatic neurovascular involvement, and/or seminal vesicle involvement. Stage T4 describes tumor extending to other adjacent structures.25
Metastatic Disease (N and M Staging)
Prostate cancer may metastasize by lymphatic or hematogenous routes. The incidence of metastatic disease is extremely low in patients with stage T1-2 disease, serum PSA less than 20 ng/mL, and Gleason score less than 8.26 Thus, metastatic workup is usually reserved for patients with a Gleason score greater than 7, serum PSA greater than 20 ng/mL, stage greater than T2, or symptoms suggestive of metastasis.26
Metastasis (see Figure 22-3) to regional lymph nodes occurs primarily along the obturator, internal iliac, common iliac, and presacral chains. Metastasis to inguinal lymph nodes is exceedingly rare. It has been suggested that lymph node metastasis occurs stepwise from the pelvis to the retroperitoneum27; however, there are reports that up to 50% of nodal metastases can be para-aortic without a concurrent pelvic nodal metastasis, suggesting hematogenous rather than lymphatic spread.28 Such hematogenous spread potentially occurs via Bateson’s plexus. Importantly, at staging, nodal disease within the pelvis is considered regional nodal metastasis (N1); whereas nodal disease in the common iliac chains and retroperitoneum is considered distant metastasis (M1a). This, as well as the propensity of prostate cancer to metastasize to the lumbar spine, suggests that including the abdomen may be helpful when performing prostate MRI or this area may be evaluated by CT if abdominal CT is already to be performed in the workup of the patient.29
Hematogenous metastasis to bone occurs most frequently to the lumbar spine, pelvis, ribs, and femoral heads (M1b). In advanced disease (M1c), viscera more commonly involved include the liver, adrenal glands, and lungs.25,26
Key Points Staging
• T2 lesions may be visualized by imaging, most commonly by MRI.
• T3 and T4 disease may be visualized by CT and MRI.
• T3 includes EPE and microscopic invasion of the bladder neck and seminal vesicles.
• Regional lymph nodes encompass N1.
• M1a includes nonregional lymph nodes including along the common iliac chain and retroperitoneum.
• Metastases commonly occur to bone whereas visceral organ metastases are rare.
Imaging
Imaging in Local Staging
Transrectal Ultrasound
In patients suspected to have prostate carcinoma, diagnosis is generally made by TRUS-guided biopsy. Because ultrasound has limited sensitivity to distinguish prostate tumors, systematic biopsy is used. Traditionally, it employed a sextant (six site) approach in which a parasagittal plane guided three cores of each lobe (base, mid gland, and apex), yielding an approximately 25% cancer detection rate when serum PSA was between 4 and 20 ng/mL.30 Repeat biopsy demonstrated cancer in approximately 20% of men with persistently elevated serum PSA and negative initial biopsy.31,32 Thus, more cores were tried. Presti and colleagues33 achieved approximately 40% yield using a 10-core approach; even more cores have been advocated34 to minimize sampling errors.
TRUS utilizes a high-frequency transducer (7-10 MHz) to delineate zonal anatomy, but it cannot reliably differentiate between benign and malignant prostate tissue. Tumors have varying appearances on TRUS: approximately 40% to 50% are hypoechoic (Figure 22-4), 40% are isoechoic, and others are hyperechoic.35 Confounding lesions can also present as hypoechoic lesions, for example, prostatitis, atrophy, prostatic epithelial neoplasia, and ductal ectasia.36 Lee and associates35 noted that the positive predictive value for cancer of a hypoechoic area in the peripheral zone alone was 41%, 52% if PSA was greater than 4 ng/mL, and greater than 71% if there was also an abnormal DRE.
Vascular imaging using color or power Doppler ultrasound may demonstrate lesions not visualized by conventional gray-scale ultrasound; however, such lesions are not specific and the new techniques do not perform as well as systematic biopsy in diagnosing prostate cancer.37 Although new intravenous sonographic contrast agents can improve sensitivity for prostate cancer compared with gray-scale imaging from 38% to 69%, the role of contrast-enhanced ultrasound has yet to be determined. It has been reported that TRUS can detect EPE, but accuracy remains suboptimal.38,39
Magnetic Resonance Imaging
EPE is more accurately diagnosed by TRUS or endorectal MRI than by DRE.39 Most studies have shown that high-resolution endorectal MRI provides higher accuracy in staging local disease than does TRUS.40–42 Moreover, adding data from endorectal MRI to Partin nomograms improves prediction of organ-confined prostate cancer versus extraprostatic disease at radical prostatectomy (area under receiver operating curve [ROC] curve 0.81 vs. 0.90, nomogram vs. nomogram plus MRI) in all risk groups, with greatest impact on intermediate- and high-risk groups.43 In the study by Wang and coworkers,43 extraprostatic disease was defined on MRI by extracapsular extension, seminal vesicle invasion, or lymph node metastasis; no statistically significant improvement was noted by the addition of spectroscopy. MRI also allows simultaneous screening of the regional lymph nodes and pelvic bones.
T1-weighted axial images are employed to screen the pelvic nodes and bones for metastasis and to identify hemorrhage in the prostate gland. Zonal anatomy is best seen on T2-weighted images (T2WI) (Figure 22-5), but cannot be distinguished on T1-weighted imaging (T1WI). Typically, the central gland is heterogeneous on T2WI owing to BPH and is isointense on T1WI. In the central zone, it is difficult to separate cancer from the BPH that invariably occurs in this age group. In comparison, the peripheral zone is relatively homogeneous and hyperintense on T2WI and isointense on T1WI. Located at 5 and 7 o’clock, the neurovascular bundles appear as oval in axial and linear in long axis, hypointense structures surrounded by hyperintense fat on T1WI. Periprostatic vessels appear as serpiginous or honeycomb iso- to hyperintense structures on T2WI (Figure 22-6) and should be identified owing to the possibility of hemorrhage at the time of surgery.
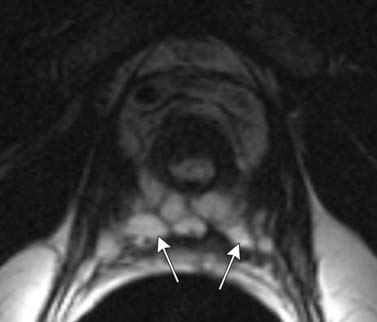
Figure 22-6 Axial MRI demonstrates periprostatic blood vessels (arrows) adjacent to the prostate apex.
In the peripheral zone, prostate tumors appear hypointense on T2WI and isointense on T1WI (Figure 22-7). However, low T2-weighted signal is not specific for cancer. Underlying hemorrhage secondary to biopsy (Figure 22-8), prostatitis, atrophy, or posttreatment change can present with low signal intensity. MRI is generally performed at least 6 weeks after prostate biopsy to allow resolution of hemorrhage, which has become more problematic with extended numbers of core biopsies. Fortunately, hemorrhage can be identified as high signal on T1WI. Unfortunately, areas of high T1 signal compromise detection of tumor in the prostate on T2WIs. Performing axial T1WI and T2WI with the same slice thickness and field of view allows alignment of the two sequences that can aide distinguishing tumor separate from hemorrhage. Using MRI can reduce the number of false-negative biopsies in patients with a previous negative biopsy (negative predictive value of 84-91% and accuracy of 77-78%).44
Magnetic resonance spectroscopic imaging (MRSI) with an endorectal coil has been used to improve tumor detection. MRSI is a metabolic imaging technique, and for prostate cancer, the most commonly studied markers include choline, creatine, and citrate; others include polyamines, lipids, and lysine.45 Most commonly, prostate cancer is identified by increased choline (a byproduct of increased cell membrane metabolism) and decreased citrate, a normal product of prostate metabolism (Figure 22-9). In conjunction with endorectal MRI, MRSI has been reported to provide sensitivity and specificity for prostate cancer as high as 95% and 91%, respectively, and to provide similar accuracy to sextant biopsy for intraprostatic tumor localization, except at the apex where MRI with MRSI was more accurate than biopsy.46,47 MRSI may also predict tumor aggressiveness.48 However, obtaining optimal spectral resolution and interpreting MRSI remains challenging, and efficacy compared with T2WI or other functional techniques is unclear. Drawbacks of MRSI include relatively poor spatial resolution (~4 mm), technical demand, artifacts, inability to directly predict the periprostatic area, and requirement of specialized software and expertise in acquiring and interpreting the data.
