Chapter 16 Prognostic Implications of MPI Stress SPECT
INTRODUCTION
At a time when other modalities—specifically cardiac computed tomography (CT), cardiac magnetic resonance (CMR), and positron emission tomography (PET)—are increasingly being used in the assessment and management of patients with known or suspected coronary artery disease (CAD),1 the question of why stress myocardial perfusion single-photon emission computed tomography (SPECT), or MPS, continues to be the most commonly utilized of these modalities must be asked. Cardiac CT and CMR have superior resolution, hence can successfully image coronary arteries, left ventricular size, shape, and wall thickness, as well as valves, pericardium, and other clinically relevant structures. Further, coronary CT angiography (CCTA) has the promise of imaging atherosclerotic plaque burden, morphology, and composition. In this era of newer, advanced modalities, will MPS still have a home?
PRINCIPLES OF RISK STRATIFICATION: PATIENT SELECTION AND METRICS OF RISK
Several concepts define the basis of risk stratification after stress imaging; generally speaking, these principals hold true for all testing modalities. First, with respect to appropriate selection of patients for testing, the basic concept underlying the use of nuclear testing for risk stratification is that only those patients who can be successfully further stratified (or restratified) in a cost-effective manner would be appropriate patients for stress MPS.2 While MPS has been shown to successfully risk stratify multiple, diverse populations,3 it is cost-effective only when applied to intermediate and high-risk patients,3–6 and its use should be limited to these populations.7
Practically, optimal risk stratification is based on the hypothesis that the risk associated with a normal stress imaging study is sufficiently low that aggressive CAD management and therapeutics will not further improve patient outcomes.3,8,9 Hence, for example, it is commonly held that invasive coronary angiography and coronary interventions are less frequently performed in patients with normal stress imaging studies than in symptomatic patients in whom these studies are not performed.6
On the other hand, patients with abnormal stress imaging results are at greater risk of adverse events, thus resulting in risk stratification in its most basic form relative to normal MPS results (Fig. 16-1). Also, these patients are potential candidates for intervention,10–12 and the magnitude of their risk is related to the extent and severity of the imaging abnormalities. Based on this premise, outcomes data from an imaging modality should initially be examined for two patterns: (1) risk of adverse events after a normal study and (2) relationship between risk and increasing test abnormality.2
To date, examination of MPS test performance has focused on significant clinical events as endpoints—all cause death, cardiac death, and nonfatal myocardial infarction. In this chapter, we will focus on studies evaluating the association of MPS with major events. For purposes of risk assessment, it has been proposed that low risk be defined as a less than 1% annual cardiac mortality rate, intermediate risk defined by the range of 1% to 3% per year, and high risk as greater than 3% per year.13 It is likely that in the future, studies will increasingly use endpoints related to resource utilization (hospitalization of cardiac causes, emergency department visits, referral to downstream interventions and procedures) to better capture the association of MPS with the global cardiac outcomes, thus better defining its role in patient care.2
The use of metrics and thresholds to define the success of risk stratification by testing is challenging. Historically in nuclear cardiology, risk stratification was considered successful if patients with normal scans had either a hard event rate or a mortality rate (varying with publication) of less than 1% per year of follow-up, while patients with abnormal scans have rates exceeding 1%. As will be discussed, the use of thresholding to define levels of risk is problematic. The effectiveness of stratification may also be judged by the ratio of risk in patients with abnormal, compared to those with normal, scans (as measured by a relative risk or odds ratio); increasing relative risks indicate increasing effectiveness in risk stratification.2
Risk of Adverse Events After a Normal Imaging Study
To date, there is extensive literature that supports the concept that a normal stress SPECT study is associated with a low risk of hard events (cardiac death or nonfatal myocardial infarction). A pooled analysis from 19 series in the literature comprising 39,173 patients with normal stress SPECT studies, followed for an average of 2.3 years, showed an annual death or myocardial infarction rate of 0.6%.8 Further, an American Society of Nuclear Cardiology position statement on normal SPECT results reported the very low likelihood (<1%) of adverse events such as cardiac death or myocardial infarction for at least 12 months, independent of gender, age, symptom status, past history of CAD, presence of anatomic CAD, imaging technique, or isotope.14
A closer scrutiny of the published literature reveals inconsistency in the message of the statements in the previous paragraph. In general, these studies have suggested that this low risk is independent of imaging type (SPECT versus planar), the type of stress performed (exercise versus pharmacologic), the radiopharmaceutical used, patients’ clinical characteristics, patients’ prior history of CAD, the results of stress testing, as well as many other factors. However, studies in patients undergoing pharmacologic stress, a population at higher risk and with more comorbidities than patients undergoing exercise stress, have reported hard event rates of 1.3% to 2.7% per year, suggesting that underlying clinical risk and previous CAD may influence event rates after a normal MPS.15–21
These studies encompass cohorts undergoing dipyridamole stress,15 patients aged 70 years or older,22 [Hachamovitch, 2003 #11] patients with stable chest pain undergoing dipyridamole stress,18 patients with diabetes mellitus undergoing adenosine stress,19,21,19–21 and patients undergoing dobutamine stress.17 This paradigm is particularly challenged with diabetic patients. Diabetic patients have been found to have strikingly higher event rates after normal MPS,23 with a number of studies reporting annual hard event rates of 2.0% or more. Interestingly, the event rates in diabetics after stress echocardiography are even greater.24
The issue of variability in risk after a normal MPS and the temporal characteristics of this risk (e.g., its “warranty” period) was addressed by a series of 7376 patients with normal stress MPS.21 This study identified a number of variables: the use of pharmacologic stress, the presence of known CAD, diabetes mellitus (in particular, female diabetics), and advanced age as markers of increased risk and shortened time to risk (e.g., risk in the first year of follow-up was less than in the second year). This study attributed the increased risk after normal MPS in a small subset of patients to the presence of comorbidities that increased the baseline risk of these patients (diabetes mellitus, age, inability to exercise, previous CAD). The more of these characteristics present, the greater the risk after a normal MPS test (Figs. 16-2 and 16-3).
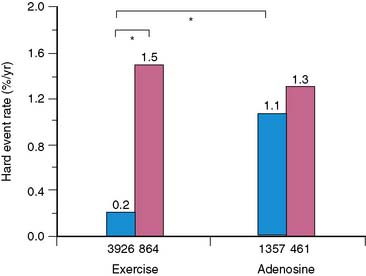
Figure 16-2 Hard event rates (% per year) in patients without history of known coronary artery disease (blue bars) versus with history of known coronary artery disease (pink bars) undergoing exercise (left) or adenosine (right) stress. Numbers under bars represent number of patients within category.21 *P < 0.001.
A review by Kalamesh et al.24 addresses the issue of event rates exceeding the threshold of 1% risk per year in specific patient subsets and posed the question of whether it is a failure of the test or a characteristic of the patient. If it is a failure of the test, the implication would be that the test should not be relied on in certain patient subsets (e.g., diabetic patients with suspected CAD should not go to MPS). If the higher event rates after a normal study result from a characteristic of the patient, then it becomes important to set aside generalized thresholds and define what patient-specific event rates are acceptable after a normal study. An alternative answer is that the failure is of the paradigm of defining risk by a single threshold. Given the diversity of patients presenting for evaluation for risk of cardiac events and their wide pretest range of risk of adverse events, the definition of low risk after testing (i.e., the posttest risk) needs to take into account the patient’s pretest risk as well as the characteristics of the test, similar to the methods applied to calculate the pretest and posttest likelihood of CAD. Thus, although normal MPS results are associated with low absolute risk in most patient cohorts, care must be taken in assessing post-MPS risk in patients with comorbidities and risk factors.
Relationship Between Risk and the Extent and Severity of Imaging Results
In general, it is safe to say that a close relationship exists between the extent and severity of perfusion abnormalities on stress MPS and subsequent risk of adverse outcomes (Fig. 16-4).11,12,25 Several characteristics of abnormal studies are worth highlighting. First, even after first stratifying a cohort by their pre-MPS risk, MPS results will still achieve further risk stratification in all levels of pre-MPS risk (Figs. 16-5 and 16-6).2,12 This pattern of results can be considered to be a demonstration of clinical incremental prognostic value.12 In a similar relationship, nonperfusion SPECT imaging variables such as transient ischemic dilation of the left ventricle and variables reflecting regional and global LV function add useful prognostic information to sole assessment of extent and severity of perfusion defects.3 Further, in patients undergoing vasodilator stress, the presence of a lower baseline heart rate and a greater peak heart rate were both associated with decreased risk (Fig. 16-7).26
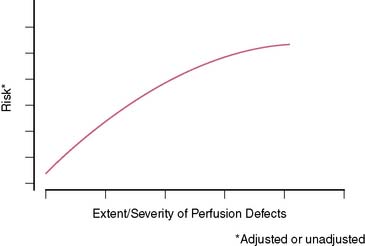
Figure 16-4 A generalized schematic representing the relationship between the extent and severity of MPS defects and post-MPS risk. The shape of this curve (flattening of the curve at high levels of defect extent and severity) is related to the use of revascularization in higher-risk patients, thus reducing the observed risk in MPS populations.2,5 This curve is shifted up or down (change in risk for any MPS result) by the patients’ baseline (pre-MPS) risk.
(From Hachamovitch R, Di Carli MF: Contemporary reviews in cardiovascular medicine: Methods and limitations of assessing new noninvasive tests II. Outcomes-based validation and reliability assessment of noninvasive testing. Circulation 117:2793-2801, 2008.)