The main roles of MRI in prostate cancer currently are local staging of tumors in patients with an intermediate risk for treatment failure11 and assessing prostate size. The formula for a prolate ellipse is commonly used (anteroposterior × transverse × cephalocaudal × 0.52) to calculate prostate volume. Jager and colleagues49 suggested that MRI is cost-effective when used for the patients with a prior probability of EPE of at least 30% (PSA >10 or Gleason score >7). EPE or seminal vesicle invasion is associated with a higher risk of recurrence after resection.50 The accuracy of endorectal MRI in detecting EPE can vary between 58% and 90% depending on the criteria and techniques used.51 For patients at intermediate risk, it has shown approximately 80% accuracy in predicting the pathologic stage.41,42
Specificity is more important than sensitivity for determining EPE in order to prevent patients from being excluded from potentially curative treatment.52 The most specific findings for EPE include asymmetry of the neurovascular bundle, blunting of the rectoprostatic angle, and direct tumor extension outside of the capsule (Figure 22-10); these provide a specificity of greater than 90%.51 Focal bulging (Figure 22-11) is often used but is less specific.51 Because microscopic extracapsular tumor extension does not affect patient survival, subtle findings suggesting capsular penetration such as irregular bulge, irregular gland contour, focal capsular thickening, or retraction may not influence management.53
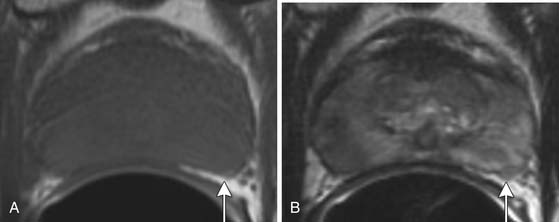
Figure 22-11 Focal bulge (arrow) is not a specific sign for extraprostatic extension. Axial MRI. A, T1-weighted. B, T2-weighted.
Neurovascular bundle invasion is often better assessed on T1WI than T2WI and may appear as asymmetric enlargement with loss of the intervening periprostatic fat plane or as gross tumor extension.54 In the seminal vesicles, tumor appears as a low signal area within the high signal fluid on T2WI and as low signal on T1WI (Figure 22-12). In contrast, postbiopsy hemorrhage usually has high T1 signal and either low or high T2 signal. Seminal vesicle involvement is also suggested by asymmetry or loss of the fat plane between the base of the bladder and the inferior aspect of the seminal vesicle that is usually better noted on coronal images, by focal or diffuse wall thickening, or by nonvisualization of the ejaculatory ducts or seminal vesicle wall.55
T4 disease involves invasion into structures other than the seminal vesicles and is indicated by the loss of the fat plane between the tumor and the adjacent structure or by direct tumor visualization of tumor in the adjacent structure (Figure 22-13).
Imaging Advanced Disease
Computed Tomography
CT scans of the abdomen and pelvis are obtained primarily in patients with suspected metastatic disease to lymph node (Figure 22-14) and/or bone. As on MRI (see Figures 22-12 and 22-14), lymph nodes greater than 1 cm in short axis are considered suspicious for metastasis by CT. Rare metastases to other solid organs, such as lung, liver, pleura, and adrenal glands, may also be detected by CT (Figure 22-15) or MRI.28,29 The chest is commonly evaluated by radiography, but a chest CT may be obtained when a suspicious lesion is noted.
Bone Scan, Radiographs, and Magnetic Resonance Imaging
Bone scan, radiographs, and MRI are used for detecting visceral and bone metastases. Bone scan most commonly employs 99mTc-methylene diphosphate (MDP) and is performed on patients with elevated PSA or clinically suspected bone metastasis, for example, due to pain. Because of the low likelihood of skeletal metastasis, a bone scan has been considered unnecessary in patients with newly diagnosed, untreated prostate cancer with PSA less than 10 ng/mL and no clinical signs of bone pathology.56 Patients with PSA greater than 20 ng/mL have a relatively higher risk of bone metastasis. Metastases from prostate cancer usually present as focal areas of increased uptake on bone scan (Figures 22-16 and 22-17). When there are diffuse bone metastases, the radiopharmaceutical may become incorporated into multiple metastases without apparent focal uptake or apparent excretion of the radiopharmaceutical. Nonvisualization of the kidneys is the classic finding of such a “superscan.” Late in disease, very rarely, an aggressive prostate cancer bone metastasis may appear as normal bone or as a cold spot on bone scan. A flair reaction of increased uptake can be seen when therapy decreases the size of a metastasis and healing results. 99mTc-MDP mimics calcium phosphate and is incorporated into bone matrix; thus, it assesses mineralized bone turnover, not the soft tissue tumor itself. Bone scan is a functional technique that is more sensitive for bone metastasis than are anatomic CT or plain radiographs. However, the latter two can aide in distinguishing increased uptake on bone scan due to metastasis from other etiologies, such as degenerative disease, healing fractures, or metabolic disorders and their complications. On the x-ray–based techniques, prostate cancer metastases are most often sclerotic (Figure 22-18). Unlike radiographs, CT can separate bone from overlying tissue. Bone scan, radiographs, and CT visualize the mineralized component of bone primarily. Whereas CT is more sensitive than conventional radiographs for detecting cortical invasion, it is less sensitive than MRI for medullary bone or marrow involvement, where metastasis begins.57 On MRI, bone metastases demonstrate low T1 signal, high T2 signal, and enhance, but highly sclerotic metastases may appear dark on each of these sequences (Figure 22-19). MRI can delineate the soft tissue component of the tumor and is more sensitive than bone scan for detecting bone metastasis.58 An advantage of bone scan is its ability to visualize the entire skeleton. However, newer MRI techniques for evaluating the entire skeleton are being evaluated. Major indications for MRI include evaluation for epidural disease and resolving ambiguity or discrepancy between other modalities.
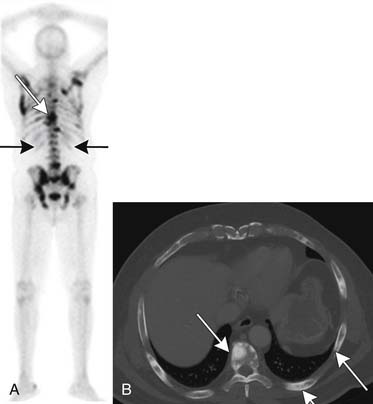
Figure 22-17 Multiple bone metastases (arrows) seen as increased uptake on bone scan (A) and sclerotic lesions on axial CT (B).
Immunoscintigraphy has been used for detecting lymph node metastasis and recurrence. ProstaScint scan, which uses an 111In-labeled murine monoclonal antibody to a transmemberane glycoprotein, prostate-specific membrane antigen (PSMA), may be used when other imaging tests are negative for metastasis but suspicion remains high. PSMA expression is up-regulated in prostate cancer. For detecting lymph node metastases, a sensitivity of 17% to 62% and a specificity of 72% has been reported for ProstaScint.59,60 Compared with using ProstaScint studies alone, a study with a small series of patients suggests that fusion with CT may improve sensitivity and specificity.61 Newer small molecule– and antibody-based imaging methods are being investigated that target the extracellular domain of PSMA instead of the less available intracellular domain targeted by ProstaScint.62
Key Points Imaging
• Ultrasound is used primarily to visualize the prostate and guide biopsy. It is limited in detecting prostate cancer.
• MRI is used for local and metastatic disease.
• Within the prostate, tumor is commonly low signal on T2 and isointense on T1.
• Increased signal on T1 implies hemorrhage and confounds interpretation of T2WIs.
• MRI offers anatomic and functional evaluation.
• CT is better used for metastatic disease.
• Bone scan is used for detecting bone metastases and is of little value if PSA is less than 10 unless there is bone pain and one can suggest not obtaining a bone scan unless PSA is greater than 20.
Future Imaging of Prostate Cancer
Classic T1- and T2-based evaluation of prostate cancer has limitations. For intraprostatic cancer, limitations to endorectal MRI at 1.5 T include less than ideal accuracy and, in particular, specificity; inability to distinguish tumors in the central gland, where 30% of prostate cancers arise; inability to distinguish cancer in the presence of hemorrhage; and limited evaluation after hormone or radiation therapy owing to gland shrinkage and diffusely decreased T2 signal.5 Therefore, additional MRI methods are being evaluated. For example, as discussed previously, some studies suggest that adding spectroscopy (see Figure 22-9) is promising for improving accuracy of localizing prostate cancer, particularly improving specificity compared with traditional T1WI and T2WI alone. But in other studies, no significant improvement in accuracy was seen, in particular, by experienced readers. For example, Costouros and associates63 found no significant difference between endorectal MRI and endorectal MRI plus spectroscopy in diagnosing prostate cancer in 40 patients with elevated PSA, and they noted that MRI may supplement, but not replace, biopsy for diagnosis of prostate cancer. Wetter and coworkers64 found no significant improvement in staging endorectal MRI by adding spectroscopy. However, a preliminary study suggested that MRSI can detect prostate tumors in the central zone65: although there was overlap with BPH, the presence of choline implied cancer. MRSI has also shown promise in evaluating for metabolic atrophy posttreatment and for distinguishing recurrence post radiation therapy.66 When combined with endorectal MRI, MRSI improved detection of recurrence within 4 months of hormone therapy compared with endorectal MRI alone for a less experienced reader.67 Improved spatial, temporal, and spectral resolution and potentially new markers should improve spectroscopy.
Functional MRI techniques may be combined but will still depend on the amount of pathology in the voxel. For example, combining MRSI and diffusion-weighted imaging (DWI; Figure 22-20) was superior to MRSI alone when tumor filled 70% of the voxel (area under the curve [AUC] of 0.98 vs. 0.92), but not if tumor filled 30% of the voxel (AUC of 0.81 vs. 0.79).68 This study points to the importance of spatial resolution. DWI measures the restriction of diffusion in biologic tissues and can be acquired rapidly. It may be applied to both the central gland and the peripheral zone. Although DWI alone shows promise, there is not yet a standard acquisition protocol and there appears to be intersubject variability, which may limit utility; further, a precise ADC (apparent diffusion coefficient) cutoff value is as yet uncertain both at 1.5 and 3 T.69 Yet, DWI can identify prostate cancer. Visual inspection may perform as well as quantifying ADC values. Tanimoto and colleagues70 noted that compared with T2WI alone, combining T2WI with DWI improves prostate cancer detection; furthermore, there was a trend to even better test characteristics with addition of dynamic contrast–enhanced (DCE) imaging for assessing early enhancement and late washout (ROC AUC 0.71, 0.91, and 0.97, respectively); however, the utility of multiple functional tests compared with improvement in sensitivity and specificity is debatable. T2WI plus a functional test may be adequate. Addressing prostate cancer in the central zone, ADC values appear to be lower in prostate cancer than in the central gland.
DCE MRI (see Figure 22-20) depicts the function of the tumor vasculature. The terminology for DCE-MRI is a bit unclear and, depending on the author, may represent a time-resolved enhancement pattern or a quantitative pharmacologic model–based assessment. Both benefit from rapid temporal resolution, thus, taking advantage of fast gradient echo sequences. In the former, some have espoused early enhancement and either early contrast wash-in or delayed contrast wash-out to suggest tumor,70 and sensitivity and specificity as high as 96% and 97% has been reported71; whereas, others prefer peak or relative peak enhancement.72 Overlap of enhancement patterns of cancer and normal or BPH in the central gland may be seen.71,73 In the latter, pharmacodynamic parameters such as Ktrans, kep, and ve may be extracted, depending on the pharmacodynamic model used. Some have advocated that Ktrans better than ve distinguishes prostate cancer from peripheral zone and central gland.74 Others have advocated ve.75 Time-resolved DCE-MRI does not appear to correlate with tumor stage73; and although it does not assist experienced readers in staging, it may benefit less experienced readers.76 Exact timing and parameters for dynamic imaging have not yet been standardized. DCE-MRI may be most useful in detecting recurrence after prostatectomy.
In addition to employing functional techniques, improvement in test characteristics may be afforded by higher field strength. 3-T magnets provide better signal-to-noise (SNR) and, thus, spatial, temporal, and spectroscopic resolution compared with 1.5-T systems and can be used with combined endorectal coils and phased array coils.77,78 Although susceptibility artifacts can increase at higher field strength, 3-T imaging should be beneficial because spatial resolution is important for all MRI of the prostate, SNR is important for diffusion imaging to improve signal and to reduce scan time, temporal resolution is important for DCE-MRI, and spectral resolution is important for MRSI. Miao and associates79 found that 3 T provides higher SNR and reduces scan time, thus motion artifacts, when combined with parallel imaging; this resulted in decreased distortion on DWI images compared with images on 1.5-T systems that suffer from poor SNR.
For lymph node evaluation, DWI may be helpful in identifying lymph nodes, but it is not specific for metastasis. MRI lymphography using ultrasmall superparamagnetic iron oxide (USPIO) contrast has shown potential in detecting and characterizing malignant nodes in patients with prostate carcinoma, with 90% sensitivity and 98% specificity reported in an early experience.80 However, these agents are not currently available clinically.
Use of 18F-fluoro-2-deoxy-D-glucose positron-emission tomography (FDG-PET) has been discouraging in detecting prostate cancer primarily because of the inherently low level of glucose metabolism by prostatic tumor cells and the physiologic excretion of 18F-FDG into the urinary bladder, which can mask tumor within or adjacent to the prostate.81 Promising preliminary results have been described with newer radiopharmaceuticals such as those based on choline, methionine, acetate, or L-leucine, and many are under active investigation.5,82–84
Treatment*
Prostate cancer is a relatively unique disease in that there are multiple treatment choices for localized tumors. The list of therapeutic options continues to grow, which is often a source of confusion and anxiety for patients. Treatment options include radical prostatectomy by the open or laparoscopic robotic approach, external beam radiation with photon or proton therapy, brachytherapy, cryotherapy, and high-intensity focused ultrasound on protocol. Hormone therapy may be indicated with external beam radiation, and appropriate patients are also considered for active surveillance. Adding to the dilemma is the evidence that disease-free survival is similar for the modalities with long-term follow-up and that the treatment choices can be associated with very different acute and long-term toxicity profiles and quality of life issues. Multiple consensus conferences have come to the conclusion that several good treatment options are available, but no “best option” can be agreed upon. Therefore, many patients see more than one physician for opinions, and under the best circumstances, patients are seen in a true multidisciplinary clinic where they see all the appropriate specialists in one setting and a joint, individualized opinion is rendered. For more locally advanced disease, a multidisciplinary approach involving multiple therapies, both local and systemic and often with surgical, radiation, and medical components, is key to obtaining the best outcomes. For metastatic disease, systemic treatment takes precedence.
Models have been created to predict treatment success. These combine DRE, serum PSA level, and Gleason score (D’Amico, Partin, or Kattan nomograms) and can significantly improve accuracy in predicting the risk of treatment failure compared with single-parameter assessment alone.9,85 Importantly, they provide general probabilities, not the specific risk, for an individual patient. Recently, the number of positive biopsy cores, the amount of cancer in each core, and the location of large-volume cores have been taken into consideration to improve the accuracy of local staging.86–88 Significantly, imaging can aide individualization of risk and treatment; for example, endorectal MRI contributes incrementally to the value of staging nomograms in predicting organ-confined prostate cancer.39,43
In locally advanced disease, T3 or beyond, systemic, combination therapy is employed. Neoadjuvant hormone therapy does not improve survival compared with surgery alone.89 In comparison, hormone therapy before, during, and after radiation improves 5-year survival compared with that from external beam radiation alone.88
For distant metastasis, hormonal therapy is used primarily. Metastatic prostate cancer tends to escape hormonal control at a median time of 13 to 21 months.90 In androgen-independent prostate cancer, rising PSA, or clinical progression in the setting of castration levels of testosterone, docetaxel-based regimens improve survival.91
Surgery
The primary goal of surgery is the complete removal of the prostate gland and associated periprostatic tissues with tumor-free margins, preservation of continence, and when indicated, preservation of erectile function. Unlike the majority of other cancer operations, surgical cure of prostate cancer is only one aspect of quality outcomes because the functional outcomes of continence and potency are critical components of a patient’s quality of life. The anatomic radical retropubic prostatectomy, as refined by Walsh and coworkers,92 has become the gold standard surgical approach. Nevertheless, the perioperative morbidity and sometimes less than satisfactory outcomes have motivated development of alternate approaches.93,94 With the evolution of laparoscopic techniques and the subsequent introduction of robotic surgical technology, robot-assisted radical prostatectomy (RARP) rapidly supplanted radical retropubic prostatectomy as the primary surgical treatment of prostate cancer in the first decade of 2000. Although the data on RARP are still evolving, in general, it has been shown to be consistently associated with shorter hospitalization, more rapid recovery, less blood loss, and a lower rate of strictures at the vesicourethral anastomosis.95,96 However, data confirming superiority for continence and erectile function are not mature and generally are not available from well-controlled studies except from a few single-institution studies.97 Although biopsy evidence of perineural invasion does not appear to curtail survival, it may indicate EPE7 and remains important when planning for nerve-sparing prostatectomy.