Added Value of Gated SPECT
Since gated SPECT has become routine only recently, there are several reports of its incremental value over perfusion in assessing prognosis. The first report showed that poststress LVEF and LV end-systolic volume (ESV), as measured by gated SPECT, provided incremental information over the perfusion defect assessment in the prediction of cardiac death.27 The results of these studies were in large part confirmed by reports from other centers.28,29
USE OF MPS IN SPECIFIC PATIENT POPULATIONS
A principal strength of nuclear cardiology is that large databases have been accumulated, resulting in evidence documenting the effectiveness of MPS for risk stratification of appropriately selected patients comprising the full spectrum of patients with suspected or chronic CAD. This evidence has resulted in many class I indications for the use of stress MPS.8 Several specific lines of evidence are described in the following sections.
Patient Cohorts Defined by CAD Likelihood and ECG Criteria
Patients With an Intermediate Likelihood of CAD or Indeterminate Treadmill Test
A number of studies support a role for MPS for risk stratification in patients with either intermediate post-ETT likelihood of CAD or patients with uninterpretable ETT results.8 An initial report from Cedars-Sinai demonstrated that MPS was effective in risk stratification and driving management of patients with an intermediate Duke Treadmill Score (DTS).12 Subsequent studies revealed that the cost-effectiveness of a strategy utilizing MPS is cost saving versus a strategy of direct referral to catheterization in these patients.4,30 Similar results were shown in subsequent multicenter studies reporting event rates and catheterization rates.8
Patients With Normal Resting ECG Able to Exercise
Patients with normal resting electrocardiograms (ECGs) have been a problematic group with respect to their appropriateness for stress imaging. On the one hand, in clinical practice, these patients represent a large subgroup regularly referred to MPS when, taking into account various clinical factors, the post-ETT risk is not low. On the other hand, patients with a normal resting ECG in general are likely (92% to 96%) to have normal LV function31,32 and to have an excellent prognosis.4,33 The reticence of many writers of guidelines to embrace the use of MPS in these patients is based in part on a study from the Mayo Clinic.34 Although these investigators demonstrated that MPS was able to reclassify the likelihood of anatomically severe CAD after considering clinical and ETT data, so few patients were reclassified with respect to their likelihood that MPS was not cost-effective. Hence, previous guidelines did not recommend the use of MPS in these patients,13 and use of MPS is controversial.
More recently, however, a study designed to parallel the study mentioned was reported, with the important distinction that it employed a prognostic rather than anatomic endpoint.4 Contrary to findings of the prior study using the anatomic definition of high risk, this study reported that selective use of MPS in patients with intermediate to high post-ETT CAD likelihood yielded significant risk stratification, statistical incremental value, and cost-effectiveness in predicting hard events (Fig. 16-8). A subsequent report has shown that patients with a high clinical risk (based on a clinical score combining age, sex, prior MI, and diabetic state) are at too high pretest risk to be classified as low risk by nonimaging exercise testing alone. The authors suggested that initial stress MPS testing might be appropriate in this group.35
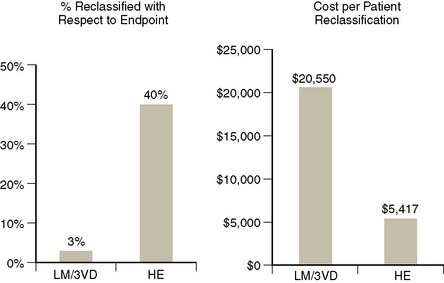
Figure 16-8 Comparison of two studies examining clinical and cost-effectiveness of MPS in patients with normal rest ECG and no prior history of CAD, one using an anatomic endpoint (presence of left main or three vessel CAD; 411 patients)34 and a second a prognostic endpoint (hard events; 3058 patients with a 1.6-year follow-up).4 On the left, the percent of patients reclassified with respect to their likelihood of the endpoint; on the right, the cost per patient reclassified. In the first study, very few patients were reclassified with respect to their risk of severe anatomic CAD, thus the cost per reclassification was unacceptably high. On the other hand, the use of a prognostic endpoint in this population resulted in considerably more patients reclassified with respect to the endpoint, thus a much lower cost per reclassification.
Thus, while referral of functionally capable patients with a normal ECG to MPS is considered inappropriate according to the ACC appropriateness criteria,7 recent studies indicate that there are patient groups able to exercise with normal rest ECG (such as those with a high pretest likelihood of CAD5 and the elderly) in which MPS may be indicated.
Patients With Normal Resting ECG Unable to Exercise
In patients unable to exercise to a target heart rate, there is a clear consensus supporting MPS using pharmacologic stress as the initial test in symptomatic male and female patients with intermediate or high pretest likelihood of CAD.8,36 As shown in multiple previous studies, the inability to exercise per se is itself an incremental predictor of adverse outcomes8,11,21,37 on par with prior CAD, abnormal MPS, or other high-risk markers. Despite the higher event rates for any test result, for patients who have a normal resting ECG and an intermediate to high likelihood of CAD but are unable to exercise, vasodilator stress MPS has been shown to be effective for both CAD diagnosis and risk stratification.8,20,38 The relative effectiveness of risk identification tends to be superior with pharmacologic versus exercise stress (due to the considerably greater event rates in the setting of abnormal MPS with pharmacologic stress).
Patients With High Pretest Likelihood of CAD
Historically, symptomatic patients without known CAD who have a high likelihood of CAD based on age, sex, symptoms, and risk factors were considered candidates for direct referral to revascularization. This was based on the argument that a normal ETT or MPS result would not be sufficient to reclassify the patient as having a low likelihood of CAD, hence precluding the ability for the clinician to confidently exclude the presence of angiographically significant CAD, resulting in diagnostic uncertainty. However, prognostically, it might be possible to classify such patients as low risk. A study assessing the clinical and cost-effectiveness of MPS in 1270 patients with a high CAD likelihood (=0.85) revealed that the majority of these patients had a normal MPS study (which had an associated hard event rate of 1.3%).5 A strategy incorporating initial testing with MPS in these patients to guide decision for coronary angiography was shown to be cost-effective.5,6 The ACC appropriateness criteria support MPS in high-likelihood patients who have an interpretable or uninterpretable ECG, as well as for those able or unable to exercise.7
Patients With Left Bundle Branch Block (See Chapter 5)
At the current time, the guidelines support the use of MPS in symptomatic patients with left bundle branch block, since ETT is not an option in these patients,8 and the rate of false-positive perfusion defects is observed less frequently with vasodilator stress. This is further supported by the finding of a greater specificity associated with vasodilator stress compared to exercise stress in these patients with similar sensitivities.39 This approach has also been found to be prognostically valuable and predictive of adverse outcomes in LBBB patients.40,41 Whereas patients with LBBB and normal MPS have relatively low event rates, patients with LBBB and abnormal MPS results tend to have greater event rates for any defect size compared to other patients.
Patients With LVH or Atrial Fibrillation
In patients with LVH, exertional ST-segment depression is frequently associated without significant CAD. MPS has been shown to be similarly effective in patients with and without LVH for identifying obstructive disease and for risk stratification. In one report, patients with LVH and a low-risk MPS had a less than 1% annual risk of cardiac death or nonfatal myocardial infarction, while the annual cardiac death or nonfatal myocardial infarction rates ranged from 4.9% for mildly abnormal scans to 10.3% for those with moderate to severely abnormal MPS.42
In asymptomatic patients with new-onset atrial fibrillation, the use of stress MPS in patients with a high pretest risk is considered appropriate7 in view of a higher baseline clinical risk, resulting in higher expected cardiac events. A study on the prognostic value of MPS in patients with atrial fibrillation reported an annualized cardiac death rate of 1.6% in the setting of a normal MPS result versus 0.4% for a normal MPS in patients without AF (P < 0.001).43 These authors also reported that a mildly abnormal MPS study in patients with atrial fibrillation is associated with a higher risk than in those without atrial fibrillation, potentially implying the need for a different threshold for determining the appropriateness of referral of these patients catheterization.
Patient Cohorts Defined by Risk Factors and Demographics
Asymptomatic Patients
The diagnostic and prognostic value of stress MPS in asymptomatic populations has been previously examined. The routine use of any test for detection of CAD in a population at low risk/low prevalence of CAD is unlikely to be effective and will be associated with high cost-effectiveness ratios and low positive predictive values. Nonetheless, these evaluations are often performed in patients with high-risk occupations (e.g., pilots, firefighters).8 However, specific asymptomatic populations who are at intermediate to high risk will be candidates for MPS. For example, asymptomatic siblings of patients with manifest CAD have been found to be at elevated risk of developing CAD and at higher risk of adverse outcomes subsequently.44 Similarly, certain diabetic patients and women, the former often asymptomatic and the latter with atypical or noncardiac symptoms, also fall into the asymptomatic category but may well be MPS candidates, depending on their estimated risk. The ACCF/ASNC appropriateness criteria consider the use of MPS in asymptomatic patients with a high Framingham risk and those classified as CAD risk equivalent (diabetics) to be appropriate.7
Nuclear Imaging in Patients With Diabetes Mellitus
Multiple reports, to date, have supported the value of MPS for risk stratification of diabetic patients.3 Event rates associated with any MPS result are greater in diabetic compared to nondiabetic patients (Fig. 16-9).19,20,45 These findings were confirmed in a multicenter series.46 In the latter study, diabetic women had the worst outcome for any given extent of myocardial infarction. In patients with normal MPS results, survival worsened sooner in diabetic compared to nondiabetic patients, suggesting that retesting of diabetics with normal studies might be needed earlier than in nondiabetics.21 In a study of 1430 diabetic patients (701 asymptomatic) followed for a mean of 2.1 years after MPS, Zellweger et al.47 reported that significant risk stratification was seen when comparing normal and abnormal MPS results in asymptomatic diabetic patients. They observed 1.6% and 3.4% annual hard event rates in those with normal and abnormal MPS scans, respectively. Of interest, this same report revealed that while risk stratification was also observed in the diabetics with angina and shortness of breath, the event rates for both the normal and abnormal scan groups were higher in these groups than in the asymptomatic diabetics (Fig. 16-10). It has been shown that 22% of asymptomatic diabetic patients have ischemia by adenosine MPS,48 but the preponderance of these patients with abnormal MPS had mildly abnormal studies. Nonetheless, another recent large study has shown that 59% of asymptomatic diabetics have abnormal stress MPS studies, including 20% with a “high-risk” scan.49 A further study by this latter group showed that ECG Q waves and/or evidence of peripheral artery disease identified the most suitable diabetic candidates for screening with MPS.50 The differences in these studies is likely explained by differences in underlying risk of the patients studied. Given the diversity of pretest risk in these various diabetic groups, some investigators recommend atherosclerosis testing rather than MPS as a more cost-effective approach to the initial screening tool of diabetics.51,52 A more recent statement from the American Diabetes Association recommended that testing for atherosclerosis or ischemia for patients with type 2 diabetes, perhaps with cardiac CT as the initial test, be reserved for those in whom medical treatment goals cannot be met. Similar recommendations were made for selected asymptomatic diabetics in whom there is strong clinical suspicion of very-high-risk CAD.53
Gender-Based Differences in the Prognostic Value of MPS
The historical limitations of MPS, related to breast tissue artifact and smaller left ventricular chamber size, has been ameliorated in large part with the advent of 99mTc agents. This is related to both enhanced image quality and the ability to gate SPECT images; prone imaging and/or the use of validated attenuation correction algorithms has further aided efforts.36,54
With respect to the prognostic value of MPS, the low risk associated with normal MPS is similar in men and women.8 This is limited, however, in female diabetics where event rates tend to be far greater.21 High-risk findings (e.g., >10% ischemic myocardium) elevated a woman’s risk by nearly 10-fold, with annual rates of major cardiac events of 6.3% for all women and 10.9% for diabetic subsets of women.55
Endothelial dysfunction and microvascular disease have been proposed as mechanisms for false-positive stress testing results, suggesting that some of these studies may represent true perfusion abnormalities without large-vessel CAD. Recent evidence suggests that these MPS perfusion findings may be associated with increased near-term risk of major cardiac events, more so in women than in men,56,57 suggesting that prognostically important coronary disease states not involving obstructive CAD occur more frequently in women than in men, and MPS could provide a tool for detection of this process.
Nuclear Imaging in Elderly Patients
The importance of MPS in an elderly population has grown because of two distinct factors: (1) the aging of the U.S. population and (2) the difficulty in assessing CAD in an elderly population in light of the frequency of asymptomatic and atypical presentations.58,59 This is further confounded by the reduced value of indices such as the DTS60 in an elderly population. Although a relatively smaller proportion of the elderly population is able to achieve adequate exercise on a treadmill, in those who are able to exercise, MPS provides effective risk stratification in elderly men and elderly women.61 This suggests the possibility that exercise MPS may replace ETT as the initial test in an elderly population.
Pharmacologic stress testing is increasingly being applied in the elderly, who frequently are unable to exercise adequately; this population accounts for a high proportion of patients undergoing pharmacologic stress imaging. For elderly patients, as well as for those with functional limitations, similar risk assessment is possible with pharmacologic stress SPECT.62–64 Consistent with data on other functionally impaired patients, the prognostic value of MPS is associated with higher cardiac event rates for normal to severely abnormal test results.
MPS in Patients With Chronic Kidney Disease
There is an increasing recognition of the cardiovascular implications of chronic kidney disease (CKD), and examination of the role of MPS in these patients. CKD is associated with hypertension and dyslipidemia, both promoters of atherosclerosis and further renal damage.65 Because diabetic nephropathy is the leading cause of CKD in the United States, diabetes is often present as well. In addition, CKD is also associated with activation of both inflammatory mediators and the renin-angiotensin system. These factors all contribute to accelerated atherosclerosis and early development of CAD in these patients. Additionally, CKD is associated with worsening risk for the entire spectrum of cardiovascular disease—for example, increased risk of thromboembolism in atrial fibrillation (AF), independent of other risk factors.66 As a result, patients with CKD are exposed to increased morbidity and mortality due to cardiovascular events.65,67 Indeed, the cardiovascular mortality rate in CKD patients is 15 to 30 times the age-adjusted cardiovascular mortality rate in the general population.65,68,69
Determining the role of MPS in this patient population is challenging. On the one hand, successful risk stratification of these patients by MPS results has been reported by a number of investigators.70–74 As we have touched on earlier, post-MPS risk is contextual, resulting in worsening event rates at every level of MPS abnormalities.21,75 In fact, the presence of CKD has been shown to increase risk at any level of MPS results. Hakeem and colleagues followed 1652 patients who underwent stress MPS for more than 2 years, finding that both stress perfusion defects and CKD were independent and incremental predictors of cardiac death after accounting for baseline data, risk factors, left ventricular dysfunction, type of stress used, and symptom status. Hence, MPS results add incrementally and risk-stratify these patients. As important, for any MPS result, normal or abnormal, cardiac mortality is far greater in CKD patients, and the degree of renal dysfunction is predictive of adverse outcome, even after adjusting for MPS data.74 However, in light of the relatively high event rates after a normal MPS, it remains unclear whether and how MPS results can guide the management of CKD patients. Is the risk associated with normal MPS in CKD patients amenable to treatment? Is it lower than the baseline risk of patients with kidney disease in the United States? Does therapeutic action based on abnormal MPS data result in improved patient outcomes?
MPS in Ethnic Minority Patients
Limited studies to date, have evaluated the impact of patient race on the association of MPS results and patient outcomes. This question is challenging in that defining this relationship necessitates the separation of socioeconomic and risk-factor components from patient race per se. The rate of cardiac death or nonfatal myocardial infarction in African Americans with a normal MPS is approximately 2% per year.8 Whether this is a function of inherent risk (as has been the case with other disease entities such as chronic renal failure76) or whether this is due to aggregation of risk factors and/or depressed socioeconomic conditions associated with patient race is unclear.
Evidence Supporting Nuclear Imaging for Obese Patients
Despite the potential obfuscation of scan interpretation in obese patients by attenuation artifact, MPS remains a highly useful test for diagnosis and prognosis in these patients. The use of both attenuation correction hardware and software in combination with quantitation and ECG gating, a well as prone imaging of stress images as a part of 99mTc protocols as an alternative to attenuation correction, have been associated with improvements in diagnostic test performance in obese cohorts.77
Several studies, to date, have demonstrated the value of MPS in the risk stratification of obese patients.78–80 Early data using PET imaging have also been associated with risk stratification in these patients.81 Whether normal MPS is associated with a “low” event rate in an obese cohort is unclear.80,81
MPS After Other Noninvasive Testing
MPS After Coronary Calcium Screening or Coronary CT Angiography (See Chapter 20)
A recent revision of ACC/AHA guidelines supports the use of CT-derived coronary calcium scores (CCS) as a means to evaluate asymptomatic patients with multiple risk factors for detection of early subclinical coronary atherosclerosis.82 Referral of patients first for CCS and then subsequently for MPS if extensive coronary atherosclerosis is found would seem clinically intuitive—patients with a high CCS would likely be at sufficient likelihood of CAD to justify MPS. Conversely, it may also be helpful to evaluate symptomatic patients with normal MPS and multiple risk factors for CCS determination to assess coronary atherosclerosis.
In an early study addressing this question, 46% of patients with CCS ≥ 400 had an abnormal MPS.83 These patients were selected from a large population presenting for CCS where patients with abnormal CCS were encouraged to undergo MPS. A subsequent study reported 1195 asymptomatic patients who underwent MPS and also had CCS, either on the basis of self-referral to CCS (8%), physician referral to CCS (65%), or recruitment into ongoing research (27%).84 In this study, CCS ≥ 400 was associated with a 29% frequency of any MPS ischemia and an 11% frequency of moderate to severe ischemia. Interestingly, a CCS threshold of ≥ 1000 was associated with only a 19.9% frequency of MPS ischemia (Fig. 16-11). Finally, a more recent report of 695 symptomatic patients referred for adenosine stress PET who underwent CCS as part of routine image acquisition reported a 48.5% frequency of abnormal stress PET in patients with CCS ≥ 400, with an only slightly greater frequency (49.4%) of abnormal PET with a CCS ≥ 1000.85 Interestingly, 16% of patients with no measurable calcium had PET-identified ischemia (negative predictive value 84%) (Fig. 16-12).