Radiation Therapy
External Beam Therapy
Methods for accurate tumor imaging and computer-based planning and treatment systems have been key to the necessary dose escalation and tumor targeting for prostate cancer radiation therapy. CT based three-dimensional treatment planning is now standard, as are sophisticated delivery techniques such as intensity-modulated radiation therapy (IMRT; Figure 22-21). For the latter, multiple beams, usually five to eight, are consecutively applied at different angles, and during each of these, a portion of the radiation field is blocked at a specified time. This enables intensity modulation and convergence to maximize dose to the target, but limits the dose to nearby critical structures such as the bladder, rectum, and bowel. CT and MRI in the preplanning stage can help identify such structures, including whether there may be small bowel in the field such as in a patient with an enterocoele. Computer-based planning allows calculation of radiation dose to each structure.
Outcome Studies for Primary Radiation Therapy
Several clinical trials have shown biochemical disease-free survival for patients receiving 74 to 79 Gy versus 64 to 70 Gy, including up to 10 years follow-up.98–103 Higher doses above 75.6 Gy (81 and 86.4 Gy) in studies with shorter follow-up did not further improve biochemical outcome in intermediate-risk patients.104 For high-risk patients, PSA disease-free outcome was better with 86.4 Gy but not when androgen-deprivation therapy was used. Benefit of ultrahigh doses must be proved in longer-term follow-up studies, especially because current data suggest slightly higher associated complication rates.105
Multiple randomized trials have shown improved biochemical and clinical outcome by adding hormone therapy (a luteinizing hormone–releasing hormone [LH-RH] agonist with or without an antiandrogen) to radiation for patients with intermediate- and high-risk prostate cancer.106–110 Not only is local control improved, but distant metastasis is decreased as well, leading to improved disease-specific and overall survival. Cardiac and metabolic complications of hormone therapy have led to the need for additional studies on decreasing the use and duration of androgen-deprivation therapy.111,112 Hormone therapy is not generally applied for low-risk prostate cancer because a recently reported randomized trial does not show additional benefit and PSA disease-free survival at 8 to 10 years after therapy is in the 90% range with high-dose radiation alone.98,104,113
Radioisotopic Implant
Although not as widely applicable as external beam radiation, radioisotopic implant has become a standard treatment option for patients with low-risk prostate cancer who have relatively small gland size and low American Urological Association (AUA) symptom scores. Long-term outcomes of permanent implants with iodine-125 and palladium-103 seeds are similar to prostatectomy or high-dose external beam therapy.114 Studies evaluating selected intermediate-risk patients without extracapsular extension or seminal vesicle involvement are under way. High dose-rate temporary iridium implants are also being explored.115
Hormone Therapy/Chemotherapy
If medical therapy is needed, it commonly begins with hormone therapy. This entails either reducing production of testosterone to castrated levels (e.g., by surgical orchiectomy or medical castration by administration of a gonadotropin-releasing hormone analogue) or blocking the effect of testosterone by inhibiting the testosterone receptor. Hormone therapy remains the primary treatment of metastatic prostate cancer. It also serves a role for treatment of intermediate-and high-risk disease that are treated with radiation, and based on data showing synergy and improved survival with multimodality therapy, hormone therapy may be given before, during, and after external beam radiation.116 Both hormone and radiation therapy result in shrinkage of the prostate gland and low signal on T2WI in the peripheral zone that can limit identification of prostate cancer (Figure 22-22). Functional techniques have the potential to allow evaluation of such a post therapy prostate gland. The use of hormone therapy before surgery has been investigated, and although it has been shown to improve surgical outcomes, such as lower positive margin rates, it does not appear to affect survival.117 Thus, efforts continue on finding the ideal neoadjuvant drug or combination drugs to use in such cases.118 More recently, there has been the recognition that hormone therapy, even for short courses of 1 to 2 years, can have deleterious systemic effects, and these are briefly reviewed in the section later on “Complications of Therapy.”
Inevitably and unpredictably, metastatic disease treated with hormone therapy becomes androgen-independent (or, conversely, hormone-resistant) prostate cancer, defined by either clinical or PSA progression in the face of a castrated level of testosterone. Until recently, such patients were relegated to palliative care only.119 Taxane-based therapies, however, have recently been shown to improve survival in this population and are now considered first-line therapy for androgen-independent prostate cancer. Skeletal-related events are a major source of morbidity in this population, but the introduction of bisphosphonates has lowered this risk.120 Efforts continue with newer agents as well as combination regimens in improving the outcomes of patients with androgen-independent prostate cancer.
Surveillance
Following radical prostatectomy, recurrence risk correlates with preoperative serum PSA, pathologic tumor stage, Gleason score, and positive surgical margins.121,122 Approximately 50% of patients with preoperative serum PSA greater than 10 ng/mL and 70% of patients with a Gleason score of 8 to 10 recur within 7 years after radical prostatectomy. Approximately 25% of patients with positive tumor margins recur within 5 years.121,123 After radical prostatectomy, any detectable PSA suggests recurrence. In comparison, after radiation therapy, PSA remains detectable at a low level; and in general, biochemical failure or recurrent disease is suspected when three consecutive increases in PSA are observed after the radiation therapy serum PSA nadir.123
When suspected, PSA kinetics can suggest the site of recurrence. In general, local recurrence is suggested by biochemical failure after a long duration post treatment (>24 mo after local treatment), low PSA velocity (change in serum PSA over time), and long PSA doubling time (>6 mo). In contrast, recurrence at a distant site is suggested by rapid biochemical failure, high PSA velocity, and short PSA doubling time.124,125
Thus, recurrent tumor after therapy may present as a distant metastasis or as a new mass in the prostatectomy bed. For the latter, MRI is superior to CT. TRUS, CT, FDG-PET, and DRE are limited in detecting local recurrence. ProstaScint and MRI have demonstrated encouraging initial results; however, they require further investigation.126 On MRI, the recurrent lesion may be hyperintense on T2 (instead of hypointense on T2 when the tumor is in the context of the prostate), isointense on T1, and enhances (Figure 22-23).127,128
If local recurrence is suspected, a biopsy is usually required for confirmation. However, an ultrasound-guided biopsy is unlikely to be of value unless the PSA is greater than 0.5 ng/mL, there is a palpable abnormality on DRE, or a target lesion is seen near the site of anastomosis after prostatectomy.129 Posttreatment changes can make histologic confirmation of recurrence difficult after radiation therapy.
After systemic therapy, lymph node metastasis may be pelvic, retroperitoneal, retrocrural, or rarely, mediastinal; but after pelvic radiation or lymph node dissection, metastasis to pelvic lymph nodes is less common and evaluation of nodes outside the treatment field is advisable.130 For the uncommon patient with a poor PSA-producing tumor, radiographic surveillance plays an even more important role. For identifying distant metastatic disease, bone scan, CT, or MRI is commonly employed.