The discrepancies in the reported frequencies of abnormal MPS in patients with high CCS appear to be largely explained by differences in the underlying patient risk. Recent subset analyses have supported this concept, indicating that the threshold of CCS warranting referral for MPS will vary further as a function of underlying patient risk. In this regard, the frequency of abnormal MPS for any level of CCS has been reported to be higher in patients with type 2 diabetes,51,86 in patients with the metabolic syndrome,87 in patients with a family history of premature CAD,86 and in patients with a high likelihood of CAD.88 Although no validated threshold is currently recognized, in patient cohorts at greater risk for developing early atherosclerosis, it has been suggested that a threshold CCS of 100 might be appropriate for these patients. Nonetheless, the ACC appropriateness criteria support the use of MPS in patients with a high-risk CCS, or = 400.7
MPS in Patients with Prior CAD
Patients After Percutaneous Coronary Intervention
Despite these potential applications and the clear superiority of MPS to ETT techniques, a number of limitations exist in the use of MPS in these settings. First, the absolute risk of patients after PCI is relatively low (~1%), suggesting no need for routine testing despite the predictive value of abnormal MPS for adverse events.89,90 Given the relatively low prevalence of post-stent restenosis, the use of routine MPS post-PCI would be associated with the likelihood of a false-positive MPS exceeding the likelihood of a true-positive MPS.91 Indeed, due to the relatively low prevalence of clinically significant silent restenosis in the era of drug eluting stents, routine poststent MPS is not currently recommended.7,8
Evidence Supporting Nuclear Testing for Patients After CABG
MPS is frequently used in patients with prior coronary artery bypass grafting (CABG) to assess graft patency, the development of silent graft disease or progression of underlying graft disease, and to determine the importance of new symptoms. As more than 50% of vein grafts can be expected to be occluded by 10 years post-CABG, an intermediate likelihood of vein graft disease can be considered to be present at this time point.92
A number of studies have investigated the prognostic value of MPS in patients with prior CABG in various circumstances. MPS was prognostically valuable both when performed early (<2 years post-CABG)93 and later (≥5 years).89,94,95 MPS yielded incremental value with respect to death and nonfatal myocardial infarction even in asymptomatic, stable patients with prior CABG.96
USE OF MPS IN GUIDING DECISIONS FOR CATHETERIZATION
Several studies have shown that MPS results heavily influence post-MPS clinical decision making. Among patients with normal scans, only a small proportion undergo early post-MPS cardiac catheterization, usually as a result of persisting or worsening clinical symptomatology.11,12 Post-MPS referral to catheterization is overwhelmingly driven by the extent and severity of present on MPS.11,12 In patients without prior CAD, the relationship between the percent ischemic myocardium ischemic and the likelihood of referral to catheterization and/or revascularization after MPS is highly nonlinear and takes on a distinct shape (Fig. 16-13).11 Although the likelihood of this referral is very low in the absence of ischemia, in the range of small to medium amounts of ischemia, the slope of the relationship is very steep, particularly in the setting of anginal symptoms. This steep slope indicates that within this critical range of small to medium amounts of ischemia, small changes in reported ischemia yield large changes in the likelihood of physician action. Interestingly, once a threshold of ischemia is exceeded, there is no further increase in the likelihood of referral to revascularization (plateau phase).11 Importantly, referring physicians’ actions are not triggered by MPS results alone but factors such as ST-segment change on the electrocardiogram during stress, anginal symptoms on presentation, cardiovascular risk factors, and other clinical information also modulate the level of aggressiveness of post-MPS care.
ESTIMATING THE TRUE PROGNOSTIC VALUE OF MPS AND POSTTEST REFERRAL BIAS
An important consequence of the referral pattern described is the widely recognized post-MPS referral bias (partial verification bias) that has been widely described.97 This bias—the high referral rate to catheterization after abnormal MPS, the low referral rates to catheterization after normal MPS—results in a significant lowering of test specificity and a slight increase in test sensitivity. What is less widely understood is that this same pattern of post-MPS resource utilization introduces a referral bias that affects our abilities to assess the prognostic value of testing.98
Prognostic analyses performed, to date, are predominantly based on data from patients who underwent MPS and were then treated medically.97 This is the result of the accepted methodology; prognostic analyses of noninvasive testing using observational data series typically remove or censor patients undergoing early revascularization after testing, owing to the relationship between the referral to revascularization and the test results (since the test results drive the revascularization, an intervention that alters the natural history of the disease, the patients with the most abnormal test results are most likely to have their risk reduced). With increasing physician acceptance of and dependence on MPS to guide patient management, progressive increases in early revascularization rates in the setting of ischemia occur, accompanied by progressive decreases in patient risk after abnormal MPS (owing to the revascularization). Hence, studies evaluating medically treated patients will underestimate the prognostic value of MPS.2,3 The impact of this bias has been quantified by a recent study.5
This finding indicates that prognostic studies of MPS in medically treated patients drawn from routine practice may be misleading, particularly in the absence of information regarding post-MPS referral patterns to revascularization (the latter defining the amount of potential bias). This suggests that to avoid this bias, future studies of MPS (or the assessment of any modality in active use) include both patients treated medically and those referred to early revascularization. The implications of including the latter patients can be handled statistically to permit more accurate estimates of patient risk.2,11,99
Other more complicated biases can develop as well when data elements associated with risk and those associated with referral to revascularization become disparate.2,11,98,99 For example, if post-MPS referral to revascularization is based on one variable (e.g., ischemia) but not on a second (e.g., scar), a referral bias will result in underestimation of risk associated with the first variable (blunted increase in risk as a function of ischemia) but no such finding with respect to the second variable (appropriate increase in risk as a function of increasing scar). It is important to note that this type of bias is actually ubiquitous in prognostic research. Since, as was discussed earlier, revascularization referral is heavily based on ischemia, prognostic studies conducted in medically treated patients will underestimate the value of MPS ischemia in comparison to other factors. As noted, this bias can be overcome in two ways, either by utilizing MPS results obtained outside of conventional patient care pathways (e.g., research studies only) or statistical adjustment of observational patient data utilizing both medically treated and revascularized patients.2 Finally, the use of validated prognostic scores, such as the DTS, can also overcome this bias by including and appropriately weighting non-MPS factors, as well as basing the score on both medically treated and revascularization patients. Importantly, estimations of risk with both medical therapy and revascularization will need to be generated.
INCREMENTAL PROGNOSTIC VALUE OF PRE-MPS DATA
Understanding and Estimating Posttest Risk
In the previous discussion and in many reviews and reports of MPS, post-MPS risk is expressed as an annualized event rate, either for a general MPS result category (normal, abnormal MPS) or for a specific category of defect type (e.g., >20% myocardium ischemic). Most clinicians consider their patients’ post-MPS risk in this context and possibly make their clinical management decisions based on this approach as well. As seen in Figure 16-14, a wide range of posttest risk exists for any level of test abnormality. The risk of cardiac death associated with a moderate amount of ischemia, 10% to 20% of the myocardium, varies widely (from 2% to 10%) according to the patient subset examined. Patients with a higher clinical risk profile (increased age, pharmacologic stress, diabetic) will have higher risk than patients with lower clinical risk profiles (younger, exercise stress, nondiabetic). As noted previously, this same phenomenon also holds true after normal MPS.21
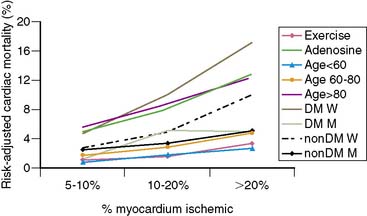
Figure 16-14 Rates of risk-adjusted cardiac mortality in medically treated patients as a function of percent myocardium ischemic (5% to 10%, 10% to 20%, and > 20%): exercise versus adenosine stress; patients aged < 60 years, 60 to 80 years, and > 80 years; diabetic men versus women and nondiabetic men and women. Although predicted cardiac mortality increases with increasing percent myocardium ischemic, the rates at any level of ischemia vary widely at any level of ischemia as a function of clinical information.45 DM W, diabetic women; DM M, diabetic men; nonDM W, nondiabetic women; NonDM M, nondiabetic men.
(From Hachamovitch R, Hayes SW, Friedman JD, Cohen I, Berman DS: Comparison of the short-term survival benefit associated with revascularization compared with medical therapy in patients with no prior coronary artery disease undergoing stress myocardial perfusion single photon emission computed tomography, Circulation 107:2900-2907, 2003.)
The Need for Imaging Scores in Risk Estimation and Reporting
Recently such a score was developed for patients undergoing adenosine stress.100 The first such score was developed in 5873 patients studied by adenosine stress who experienced 387 cardiac deaths on follow-up (6.6%). Using a combination of split-set validation and bootstrapping techniques, the authors derived three scores, including both a simplified score and a more complex score (with an eye to incorporation into MPS software). The complex score presented by these authors was as follows: (age [decades] × 5.19) + (% myocardium ischemic [per 10%] × 4.66) + (% myocardium fixed [per 10%] × 4.81) + (diabetes mellitus × 3.88) + (if patient treated with early revascularization, 4.51) + (if dyspnea was a presenting symptom, 5.47) + (resting heart rate [per 10 beats] × 2.88) – (peak heart rate [per 10 beats] × 1.42) + (ECG score × 1.95)—if patient treated with early revascularization, % myocardium ischemic [per 10%] × 4.47). Separate scores can be calculated for both the use of medical therapy and revascularization, and patient risk can be determined by use of Figure 16-15. This approach can be extended to incorporate a variety of information (clinical, imaging, biochemical, etc.) but will require validation in a variety of populations to ensure generalizability.