Complications of Therapy
Immediately after prostatectomy, cystography may be used to evaluate for an anastomotic leak. Most leaks heal on their own. The most dreaded complication of prostatectomy is urinary incontinence, which is thankfully rare. Rates of complete incontinence in mature series and experienced hands are generally in the 1% to 2% range.93 However, it is not unusual for 10% to 30% of patients to experience stress urinary incontinence, with loss of minor urine volumes during periods of abdominal straining. Total incontinence is addressed by placement of an artifical urinary sphincter, and stress incontinence may be treated with various less extensive measures. Recuperation of erectile function depends primarily on three factors: the quality of preoperative erectile function, patient age (men < 60 have better recovery), and the quality and extent of nerve sparing.93 Complete erectile function recovery can take up to 2 years. A variety of treatments are available for treatment of postoperative erectile function, including use of oral phosphodiesterase inhibitors, vacuum erectile device, pharmacologic injection therapy, and placement of a prosthesis. The latter can be seen upon imaging. Urethral stricture disease is not uncommon after radical retropubic prostatectomy, ranging from 10% to 20% depending on the series, but is rare after RARP.131 Retrograde urethrogram may be performed to evaluate for such strictures.
Hormone therapy, particularly when sustained for over a year, is associated with several important systemic adverse outcomes. Commonly experienced side effects include hot flashes and lethargy; other complications may include anemia, muscle wasting, central obesity, increased rates of heart disease, loss of cognitive function, and osteoporosis with ensuing higher risk of fractures.132 In some patients, the complications may negatively affect overall survival to the point of potentially negating any benefit from treatment of the cancer; this has prompted the concept of reserving therapy or considering other treatments that might prolong the time to initiation of hormones.133 Patients treated with hormone therapy commonly have active intervention for some of the known adverse sequelae, such as bisphosphonates for osteoporosis.
New Therapies
Cryoablation, although not a new therapy for prostate cancer, has enjoyed a renewed interest based on new-generation machines that have improved outcomes and simplified the procedure.134 The ability to monitor the iceball by TRUS and the ability to localize the therapy has additionally spurred the development of focal cryotherapy of unilateral prostate cancer, but this is still considered investigational and is being evaluated at several centers.135
High-intensity focused ultrasound (HIFU), popular in Europe but not yet U.S. Food and Drug Administration (FDA) approved, is another relatively noninvasive new modality currently being evaluated.136 Questions remain about its long-term efficacy as well as complications. Nevertheless, HIFU holds promise as a low-morbidity therapy. Proton therapy is another form of external radiation that uses accelerated, positively charged particles. Compared with high-energy x-rays, with protons, there is a lower entrance dose as the beam enters tissue, followed by a peak of energy (Bragg peak), the depth of which is determined by the initial energy of the beam. At the distal edge of the peak, there is a sharp drop in energy, as compared with a more protracted dissipation and greater exit dose with an x-ray beam. Thus, proton therapy can be made very conformal around the tumor and fewer beams are usually necessary, allowing dose to tissues other than the prostate and urethra generally to be reduced.137,138 Although there is a paucity of outcome data for this new technique, Slater and colleagues139 reported biochemical outcome similar to x-ray therapy at similar dose levels. Quality of life studies are currently under way.
For patients with androgen-independent prostate cancer and androgen-sensitive disease at high risk for recurrence, studies are currently under way evaluating novel agents such as tyrosine kinase inhibitors, either alone or typically in combination with chemotherapy or hormone therapy.140
1. Jemal A., Murray T., Ward E., et al. Cancer statistics, 2005. CA Cancer J Clin. 2005;55:10-30.
2. Cancer Facts & Figures. Atlanta: American Cancer Society; 2003. 16–17
3. Brandt A., Bermejo J.L., Sundquist J., Hemminki K. Age at diagnosis and age at death in familial prostate cancer. Oncologist. 2009;14:1209-1217.
4. Kolonel L.N., Altshuler D., Henderson B.E. The multiethnic cohort study: exploring genes, lifestyle and cancer risk. Nat Rev Cancer. 2004;4:519-527.
5. McNeal J.E., Redwine E.A., Freiha F.S., Stamey T.A. Zonal distribution of prostatic adenocarcinoma. Correlation with histologic pattern and direction of spread. Am J Surg Pathol. 1988;12:897-906.
6. Cannon G.M.Jr., Pound C.R., Landsittel D.P., et al. Perineural invasion in prostate cancer biopsies is not associated with higher rates of positive surgical margins. Prostate. 2005;63:336-340.
7. O’Malley K.J., Pound C.R., Walsh P.C., et al. Influence of biopsy perineural invasion on long-term biochemical disease-free survival after radical prostatectomy. Urology. 2002;59:85-90.
8. Wheeler T.M. Anatomy of the prostate and the pathology of prostate cancer. In: Vogelzang N.J., Shipley W.U., Scardino P.T., Coffey D.S. Comprehensive Textbook of Genitourinary Oncology. Philadelphia: Lippincott Williams & Wilkins; 2000:587-604.
9. Presti J.C.Jr. Prostate cancer: assessment of risk using digital rectal examination, tumor grade, prostate-specific antigen, and systematic biopsy. Radiol Clin North Am. 2000;38:49-58.
10. Makarov D.V., Sanderson H., Partin A.W., Epstein J.I. Gleason score 7 prostate cancer on needle biopsy: is the prognostic difference in Gleason scores 4 + 3 and 3 + 4 independent of the number of involved cores? J Urol. 2002;167:2440-2442.
11. D’Amico A.V., Moul J.W., Kattan M.W. Emerging prognostic factors for outcome prediction in clinically localized prostate cancer: prostate-specific antigen level, race, molecular markers, and neural networks. In: Vogelzang N.J., Shipley W.U., Scardino P.T., Coffey D.S. Comprehensive Textbook of Genitourinary Oncology. Philadelphia: Lippincott Williams & Wilkins; 2000:680-700.
12. Noguchi M., Stamey T.A., McNeal J.E., Nolley R. Prognostic factors for multifocal prostate cancer in radical prostatectomy specimens: lack of significance of secondary cancers. J Urol. 2003;170:459-463.
13. Smith D.S., Catalona W.J. Interexaminer variability of digital rectal examination in detecting prostate cancer. Urology. 1995;45:70-74.
14. Whelan P. Multidisciplinary symposium: prostate cancer: planning primary therapy. Cancer Imaging. 2000;1:44-51.
15. Thompson I.M., Ankerst D.P., Chi C., et al. Operating characteristics of prostate-specific antigen in men with an initial PSA level of 3.0 ng/mL or lower. JAMA. 2005;294:66-70.
16. Arcangeli C.G., Ornstein D.K., Keetch D.W., Andriole G.L. Prostate-specific antigen as a screening test for prostate cancer. The United States experience. Urol Clin North Am. 1997;24:299-306.
17. Thompson I.M., Pauler D.K., Goodman P.J., et al. Prevalence of prostate cancer among men with a prostate-specific antigen level ≤ 4.0 ng per milliliter. N Engl J Med. 2004;350:2239-2246.
18. Catalona W.J., Smith D.S. 5-year tumor recurrence rates after anatomical radical retropubic prostatectomy for prostate cancer. J Urol. 1994;152:1837-1842.
19. Bill-Axelson A., Holmberg L., Ruutu M., et al. Radical prostatectomy versus watchful waiting in early prostate cancer. N Engl J Med. 2005;352:1977-1984.
20. Etzioni R., Penson D.F., Legler J.M., et al. Overdiagnosis due to prostate-specific antigen screening: lessons from U.S. prostate cancer incidence trends. J Natl Cancer Inst. 2002;94:981-990.
21. Welch H.G., Albertsen P.C. Prostate cancer diagnosis and treatment after the introduction of prostate-specific antigen screening: 1986-2005. J Natl Cancer Inst. 2009;101:1325-1329.
22. Gretzer M.B., Partin A.W. PSA markers in prostate cancer detection. Urol Clin North Am. 2003;30:677-686.
23. Chun F.K., de la Taille A., van Poppel H., et al. Prostate cancer gene 3 (PCA3): development and internal validation of a novel biopsy nomogram. Eur Urol. 2009;56:659-667.
24. Jackson A.S., Sohaib S.A., Staffurth J.N., et al. Distribution of lymph nodes in men with prostatic adenocarcinoma and lymphadenopathy at presentation: a retrospective radiological review and implications for prostate and pelvis radiotherapy. Clin Oncol (R Coll Radiol). 2006;18:109-116.