USING MPS FOR MEDICAL DECISION MAKING: IDENTIFYING RISK VERSUS IDENTIFYING POTENTIAL SURVIVAL BENEFIT
A recent study in 10,627 patients without prior myocardial infarction or revascularization who underwent stress MPS compared post-MPS outcomes with revascularization versus medical therapy using multivariable modeling with a propensity score. This study identified a survival benefit for patients undergoing medical therapy versus revascularization in the setting of no or mild ischemia, whereas patients undergoing revascularization had an increasing survival benefit over patients undergoing medical therapy when moderate to severe ischemia was present (>10% of the total myocardium ischemic) (Fig. 16-16).11 This survival benefit was particularly striking in higher-risk patients (elderly, requiring adenosine stress, and women, especially diabetics)(Fig. 16-17). These results have been extended to incorporate gated MPS EF information.99 Comparing the roles in risk assessment of perfusion and function data—although EF, percent myocardium ischemic, and the percent myocardium fixed are all predictors of cardiac death—the former is by far the best predictor of cardiac mortality. On the other hand, only inducible ischemia identified patients who would benefit from revascularization in comparison to medical therapy (Fig. 16-18). With increasing amounts of ischemia, increasing survival benefit for revascularization over medical therapy was found, irrespective of EF (Fig. 16-19). As shown by previous RCTs, the absolute benefit to be gained from a therapeutic strategy for any level of ischemia present is proportional to underlying patient risk. Thus, in assessing treatment options in an individual patient, cardiac risk factors, comorbidities, and EF all have to be considered along with ischemia in order to determine the potential advantages of a specific therapeutic strategy.
Imaging in the Post-COURAGE Era
In this context, the results of the recent COURAGE trial101 comparing strategies of PCI and medical therapy versus medical therapy alone in stable patients with known CAD must be mentioned as well. Since in this RCT, no survival advantage was present with the addition of PCI, the question must be raised as to whether stress imaging has a role in the future. After all, if patients will not benefit from revascularization, catheterization is not needed, so no stress imaging will be needed to identify the potential catheterization candidate. Several issues, however, stand in the way of this new paradigm.
First, does COURAGE really suggest that revascularization does not aid in reducing patient risk, or does it suggest that PCI may have limited prognostic impact? Also, the use of CABG would have yielded different results. Further, it is very important to recognize that COURAGE was a trial of patients with known CAD. Hence, these results cannot be generalized to patients presenting for evaluation of suspected CAD but without prior CAD. Of note, the observational data suggesting the potential use of MPS to identify patients with a survival benefit with one therapeutic approach versus another was limited to those patients without prior CAD.11,99
Finally, COURAGE included many patients with prior myocardial infarction and, possibly, scar without jeopardized myocardium. More recently, the results of the FAME (Fractional Flow Reserve Versus Angiography for Multivessel Evaluation) study revealed that guiding PCI by means of physiologic data (fractional flow reserve) was associated with a significantly lower incidence of major adverse cardiac events compared with routine angiography-guided PCI in patients with multivessel disease, without a significant increase in the procedure time.102 Whether the use of ischemia imaging in COURAGE to identify patients with jeopardized myocardium, hence enhanced benefit from revascularization, would have substantively altered the results of this trial is unknown. It is also unclear how much ischemia was present in the COURAGE patients, and whether the absence of demonstrable therapeutic benefit is related to insufficient amounts of ischemia in recruited patients. It must be noted that the results of COURAGE are generalizable to patients with known CAD but not to patients being evaluated with suspected CAD, a patient group with very different hazard function in whom very different results may have occurred.
New Paradigm: The Added Value Of a Modality Is Its Ability to Identify Candidates for Expensive Rx Resulting in Enhanced Clinical and Cost-Effectiveness
The application of MPS defined herein is one example of a new paradigm for cardiovascular imaging, the use of a modality to identify potential patient benefit, hence, aiding in the allocation of more expensive therapeutic options. Thus the finding of jeopardized myocardium (ischemic and/or hibernating) identifies candidates for PCI or CABG. This approach is readily applied to other current (and potential) roles of imaging techniques (Fig. 16-20).
Important Questions to Help Define the Role and Value of MPS
First, as already noted, it is recognized that MPS is susceptible to issues of “balanced reduction” of flow, potentially resulting in underestimation or even missing the presence of CAD entirely (Fig. 16-21). While it is likely that the frequency of this occurring is in part dependent on the patient profile of any given laboratory, the frequency of this phenomenon is unclear. If it is found to be significant, despite the use of various ancillary markers (TID, lung uptake of tracer, etc.), adjunct CT (for either CAC or CCTA) might be helpful. Alternatively, PET might be useful in this setting to assess coronary flow reserve, but whether this assessment will be sufficiently specific for cost-effective application has not yet been shown; that is, if no or mild perfusion abnormality is seen on myocardial perfusion PET but abnormal coronary flow reserve is seen, will this finding be sufficiently associated with severe ischemia to warrant invasive coronary angiography? Importantly, however, recent data indicate that PET without flow reserve can also circumvent “balanced reduction” by assessment of peak pharmacologic stress versus rest left ventricular ejection fraction.103
The clear advantage of CCTA is its ability to exclude the presence of coronary atherosclerosis and, in most cases, to exclude the presence of obstructive CAD because of its very high negative predictive value. The disadvantage of CCTA is that multiple limitations of the technology remain, including inability to characterize stenosis in areas with dense coronary calcification, dependence on slow heart rate and regular rhythm, and a tendency to overestimate stenosis severity. Additionally, the ability of CCTA to accurately identify the presence of hemodynamically significant stenosis is limited with current technology (Fig. 16-22).
The primary advantage of MPS is its being based on functional assessment of disease. The primary justification of stress imaging for prognostic assessment is based on the role of ischemia assessment in the management of the CAD patient. It is hypothesized (although not proven) that this will translate into identification of which patients may benefit from a revascularization strategy. Although with its advantages—avoidance of excess catheterizations, their associated cost and risk, and the potential “occulostenotic reflex,”6 differentiation of high-risk patients into those with extensive scar versus extensive ischemia,84 etc.—only indirect evidence supports its use inasmuch as no RCT to date compare medical therapy to revascularization on the basis of noninvasive ischemia estimates in stable patients.
As mentioned, the recently presented FAME study supports the concept that evaluation of the hemodynamic significance of CAD enhances the identification of what is optimal therapy for a given patient.102 These results are similar to older data showing that revascularization in patients with three-vessel CAD was associated with enhanced survival only in those patients with ischemic ETT results, whereas medical therapy was a superior initial therapy in patients without this finding.104 In the FAME study, data also parallel the results reported using MPS in patients without prior CAD in whom there was accrued survival benefit from revascularization over medical therapy only when significant ischemia was present,11 with the absolute benefit varying with underlying patient risk and LVEF.11,99
1. Di Carli M.F., Hachamovitch R. New technology for non-invasive evaluation of coronary artery disease. Circulation. 2007;115:1464-1480.
2. Hachamovitch R., Di Carli M.F. Contemporary reviews in cardiovascular medicine: Methods and limitations of assessing new noninvasive tests II. Outcomes-based validation and reliability assessment of noninvasive testing. Circulation. 2008;117:2793-2801.
3. Berman D.S., Hachamovitch R., Shaw L.J., Germano G., Hayes S. Nuclear Cardiology. In: Fuster V.A.R., King S., O’Rourke R.A., Wellens H.J.J., editors. Hurst’s The Heart. New York, NY: McGraw-Hill Companies; 2004:525-565. 2004
4. Hachamovitch R., Berman D.S., Kiat H., Cohen I., Friedman J.D., Shaw L.J. Value of stress myocardial perfusion single photon emission computed tomography in patients with normal resting electrocardiograms: an evaluation of incremental prognostic value and cost-effectiveness. Circulation. 2002;105:823-829.
5. Hachamovitch R., Hayes S., Friedman J., Cohen I., Berman D. Stress myocardial perfusion SPECT is clinically effective and cost-effective in risk-stratification of patients with a high likelihood of CAD but no known CAD. J Am Coll Cardiol. 2004;43:200-208.
6. Shaw L.J., Hachamovitch R., Berman D.S., Marwick T.H., Lauer M.S., Heller G.V., Iskandrian A.E., Kesler K.L., Travin M.I., Lewin H.C., Hendel R.C., Borges-Neto S., Miller D.D. The economic consequences of available diagnostic and prognostic strategies for the evaluation of stable angina patients: an observational assessment of the value of precatheterization ischemia. Economics of Noninvasive Diagnosis (END) Multicenter Study Group. J Am Coll Cardiol. 1999;33:661-669.
7. Brindis R.G., Douglas P.S., Hendel R.C., Peterson E.D., Wolk M.J., Allen J.M., Patel M.R., Raskin I.E., Bateman T.M., Cerqueira M.D., Gibbons R.J., Gillam L.D., Gillespie J.A., Iskandrian A.E., Jerome S.D., Krumholz H.M., Messer J.V., Spertus J.A., Stowers S.A. ACCF/ASNC appropriateness criteria for single-photon emission computed tomography myocardial perfusion imaging (SPECT MPI): a report of the American College of Cardiology Foundation Quality Strategic Directions Committee Appropriateness Criteria Working Group and the American Society of Nuclear Cardiology endorsed by the American Heart Association. J Am Coll Cardiol. 2005;46:1587-1605.
8. Klocke F.J., Baird M.G., Bateman T.M., Berman D.S., Carabello B.A., Cerqueira M.D., et al. ACC/AHA/ASNC Guidelines for the clinical use of cardiac radionuclide imaging: A report of the American 1995 guidelines for the clinical use of radionuclide imaging. Circulation. 2003.