25. AJCC. Prostate. In: Greene F.L., American Joint Committee on Cancer, American Cancer Society. AJCC Cancer Staging Manual. New York: Springer-Verlag; 2002:337-345.
26. Yu K.K., Hawkins R.A. The prostate: diagnostic evaluation of metastatic disease. Radiol Clin North Am. 2000;38:139-157. ix
27. Spencer J., Golding S. CT evaluation of lymph node status at presentation of prostatic carcinoma. Br J Radiol. 1992;65:199-201.
28. Saitoh H., Yoshida K., Uchijima Y., et al. Two different lymph node metastatic patterns of a prostatic cancer. Cancer. 1990;65:1843-1846.
29. Bubendorf L., Schopfer A., Wagner U., et al. Metastatic patterns of prostate cancer: an autopsy study of 1,589 patients. Hum Pathol. 2000;31:578-583.
30. Keetch D.W., Catalona W.J., Smith D.S. Serial prostatic biopsies in men with persistently elevated serum prostate specific antigen values. J Urol. 1994;151:1571-1574.
31. Roehrborn C.G., Pickens G.J., Sanders J.S. Diagnostic yield of repeated transrectal ultrasound-guided biopsies stratified by specific histopathologic diagnoses and prostate specific antigen levels. Urology. 1996;47:347-352.
32. Ellis W.J., Brawer M.K. Repeat prostate needle biopsy: who needs it? J Urol. 1995;153:1496-1498.
33. Presti J.C.Jr., Chang J.J., Bhargava V., Shinohara K. The optimal systematic prostate biopsy scheme should include 8 rather than 6 biopsies: results of a prospective clinical trial. J Urol. 2000;163:163-166. discussion 166–167
34. Singh H., Canto E.I., Shariat S.F., et al. Improved detection of clinically significant, curable prostate cancer with systematic 12-core biopsy. J Urol. 2004;171:1089-1092.
35. Lee F., Torp-Pedersen S., Littrup P.J., et al. Hypoechoic lesions of the prostate: clinical relevance of tumor size, digital rectal examination, and prostate-specific antigen. Radiology. 1989;170:29-32.
36. Littrup P.J., Bailey S.E. Prostate cancer: the role of transrectal ultrasound and its impact on cancer detection and management. Radiol Clin North Am. 2000;38:87-113.
37. Kuligowska E., Barish M.A., Fenlon H.M., Blake M. Predictors of prostate carcinoma: accuracy of gray-scale and color Doppler US and serum markers. Radiology. 2001;220:757-764.
38. Halpern E.J., Rosenberg M., Gomella L.G. Prostate cancer: contrast-enhanced us for detection. Radiology. 2001;219:219-225.
39. Sanchez-Chapado M., Angulo J.C., Ibarburen C., et al. Comparison of digital rectal examination, transrectal ultrasonography, and multicoil magnetic resonance imaging for preoperative evaluation of prostate cancer. Eur Urol. 1997;32:140-149.
40. Ekici S., Ozen H., Agildere M., et al. A comparison of transrectal ultrasonography and endorectal magnetic resonance imaging in the local staging of prostatic carcinoma. BJU Int. 1999;83:796-800.
41. D’Amico A.V., Whittington R., Malkowicz S.B., et al. Role of percent positive biopsies and endorectal coil MRI in predicting prognosis in intermediate-risk prostate cancer patients. Cancer J Sci Am. 1996;2:343.
42. Perrotti M., Kaufman R.P.Jr., Jennings T.A., et al. Endo-rectal coil magnetic resonance imaging in clinically localized prostate cancer: is it accurate? J Urol. 1996;156:106-109.
43. Wang L., Hricak H., Kattan M.W., et al. Prediction of organ-confined prostate cancer: incremental value of MR imaging and MR spectroscopic imaging to staging nomograms. Radiology. 2006;238:597-603.
44. Comet-Batlle J., Vilanova-Busquets J.C., Saladie-Roig J.M., et al. The value of endorectal MRI in the early diagnosis of prostate cancer. Eur Urol. 2003;44:201-207. discussion 207–208
45. Swindle P., McCredie S., Russell P., et al. Pathologic characterization of human prostate tissue with proton MR spectroscopy. Radiology. 2003;228:144-151.
46. Scheidler J., Hricak H., Vigneron D.B., et al. Prostate cancer: localization with three-dimensional proton MR spectroscopic imaging—clinicopathologic study. Radiology. 1999;213:473-480.
47. Wefer A.E., Hricak H., Vigneron D.B., et al. Sextant localization of prostate cancer: comparison of sextant biopsy, magnetic resonance imaging and magnetic resonance spectroscopic imaging with step section histology. J Urol. 2000;164:400-404.
48. Kurhanewicz J., Vigneron D.B., Males R.G., et al. The prostate: MR imaging and spectroscopy. Present and future. Radiol Clin North Am. 2000;38:115-138. viii-ix
49. Jager G.J., Severens J.L., Thornbury J.R., et al. Prostate cancer staging: should MR imaging be used?—A decision analytic approach. Radiology. 2000;215:445-451.
50. D’Amico A.V., Whittington R., Malkowicz S.B., et al. Combined modality staging of prostate carcinoma and its utility in predicting pathologic stage and postoperative prostate specific antigen failure. Urology. 1997;49:23-30.
51. Barentsz J.O., Jager G.J., Engelbrecht M.R. MR imaging of prostate cancer. Cancer Imaging. 2000;1:44-51.
52. Langlotz C., Schnall M., Pollack H. Staging of prostatic cancer: accuracy of MR imaging. Radiology. 1995;194:645-646. discussion 647–648
53. Jager G.J., Ruijter E.T., van de Kaa C.A., et al. Local staging of prostate cancer with endorectal MR imaging: correlation with histopathology. AJR Am J Roentgenol. 1996;166:845-852.
54. Cheng D., Tempany C.M. MR imaging of the prostate and bladder. Semin Ultrasound CT MR. 1998;19:67-89.
55. Cheng L., Darson M.F., Bergstralh E.J., et al. Correlation of margin status and extraprostatic extension with progression of prostate carcinoma. Cancer. 1999;86:1775-1782.
56. Haukaas S., Roervik J., Halvorsen O.J., Foelling M. When is bone scintigraphy necessary in the assessment of newly diagnosed, untreated prostate cancer? Br J Urol. 1997;79:770-776.
57. Gold R.I., Seeger L.L., Bassett L.W., Steckel R.J. An integrated approach to the evaluation of metastatic bone disease. Radiol Clin North Am. 1990;28:471-483.
58. Algra P.R., Bloem J.L., Tissing H., et al. Detection of vertebral metastases: comparison between MR imaging and bone scintigraphy. Radiographics. 1991;11:219-232.
59. Manyak M.J., Hinkle G.H., Olsen J.O., et al. Immunoscintigraphy with indium-111-capromab pendetide: evaluation before definitive therapy in patients with prostate cancer. Urology. 1999;54:1058-1063.
60. Ponsky L.E., Cherullo E.E., Starkey R., et al. Evaluation of preoperative ProstaScint scans in the prediction of nodal disease. Prostate Cancer Prostatic Dis. 2002;5:132-135.
61. Ellis R.J., Kim E.Y., Conant R., et al. Radioimmunoguided imaging of prostate cancer foci with histopathological correlation. Int J Radiat Oncol Biol Phys. 2001;49:1281-1286.
62. Yao D., Trabulsi E.J., Kostakoglu L., et al. The utility of monoclonal antibodies in the imaging of prostate cancer. Semin Urol Oncol. 2002;20:211-218.
63. Costouros N.G., Coakley F.V., Westphalen A.C., et al. Diagnosis of prostate cancer in patients with an elevated prostate-specific antigen level: role of endorectal MRI and MR spectroscopic imaging. AJR Am J Roentgenol. 2007;188:812-816.
64. Wetter A., Engl T.A., Nadjmabadi D., et al. Combined MRI and MR spectroscopy of the prostate before radical prostatectomy. AJR Am J Roentgenol. 2006;187:724-730.
65. Zakian K.L., Eberhardt S., Hricak H., et al. Transition zone prostate cancer: metabolic characteristics at 1H MR spectroscopic imaging–initial results. Radiology. 2003;229:241-247.