9. Hachamovitch R., Beller G.A. Critical review of imaging approaches for diagnosis and prognosis of CAD. In: Di Carli M.F., Kwong R., editors. Novel Techniques for Imaging the Heart: Cardiac MR and CT. Oxford: Blackwell Publishing, 2008.
10. Hachamovitch R., Shaw L., Berman D.S. Methodological considerations in the assessment of noninvasive testing using outcomes research: pitfalls and limitations. Prog Cardiovasc Dis. 2000;43:215-230.
11. Hachamovitch R., Hayes S.W., Friedman J.D., Cohen I., Berman D.S. Comparison of the short-term survival benefit associated with revascularization compared with medical therapy in patients with no prior coronary artery disease undergoing stress myocardial perfusion single photon emission computed tomography. Circulation. 2003;107:2900-2907.
12. Hachamovitch R., Berman D.S., Kiat H., Cohen I., Cabico J.A., Friedman J., Diamond G.A. Exercise myocardial perfusion SPECT in patients without known coronary artery disease: incremental prognostic value and use in risk stratification. Circulation. 1996;93:905-914.
13. Gibbons R.J., Abrams J., Chatterjee K., Daley J., Deedwania P.C., Douglas J.S., Ferguson T.B.Jr, Fihn S.D., Fraker T.D.Jr, Gardin J.M., O’Rourke R.A., Pasternak R.C., Williams S.V. ACC/AHA 2002 guideline update for the management of patients with chronic stable angina—summary article: a report of the American College of Cardiology/American Heart Association Task Force on practice guidelines (Committee on the Management of Patients With Chronic Stable Angina). J Am Coll Cardiol. 2003;41:159-168.
14. Bateman T.M. Clinical relevance of a normal myocardial perfusion scintigraphic study. J Nucl Cardiol. 1997;4:172-173.
15. Heller G.V., Herman S.D., Travin M.I., Baron J.I., Santos-Ocampo C., McClellan J.R. Independent prognostic value of intravenous dipyridamole with technetium-99m sestamibi tomographic imaging in predicting cardiac events and cardiac-related hospital admissions. J Am Coll Cardiol. 1995;26:1202-1208.
16. Parisi A.F., Hartigan P.M., Folland E.D. Evaluation of exercise thallium scintigraphy versus exercise electrocardiography in predicting survival outcomes and morbid cardiac events in patients with single- and double-vessel disease. Findings from the Angioplasty Compared to Medicine (ACME) study. J Am Coll Cardiol. 1997;30:1256-1263.
17. Calnon D.A., McGrath P.D., Doss A.L., Harrell F.E.J., Watson D.D., Beller G.A. Prognostic value of dobutamine stress technetium-99msestamibi single-photon emission computed tomography myocardial perfusion imaging: stratification of a high-risk population. J Am Coll Cardiol. 2001;38:1511-1517.
18. Stratmann H.G., Tamesis B.R., Younis L.T., Wittry M.D., Miller D.D. Prognostic value of dipyridamole technetium-99m sestamibi myocardial tomography in patients with stable chest pain who are unable to exercise. Am J Cardiol. 1994;73:647-652.
19. Kang X., Berman D.S., Lewin H.C., Cohen I., Friedman J.D., Germano G., Hachamovitch R., Shaw L.J. Incremental prognostic value of myocardial perfusion single photon emission computed tomography in patients with diabetes mellitus. Am Heart J. 1999;138:1025-1032.
20. Berman D.S., Kang X., Hayes S.W., Friedman J.D., Cohen I., Abidov A., Shaw L.J., Amanullah A.M., Germano G., Hachamovitch R. Adenosine myocardial perfusion single-photon emission computed tomography in women compared with men. Impact of diabetes mellitus on incremental prognostic value and effect on patient management. J Am Coll Cardiol. 2003;41:1125-1133.
21. Hachamovitch R., Hayes S., Friedman J.D., Cohen I., Shaw L.J., Germano G., Berman D.S. Determinants of risk and its temporal variation in patients with normal stress myocardial perfusion scans: what is the warranty period of a normal scan? J Am Coll Cardiol. 2003;41:1329-1340.
22. Shaw L., Chaitman B.R., Hilton T.C., Stocke K.S., Younis L.T., Caralis D.G., Kong B.A., Miller D.D. Prognostic value of dipyridamole thallium-201 imaging in elderly patients. J Am Coll Cardiol. 1992;19:1390-1398.
23. Bax J.J., Young L.H., Frye R.L., Bonow R.O., Steinberg H.O., Barrett E.J. ADA. Screening for coronary artery disease in patients with diabetes. Diabetes Care. 2007;30:2729-2736.
24. Kamalesh M., Feigenbaum D.H., Sawada S. Challenge of identifying patients with diabetes mellitus who are at low risk for coronary events by use of cardiac stress imaging. Am Heart J. 2004;147:561-563.
25. Berman D.S., Hachamovitch R., Kiat H., Cohen I., Cabico J.A., Wang F.P., Friedman J.D., Germano G., Van Train K., Diamond G.A: Incremental value of prognostic testing in patients with known or suspected ischemic heart disease: a basis for optimal utilization of exercise technetium-99m sestamibi myocardial perfusion single-photon emission computed tomography [published erratum appears in J Am Coll Cardiol, 27(3):756, 1996], J Am Coll Cardiol, 26:639-647, 1995.
26. Abidov A., Hachamovitch R., Hayes S.W., Ng C.K., Cohen I., Friedman J.D., Germano G., Berman D.S. Prognostic impact of hemodynamic response to adenosine in patients older than age 55 years undergoing vasodilator stress myocardial perfusion study. Circulation. 2003;107:2894-2899.
27. Sharir T., Berman D.S., Lewin H.C., Friedman J.D., Cohen I., Miranda R., Agafitei R.D., Germano G. Incremental prognostic value of rest-redistribution (201)Tl single-photon emission computed tomography. Circulation. 1999;100:1964-1970.
28. Thomas G.S., Miyamoto M.I., Morello A.P.3rd, Majmundar H., Thomas J.J., Sampson C.H., Hachamovitch R., Shaw L.J. Technetium 99m sestamibi myocardial perfusion imaging predicts clinical outcome in the community outpatient setting. The Nuclear Utility in the Community (NUC) Study. J Am Coll Cardiol. 2004;43:213-223.
29. Travin M.I., Heller G.V., Johnson L.L., Katten D., Ahlberg A.W., Isasi C.R., Kaplan R.C., Taub C.C., Demus D. The prognostic value of ECG-gated SPECT imaging in patients undergoing stress Tc-99m sestamibi myocardial perfusion imaging. J Nucl Cardiol. 2004;11:253-262.
30. Shaw L.J., Miller D.D., Romeis J.C., Younis L.T., Gillespie K.N., Kimmey J.R., Chaitman B.R. Prognostic value of noninvasive risk stratification in younger and older patients referred for evaluation of suspected coronary artery disease. J Am Geriatr Soc. 1996;44:1190-1197.
31. O’Keefe J.H.Jr, Zinsmeister A.R., Gibbons R.J. Value of normal electrocardiographic findings in predicting resting left ventricular function in patients with chest pain and suspected coronary artery disease. Am J Med. 1989;86:658-662.
32. Christian T.F., Miller T.D., Chareonthaitawee P., Hodge D.O., O’Connor M.K., Gibbons R.J. Prevalence of normal resting left ventricular function with normal rest electrocardiograms. Am J Cardiol. 1997;79:1295-1298.
33. Ladenheim M.L., Kotler T.S., Pollock B.H., Berman D.S., Diamond G.A. Incremental prognostic power of clinical history, exercise electrocardiography and myocardial perfusion scintigraphy in suspected coronary artery disease. Am J Cardiol. 1987;59:270-277.
34. Christian T.F., Miller T.D., Bailey K.R., Gibbons R.J. Exercise tomographic thallium-201 imaging in patients with severe coronary artery disease and normal electrocardiograms. Ann Intern Med. 1994;121:825-832.
35. Poornima I.G., Miller T.D., Christian T.F., Hodge D.O., Bailey K.R., Gibbons R.J. Utility of myocardial perfusion imaging in patients with low-risk treadmill scores. J Am Coll Cardiol. 2004;43:194-199.
36. Mieres J.H., Shaw L.J., Arai A., Budoff M.J., Flamm S.D., Hundley W.G., Marwick T.H., Mosca L., Patel A.R., Quinones M.A., Redberg R.F., Taubert K.A., Taylor A.J., Thomas G.S., Wenger N.K. Role of noninvasive testing in the clinical evaluation of women with suspected coronary artery disease: Consensus statement from the Cardiac Imaging Committee, Council on Clinical Cardiology, and the Cardiovascular Imaging and Intervention Committee, Council on Cardiovascular Radiology and Intervention, American Heart Association. Circulation. 2005;111:682-696.
37. Hachamovitch R., Berman D.S., Shaw L.J., Kiat H., Cohen I., Cabico J.A., Friedman J., Diamond G.A. Incremental prognostic value of myocardial perfusion single photon emission computed tomography for the prediction of cardiac death: differential stratification for risk of cardiac death and myocardial infarction. Circulation. 1998;97:535-543.
38. Hachamovitch R., Hayes S., Friedman J., Cohen I., Berman D.S. A prognostic score for prediction of cardiac mortality risk after adenosine stress myocardial perfusion scintigraphy. J Am Coll Cardiol. 2005;45:722-729.