66. Pickett B., Kurhanewicz J., Coakley F., et al. Use of MRI and spectroscopy in evaluation of external beam radiotherapy for prostate cancer. Int J Radiat Oncol Biol Phys. 2004;60:1047-1055.
67. Mueller-Lisse U.G., Vigneron D.B., Hricak H., et al. Localized prostate cancer: effect of hormone deprivation therapy measured by using combined three-dimensional 1H MR spectroscopy and MR imaging: clinicopathologic case-controlled study. Radiology. 2001;221:380-390.
68. Reinsberg S.A., Payne G.S., Riches S.F., et al. Combined use of diffusion-weighted MRI and 1H MR spectroscopy to increase accuracy in prostate cancer detection. AJR Am J Roentgenol. 2007;188:91-98.
69. Pickles M.D., Gibbs P., Sreenivas M., Turnbull L.W. Diffusion-weighted imaging of normal and malignant prostate tissue at 3.0T. J Magn Reson Imaging. 2006;23:130-134.
70. Tanimoto A., Nakashima J., Kohno H., et al. Prostate cancer screening: the clinical value of diffusion-weighted imaging and dynamic MR imaging in combination with T2-weighted imaging. J Magn Reson Imaging. 2007;25:146-152.
71. Kim J.K., Hong S.S., Choi Y.J., et al. Wash-in rate on the basis of dynamic contrast-enhanced MRI: usefulness for prostate cancer detection and localization. J Magn Reson Imaging. 2005;22:639-646.
72. Engelbrecht M.R., Huisman H.J., Laheij R.J., et al. Discrimination of prostate cancer from normal peripheral zone and central gland tissue by using dynamic contrast-enhanced MR imaging. Radiology. 2003;229:248-254.
73. Padhani A.R., Gapinski C.J., Macvicar D.A., et al. Dynamic contrast enhanced MRI of prostate cancer: correlation with morphology and tumour stage, histological grade and PSA. Clin Radiol. 2000;55:99-109.
74. Kozlowski P., Chang S.D., Jones E.C., et al. Combined diffusion-weighted and dynamic contrast-enhanced MRI for prostate cancer diagnosis—correlation with biopsy and histopathology. J Magn Reson Imaging. 2006;24:108-113.
75. Futterer J.J., Heijmink S.W., Scheenen T.W., et al. Prostate cancer localization with dynamic contrast-enhanced MR imaging and proton MR spectroscopic imaging. Radiology. 2006;241:449-458.
76. Futterer J.J., Engelbrecht M.R., Huisman H.J., et al. Staging prostate cancer with dynamic contrast-enhanced endorectal MR imaging prior to radical prostatectomy: experienced versus less experienced readers. Radiology. 2005;237:541-549.
77. Futterer J.J., Scheenen T.W., Huisman H.J., et al. Initial experience of 3 tesla endorectal coil magnetic resonance imaging and 1H-spectroscopic imaging of the prostate. Invest Radiol. 2004;39:671-680.
78. Bloch B.N., Rofsky N.M., Baroni R.H., et al. 3 Tesla magnetic resonance imaging of the prostate with combined pelvic phased-array and endorectal coils: initial experience(1). Acad Radiol. 2004;11:863-867.
79. Miao H., Fukatsu H., Ishigaki T. Prostate cancer detection with 3-T MRI: comparison of diffusion-weighted and T2-weighted imaging. Eur J Radiol. 2007;61:297-302.
80. Harisinghani M.G., Barentsz J., Hahn P.F., et al. Noninvasive detection of clinically occult lymph-node metastases in prostate cancer. N Engl J Med. 2003;348:2491-2499.
81. Shvarts O., Han K.R., Seltzer M., et al. Positron emission tomography in urologic oncology. Cancer Control. 2002;9:335-342.
82. Hara T., Kosaka N., Kishi H. PET imaging of prostate cancer using carbon-11-choline. J Nucl Med. 1998;39:990-995.
83. Nunez R., Macapinlac H.A., Yeung H.W., et al. Combined 18F-FDG and 11C-methionine PET scans in patients with newly progressive metastatic prostate cancer. J Nucl Med. 2002;43:46-55.
84. Schuster D.M., Votaw J.R., Nieh P.T., et al. Initial experience with the radiotracer anti-1-amino-3-18F-fluorocyclobutane-1-carboxylic acid with PET/CT in prostate carcinoma. J Nucl Med. 2007;48:56-63.
85. Hittelman A.B., Purohit R.S., Kane C.J. Update of staging and risk assessment for prostate cancer patients. Curr Opin Urol. 2004;14:163-170.
86. Kamat A.M., Jacobsohn K.M., Troncoso P., et al. Validation of criteria used to predict extraprostatic cancer extension: a tool for use in selecting patients for nerve sparing radical prostatectomy. J Urol. 2005;174:1262-1265.
87. Vira M.A., Tomaszewski J.E., Hwang W.T., et al. Impact of the percentage of positive biopsy cores on the further stratification of primary grade 3 and grade 4 Gleason score 7 tumors in radical prostatectomy patients. Urology. 2005;66:1015-1019.
88. D’Amico A.V., Renshaw A.A., Cote K., et al. Impact of the percentage of positive prostate cores on prostate cancer-specific mortality for patients with low or favorable intermediate-risk disease. J Clin Oncol. 2004;22:3726-3732.
89. Klotz L.H., Goldenberg S.L., Jewett M.A., et al. Long-term followup of a randomized trial of 0 versus 3 months of neoadjuvant androgen ablation before radical prostatectomy. J Urol. 2003;170:791-794.
90. So A., Gleave M., Hurtado-Col A., Nelson C. Mechanisms of the development of androgen independence in prostate cancer. World J Urol. 2005;23:1-9.
91. Oudard S., Banu E., Beuzeboc P., et al. Multicenter randomized phase II study of two schedules of docetaxel, estramustine, and prednisone versus mitoxantrone plus prednisone in patients with metastatic hormone-refractory prostate cancer. J Clin Oncol. 2005;23:3343-3351.
92. Walsh P.C., Partin A.W., Epstein J.I. Cancer control and quality of life following anatomical radical retropubic prostatectomy: results at 10 years [see comments]. J Urol. 1994;152:1831-1836.
93. Kundu S.D., Roehl K.A., Eggener S.E., et al. Potency, continence and complications in 3,477 consecutive radical retropubic prostatectomies. J Urol. 2004;172:2227-2231.
94. Nuttall M., van der Meulen J.A.N., Phillips N., et al. A systematic review and critique of the literature relating hospital or surgeon volume to health outcomes for 3 urological cancer procedures. J Urol. 2004;172:2145-2152.
95. Zorn K.C., Gofrit O.N., Steinberg G.D., Shalhav A.L. Evolution of robotic surgery in the treatment of localized prostate cancer. Curr Treatment Options Oncol. 2007;8:197-210.
96. Hu J.C., Wang Q., Pashos C.L., et al. Utilization and outcomes of minimally invasive radical prostatectomy. J Clin Oncol. 2008;26:2278-2284.
97. Krambeck A.E., DiMarco D.S., Rangel L.J., et al. Radical prostatectomy for prostatic adenocarcinoma: a matched comparison of open retropubic and robot-assisted techniques. BJU Int. 2009;103:448-453.
98. Kuban D.A., Tucker S.L., Dong L., et al. Long-term results of the M. D. Anderson randomized dose-escalation trial for prostate cancer. Int J Radiat Oncol Biol Phys. 2008;70:67-74.
99. Zietman A.L., DeSilvio M.L., Slater J.D., et al. Comparison of conventional-dose vs high-dose conformal radiation therapy in clinically localized adenocarcinoma of the prostate: a randomized controlled trial. JAMA. 2005;294:1233-1239.
100. Zietman A.L. Correction: inaccurate analysis and results in a study of radiation therapy in adenocarcinoma of the prostate. JAMA. 2008;299:898-899.
101. Al-Mamgani A., van Putten W.L., Heemsbergen W.D., et al. Update of Dutch multicenter dose-escalation trial of radiotherapy for localized prostate cancer. Int J Radiat Oncol Biol Phys. 2008;72:980-988.
102. Dearnaley D.P., Sydes M.R., Graham J.D., et al. Escalated-dose versus standard-dose conformal radiotherapy in prostate cancer: first results from the MRC RT01 randomised controlled trial. Lancet Oncol. 2007;8:475-487.
103. Kuban D.A., Levy L.B., Tucker S., et al. Long-term failure patterns and survival in a randomized dose-escalation trial for prostate cancer. Who dies of disease? Int J Radiat Oncol Biol Phys. 2008;72:S93.
104. Zelefsky M.J., Yamada Y., Fuks Z., et al. Long-term results of conformal radiotherapy for prostate cancer: impact of dose escalation on biochemical tumor control and distant metastases-free survival outcomes. Int J Radiat Oncol Biol Phys. 2008;71:1028-1033.
105. Cahlon O., Zelefsky M.J., Shippy A., et al. Ultra-high dose (86.4 Gy) IMRT for localized prostate cancer: toxicity and biochemical outcomes. Int J Radiat Oncol Biol Phys. 2008;71:330-337.
106. Pilepich M.V., Winter K., Lawton C.A., et al. Androgen suppression adjuvant to definitive radiotherapy in prostate carcinoma—long-term results of phase III RTOG 85-31. Int J Radiat Oncol Biol Phys. 2005;61:1285-1290.
107. Bolla M., Collette L., Blank L., et al. Long-term results with immediate androgen suppression and external irradiation in patients with locally advanced prostate cancer (an EORTC study): a phase III randomised trial. Lancet. 2002;360:103-106.
108. Horwitz E.M., Bae K., Hanks G.E., et al. Ten-year follow-up of radiation therapy oncology group protocol 92-02: a phase III trial of the duration of elective androgen deprivation in locally advanced prostate cancer. J Clin Oncol. 2008;26:2497-2504.
109. D’Amico A.V., Chen M.H., Renshaw A.A., et al. Androgen suppression and radiation vs radiation alone for prostate cancer: a randomized trial. JAMA. 2008;299:289-295.
110. Denham J.W., Steigler A., Lamb D.S., et al. Short-term androgen deprivation and radiotherapy for locally advanced prostate cancer: results from the Trans-Tasman Radiation Oncology Group 96.01 randomised controlled trial. Lancet Oncol. 2005;6:841-850.
111. Keating N.L., O’Malley A.J., Smith M.R. Diabetes and cardiovascular disease during androgen deprivation therapy for prostate cancer. J Clin Oncol. 2006;24:4448-4456.
112. D’Amico A.V., Denham J.W., Crook J., et al. Influence of androgen suppression therapy for prostate cancer on the frequency and timing of fatal myocardial infarctions. J Clin Oncol. 2007;25:2420-2425.
113. Jones CU, Hunt D, McGowan DG, et al. Radiotherapy and short-term androgen deprivation for localized prostate cancer. N Engl J Med. 2011;365:107-118.
114. Potters L., Morgenstern C., Calugaru E., et al. 12-year outcomes following permanent prostate brachytherapy in patients with clinically localized prostate cancer. J Urol. 2005;173:1562-1566.
115. Grills I.S., Martinez A.A., Hollander M., et al. High dose rate brachytherapy as prostate cancer monotherapy reduces toxicity compared to low dose rate palladium seeds. J Urol. 2004;171:1098-1104.
116. Lee A.K. Radiation therapy combined with hormone therapy for prostate cancer. Semin Radiat Oncol. 2006;16:20-28.
117. Soloway M.S., Pareek K., Sharifi R., et al. Neoadjuvant androgen ablation before radical prostatectomy in cT2bNxMo prostate cancer: 5-year results. J Urol. 2002;167:112-116.
118. Pettaway C.A., Pisters L.L., Troncoso P., et al. Neoadjuvant chemotherapy and hormonal therapy followed by radical prostatectomy: feasibility and preliminary results. J Clin Oncol. 2000;18:1050-1057.
119. Tannock I.F., Osoba D., Stockler M.R., et al. Chemotherapy with mitoxantrone plus prednisone or prednisone alone for symptomatic hormone-resistant prostate cancer: a Canadian randomized trial with palliative end points [see comments]. J Clin Oncol. 1996;14:1756-1764.
120. Pinski J., Dorff T.B. Prostate cancer metastases to bone: pathophysiology, pain management, and the promise of targeted therapy. Eur J Cancer. 2005;41:932-940.
121. Pound C.R., Partin A.W., Eisenberger M.A., et al. Natural history of progression after PSA elevation following radical prostatectomy. JAMA. 1999;281:1591-1597.
122. Han M., Partin A.W., Pound C.R., et al. Long-term biochemical disease-free and cancer-specific survival following anatomic radical retropubic prostatectomy. The 15-year Johns Hopkins experience. Urol Clin North Am. 2001;28:555-565.
123. Horwitz E.M., Vicini F.A., Ziaja E.L., et al. The correlation between the ASTRO Consensus Panel definition of biochemical failure and clinical outcome for patients with prostate cancer treated with external beam irradiation. American Society of Therapeutic Radiology and Oncology. Int J Radiat Oncol Biol Phys. 1998;41:267-272.
124. Partin A.W., Pearson J.D., Landis P.K., et al. Evaluation of serum prostate-specific antigen velocity after radical prostatectomy to distinguish local recurrence from distant metastases. Urology. 1994;43:649-659.
125. Lee A.K., D’Amico A.V. Utility of prostate-specific antigen kinetics in addition to clinical factors in the selection of patients for salvage local therapy. J Clin Oncol. 2005;23:8192-8197.
126. Swindle P.W., Kattan M.W., Scardino P.T. Markers and meaning of primary treatment failure. Urol Clin North Am. 2003;30:377-401.
127. Manzone T.A., Malkowicz S.B., Tomaszewski J.E., et al. Use of endorectal MR imaging to predict prostate carcinoma recurrence after radical prostatectomy. Radiology. 1998;209:537-542.
128. Silverman J.M., Krebs T.L. MR imaging evaluation with a transrectal surface coil of local recurrence of prostatic cancer in men who have undergone radical prostatectomy. AJR Am J Roentgenol. 1997;168:379-385.
129. Naya Y., Okihara K., Evans R.B., Babaian R.J. Efficacy of prostatic fossa biopsy in detecting local recurrence after radical prostatectomy. Urology. 2005;66:350-355.
130. Spencer J.A., Golding S.J. Patterns of lymphatic metastases at recurrence of prostate cancer: CT findings. Clin Radiol. 1994;49:404-407.
131. Webb D.R., Sethi K., Gee K. An analysis of the causes of bladder neck contracture after open and robot-assisted laparoscopic radical prostatectomy. BJU Int. 2009;103:957-963.
132. Kumar R.J., Barqawi A., Crawford E.D. Preventing and treating the complications of hormone therapy. Curr Urol Rep. 2005;6:217-223.
133. Gomella L.G. Contemporary use of hormonal therapy in prostate cancer: managing complications and addressing quality-of-life issues. BJU Int. 2007;99(suppl 1):25-29. discussion 30
134. Bermejo C.E., Pisters L.L. Cryotherapy for prostate cancer. Exp Rev Anticancer Ther. 2003;3:393-401.
135. Ward J.F., Nakanishi H., Pisters L., et al. Cancer ablation with regional templates applied to prostatectomy specimens from men who were eligible for focal therapy. BJU Int. 2009;104:490-497.
136. Blana A., Brown S.C., Chaussy C., et al. High-intensity focused ultrasound for prostate cancer: comparative definitions of biochemical failure. BJU Int. 2009;104:1058-1062.
137. Lee A., Levy L., Dong L., et al. IMRT for prostate cancer: the M.D. Anderson experience. Oncology. 2008;22:13. (abstract #S31)
138. Trofimov A., Nguyen P.L., Coen J.J., et al. Radiotherapy treatment of early-stage prostate cancer with IMRT and protons: a treatment planning comparison. Int J Radiat Oncol Biol Phys. 2007;69:444-453.
139. Slater J.D., Rossi C.J.Jr., Yonemoto L.T., et al. Proton therapy for prostate cancer: the initial Loma Linda University experience. Int J Radiat Oncol Biol Phys. 2004;59:348-352.
140. M. Dror Michaelson, M.M. Regan, W.K. Oh. et al. Phase II study of sunitinib in men with advanced prostate cancer. Ann Oncol.. 2009;20:913-920.