39. Vaduganathan P., He Z.X., Raghavan C., Mahmarian J.J., Verani M.S. Detection of left anterior descending coronary artery stenosis in patients with left bundle branch block: exercise, adenosine or dobutamine imaging? J Am Coll Cardiol. 1996;28:543-550.
40. Nallamothu N., Bagheri B., Acio E.R., Heo J., Iskandrian A.E. Prognostic value of stress myocardial perfusion single photon emission computed tomography imaging in patients with left ventricular bundle branch block. J Nucl Cardiol. 1997;4:487-493.
41. Wagdy H.M., Hodge D., Christian T.F., Miller T.D., Gibbons R.J. Prognostic value of vasodilator myocardial perfusion imaging in patients with left bundle-branch block. Circulation. 1998;97:1563-1570.
42. Amanullah A.M., Berman D.S., Kang X., Cohen I., Germano G., Friedman J.D. Enhanced prognostic stratification of patients with left ventricular hypertrophy with the use of single-photon emission computed tomography. Am Heart J. 2000;140:456-462.
43. Abidov A., Hachamovitch R., Rozanski A., Hayes S.W., Santos M.M., Sciammarella M.G., Cohen I., Gerlach J., Friedman J.D., Germano G., Berman D.S. Prognostic implications of atrial fibrillation in patients undergoing myocardial perfusion single-photon emission computed tomography. J Am Coll Cardiol. 2004;44:1062-1070.
44. Blumenthal R.S., Becker D.M., Moy T.F., Coresh J., Wilder L.B., Becker L.C. Exercise thallium tomography predicts future clinically manifest coronary heart disease in a high-risk asymptomatic population. Circulation. 1996;93:915-923.
45. Hachamovitch R., Berman D.S. The use of nuclear cardiology in clinical decision making. Semin Nucl Med. 2005;35:62-72.
46. Giri S., Shaw L.J., Murthy D.R., Travin M.I., Miller D.D., Hachamovitch R., Borges-Neto S., Berman D.S., Waters D.D., Heller G.V. Impact of diabetes on the risk stratification using stress single-photon emission computed tomography myocardial perfusion imaging in patients with symptoms suggestive of coronary artery disease. Circulation. 2002;105:32-40.
47. Zellweger M.J., Hachamovitch R., Kang X., Hayes S.W., Friedman J.D., Germano G., Pfisterer M.E., Berman D.S. Prognostic relevance of symptoms versus objective evidence of coronary artery disease in diabetic patients. Eur Heart J. 2004;25:543-550.
48. Wackers F.J., Young L.H., Inzucchi S.E., Chyun D.A., Davey J.A., Barrett E.J., Taillefer R., Wittlin S.D., Heller G.V., Filipchuk N., Engel S., Ratner R.E., Iskandrian A.E. Detection of silent myocardial ischemia in asymptomatic diabetic subjects: the DIAD study. Diabetes Care. 2004;27:1954-1961.
49. Miller T.D., Rajagopalan N., Hodge D.O., Frye R.L., Gibbons R.J. Yield of stress single-photon emission computed tomography in asymptomatic patients with diabetes. Am Heart J. 2004;147:890-896.
50. Rajagopalan N., Miller T.D., Hodge D.O., Frye R.L., Gibbons R.J. Identifying high-risk asymptomatic diabetic patients who are candidates for screening stress single-photon emission computed tomography imaging. J Am Coll Cardiol. 2005;45:43-49.
51. Anand D.V., Lim E., Hopkins D., Corder R., Shaw L.J., Sharp P., Lipkin D., Lahiri A. Risk stratification in uncomplicated type 2 diabetes: prospective evaluation of the combined use of coronary artery calcium imaging and selective myocardial perfusion scintigraphy. Eur Heart J. 2006;27:713-721.
52. Berman D., Hachamovitch R., Shaw L.J., Friedman J.D., Hayes S.W., Thomson L.E., Fieno D.S., Germano G., Slomka P.J., Wong N.D., Kang X., Rozanski A. Roles of nuclear cardiology, cardiac computed tomography, and cardiac magnetic resonance: noninvasive risk stratification and a conceptual framework for the selection of noninvasive imaging tests in patients with known or suspected coronary artery disease. J Nucl Med. 2006;47:1107-1118.
53. Bax J.J., van der Wall E.E. Assessment of coronary artery disease in patients with (a)symptomatic diabetes. Eur Heart J. 2006;27:631-632.
54. Slomka P.J., Nishina H., Abidov A., Hayes S.W., Friedman J.D., Berman D.S., Germano G. Combined quantitative supine-prone myocardial perfusion SPECT improves detection of coronary artery disease and normalcy rates in women. J Nucl Cardiol. 2006. In press
55. Shaw L.J., Iskandrian A.E. Prognostic value of gated myocardial perfusion SPECT. J Nucl Cardiol. 2004;11:171-185.
56. Shaw L.J., Bairey Merz C.N., Pepine C.J., Reis S.E., Bittner V., Kelsey S.F., Olson M., Johnson B.D., Mankad S., Sharaf B.L., Rogers W.J., Wessel T.R., Arant C.B., Pohost G.M., Lerman A., Quyyumi A.A., Sopko G. Insights from the NHLBI-Sponsored Women’s Ischemia Syndrome Evaluation (WISE) Study: Part I: gender differences in traditional and novel risk factors, symptom evaluation, and gender-optimized diagnostic strategies. J Am Coll Cardiol. 2006;47:S4-S20.
57. Bugiardini R., Bairey Merz C.N. Angina with “normal” coronary arteries: a changing philosophy. JAMA. 2005;293:477-484.
58. Nadelmann J., Frishman W.H., Ooi W.L., et al. Prevalence, incidence, and prognosis of recognized and unrecognized myocardial infarction in persons aged 75 years or older: the Bronx Aging Study. Am J Cardiol. 1990;66:533-537.
59. Sigurdsson E., Thorgeirsson G., Sigvaldason H., Sigfusson N. Unrecognized myocardial infarction: epidemiology, clinical characteristics, and the prognostic role of angina pectoris. The Reykjavik Study. Ann Intern Med. 1995;122:96-102.
60. Kwok J.M., Miller T.D., Hodge D.O., Gibbons R.J. Prognostic value of the Duke Treadmill Score in the elderly. J Am Coll Cardiol. 2002;39:1475-1481.
61. Valeti U.S., Miller T.D., Hodge D.O., Gibbons R.J. Exercise single-photon emission computed tomography provides effective risk stratification of elderly men and elderly women. Circulation. 2005;111:1771-1776.
62. Zafrir N., Mats I., Solodky A., Ben-Gal T., Sulkes J., Battler A. Prognostic value of stress myocardial perfusion imaging in octogenarian population. J Nucl Cardiol. 2005;12:671-675.
63. Schinkel A.F., Elhendy A., Biagini E., van Domburg R.T., Valkema R., Rizello V., Pedone C., Simoons M., Bax J.J., Poldermans D. Prognostic stratification using dobutamine stress 99mTc-tetrofosmin myocardial perfusion SPECT in elderly patients unable to perform exercise testing. J Nucl Med. 2005;46:12-18.
64. Lima R.S., De Lorenzo A., Pantoja M.R., Siqueira A. Incremental prognostic value of myocardial perfusion 99m-technetium-sestamibi SPECT in the elderly. Int J Cardiol. 2004;93:137-143.
65. Schiffrin E.L., Lipman M.L., Mann J.F.E. Chronic kidney disease: Effects on the cardiovascular system. Circulation. 2007;116:85-97.
66. Go A.S., Fang M.C., Udaltsova N., et al: Impact of proteinuria and glomerular filtration rate on risk of thromboembolism in atrial fibrillation: The Anticoagulation and Risk Factors in Atrial Fibrillation (ATRIA) Study, 2009.
67. Verma A., Anavekar N.S., Meris A., Thune J.J., Arnold J.M., Ghali J.K., Velazquez E.J., McMurray J.J., Pfeffer M.A., Solomon S.D. The relationship between renal function and cardiac structure, function, and prognosis after myocardial infarction: the VALIANT Echo Study. J Am Coll Cardiol. 2007;50:1238-1245.
68. Go A.S., Chertow G.M., Fan D., McCulloch C.E., Hsu C.Y. Chronic kidney disease and the risks of death, cardiovascular events, and hospitalization. N Engl J Med. 2004;351:1296-1305.
69. Tonelli M., Wiebe N., Culleton B., House A., Rabbat C., Fok M., McAlister F., Garg A.X. Chronic kidney disease and mortality risk: a systematic review. J Am Soc Nephrol. 2006;17:2034-2047.
70. Al-Mallah M., Hachamovitch R., Dorbala S., Kwong R., Hainer J., Di Carli M.F. Prognostic value of myocardial perfusion imaging in patients with impaired renal function. J Nucl Cardiol. 2007;14:S125. (abs)
71. Patel A.D., Abo-Auda W.S., Davis J.M., Zoghbi G.J., Deierhoi M.H., Heo J., Iskandrian A.E. Prognostic value of myocardial perfusion imaging in predicting outcome after renal transplantation. Am J Cardiol. 2003;92:146-151.
72. Dahan M., Viron B.M., Faraggi M., Himbert D.L., Lagallicier B.J., Kolta A.M., Pessione F., Le Guludec D., Gourgon R., Mignon F.E. Diagnostic accuracy and prognostic value of combined dipyridamole-exercise thallium imaging in hemodialysis patients. Kidney Int. 1998;54:255-262.
73. Venkataraman R., Hage F.G., Dorfman T., Heo J., Aqel R.A., de Mattos A.M., Iskandrian A.E. Role of myocardial perfusion imaging in patients with end-stage renal disease undergoing coronary angiography. Am J Cardiol. 2008;102:1451-1456.
74. Hakeem A., Bhatti S., Dillie K.S., Cook J.R., Samad Z., Roth-Cline M.D., Chang S.M. Predictive value of myocardial perfusion single-photon emission computed tomography and the impact of renal function on cardiac death. Circulation. 2008;118:2540-2549.
75. Berman D.S., Kang X., Hayes S.W., Friedman J., Cohen I., Abidov A., Shaw L., Amanullah A.M., Germano G., Hachamovitch R. Adenosine myocardial perfusion single-photon emission computed tomography in women compared with men. Impact of diabetes mellitus on incremental prognostic value and effect on patient management. J Am Coll Cardiol. 2003;41:1125-1133.
76. Suthanthiran M., Li B., Song J.O., Ding R., Sharma V.K., Schwartz J.E., August P. Transforming growth factor-beta 1 hyperexpression in African-American hypertensives: A novel mediator of hypertension and/or target organ damage. Proc Natl Acad Sci U S A. 2000;97:3479-3484.
77. Nishina H., Slomka P.J., Abidov A., Yoda S., Akincioglu C., Kang X., Cohen I., Hayes S.W., Friedman J.D., Germano G., Berman D.S. Combined supine and prone quantitative myocardial perfusion SPECT: method development and clinical validation in patients with no known coronary artery disease. J Nucl Med. 2006;47:51-58.
78. Kang X., Shaw L.J., Hayes S.W., Hachamovitch R., Abidov A., Cohen I., Friedman J.D., Thomson L.E., Polk D., Germano G., Berman D.S. Impact of body mass index on cardiac mortality in patients with known or suspected coronary artery disease undergoing myocardial perfusion single-photon emission computed tomography. J Am Coll Cardiol. 2006;47:1418-1426.
79. Elhendy A., Schinkel A.F., van Domburg R.T., Bax J.J., Valkema R., Biagini E., Poldermans D. Prognostic stratification of obese patients by stress 99mTc-tetrofosmin myocardial perfusion imaging. J Nucl Med. 2006;47:1302-1306.
80. Duvall W.L., Croft L.B., Corriel J.S., Einstein A.J., Fisher J.E., Haynes P.S., Rose R.K., Henzlova M.J. SPECT myocardial perfusion imaging in morbidly obese patients: image quality, hemodynamic response to pharmacologic stress, and diagnostic and prognostic value. J Nucl Cardiol. 2006;13:202-209.
81. Yoshinaga K., Chow B.J., Williams K.A., Chen L., deKemp R.A., Garrard L., Lok-Tin Szeto A., Aung M., Davies R.A., Ruddy T.D., Beanlands R.S. What is the prognostic value of myocardial perfusion imaging using rubidium-82 positron emission tomography? J Am Coll Cardiol. 2006;48:1029-1039.
82. Greenland P., Bonow R.O., Brundage B.H., Budoff M.J., Eisnberg M.J., Grundy S.M., Lauer M.S., Post W.S., Raggi P., Redberg R.F., Rodgers G.P., Shaw L.J., Taylor A.J., Weintraub W.S. Computed tomography: ACC/AHA writing committee to update the 2000 clinical expert consensus document on electron-beam computed tomography for the diagnosis and prognosis for coronary artery disease. J Am Coll Cardiol. 2006;47:998-1004.
83. He Z.X., Hedrick T.D., Pratt C.M., Verani M.S., Aquino V., Roberts R., Mahmarian J.J. Severity of coronary artery calcification by electron beam computed tomography predicts silent myocardial ischemia. Circulation. 2000;101:244-251.
84. Berman D.S., Wong N.D., Gransar H., Miranda-Peats R., Dahlbeck J., Arad Y., Hayes S.W., Friedman J.D., Kang X., Polk D., Hachamovitch R., Rozanski A. Relationship between stress-induced myocardial ischemia and atherosclerosis measured by coronary calcium tomography. J Am Coll Cardiol. 2004;44:923-930.
85. Schenker M.P., Dorbala S., Hong E.C., Rybicki F.J., Hachamovitch R., Kwong R.Y., Di Carli M.F. Interrelation of coronary calcification, myocardial ischemia, and outcomes in patients with intermediate likelihood of coronary artery disease: a combined positron emission tomography/computed tomography study. Circulation. 2008;117:1693-1700.
86. Blumenthal R.S., Becker D.M., Yanek L.R., Moy T.F., Michos E.D., Fishman E.K., Becker L.C. Comparison of coronary calcium and stress myocardial perfusion imaging in apparently healthy siblings of individuals with premature coronary artery disease. Am J Cardiol. 2006;97:328-333.
87. Wong N.D., Rozanski A., Gransar H., Miranda-Peats R., Kang X., Hayes S., Shaw L., Friedman J., Polk D., Berman D.S. Metabolic syndrome and diabetes are associated with an increased likelihood of inducible myocardial ischemia among patients with subclinical atherosclerosis. Diabetes Care. 2005;28:1445-1450.
88. Rozanski A., Gransar H., Wong N.D., Shaw L.J., Miranda-Peats R., Hayes S.W., Friedman J.D., Berman D.S. Use of coronary calcium scanning for predicting inducible myocardial ischemia: Influence of patients’ clinical presentation. J Nucl Cardiol. 2007;14:669-679.
89. Zellweger M.J., Berman D., Shaw L., et al. Evaluation of patients after intervention. In: Poshost G., et al, editors. Imaging in Cardiovascular Medicine. Philadelphia: Lippincott Williams & Wilkins, 2000.
90. Ho K.T., Miller T.D., Holmes D.R., Hodge D.O., Gibbons R.J. Long-term prognostic value of Duke treadmill score and exercise thallium-201 imaging performed one to three years after percutaneous transluminal coronary angioplasty. Am J Cardiol. 1999;84:1323-1327.
91. Garzon P.P., Eisenberg M.J. Functional testing for the detection of restenosis after percutaneous transluminal coronary angioplasty: a meta-analysis. Can J Cardiol. 2001;17:41-48.
92. Grondin C.M., Campeau L., Lespérance J., Enjalbert M., Bourassa M.G. Comparison of late changes in internal mammary artery and saphenous vein grafts in two consecutive series of patients 10 years after operation. Circulation. 1984;70(3 Pt 2):I208-I212.
93. Miller T.D., Christian T.F., Hodge D.O., Mullan B.P., Gibbons R.J. Prognostic value of exercise thallium-201 imaging performed within 2 years of coronary artery bypass graft surgery. J Am Coll Cardiol. 1998;31:848-854.
94. Zellweger M.J., Lewin H.C., Lai S., Dubois E.A., Friedman J.D., Germano G., Kang X., Sharir T., Berman D.S. When to stress patients after coronary artery bypass surgery? Risk stratification in patients early and late post-CABG using stress myocardial perfusion SPECT: implications of appropriate clinical strategies. J Am Coll Cardiol. 2001;37:144-152.
95. Palmas W., Bingham S., Diamond G.A., Denton T.A., Kiat H., Friedman J.D., Scarlata D., Maddahi J., Cohen I., Berman D.S. Incremental prognostic value of exercise thallium-201 myocardial single-photon emission computed tomography late after coronary artery bypass surgery. J Am Coll Cardiol. 1995;25:403-409.
96. Lauer M.S., Lytle B., Pashkow F., Snader C.E., Marwick T.H. Prediction of death and myocardial infarction by screening with exercise-thallium testing after coronary-artery-bypass grafting. Lancet. 1998;351:615-622.
97. Hachamovitch R., Di Carli M.F. Contemporary reviews in cardiovascular medicine: Methods and limitations of assessing new noninvasive tests: I. Anatomy-based validation of noninvasive testing. Circulation. 2008;117:2684-2690.
98. Hachamovitch R., Hayes S.W., Friedman J.D., Cohen I., Kang X., Germano G., Berman D.S. Is there a referral bias against revascularization of patients with reduced LV ejection fraction? Influence of ejection fraction and inducible ischemia on post-SPECT management of patients without history of CAD. J Am Coll Cardiol. 2003;42:1286-1294.
99. Hachamovitch R., Rozanski A., Hayes S.W., Thomson L.E.J., Germano G., Friedman J.D., Cohen I., Berman D.S. Predicting therapeutic benefit from myocardial revascularization procedures: Are measurements of resting left ventricular ejection fraction and stress-induced myocardial ischemia both necessary. J Nucl Cardiol. 2006;13(6):768-778.
100. Hachamovitch R., Hayes S.W., Friedman J.D., Cohen I., Berman D.S. A prognostic score for prediction of cardiac mortality risk after adenosine stress myocardial perfusion scintigraphy. J Am Coll Cardiol. 2005;45:722-729.
101. Boden W.E., O’Rourke R.A., Teo K.K., Hartigan P.M., Maron D.J., Kostuk W.J., et al. Optimal medical therapy with or without PCI for stable coronary disease. N Engl J Med. 2007:356.
102. Fractional Flow Reserve Versus Angiography for Multivessel Evaluation (FAME): Presented at TCT 2008, 2008.
103. Dorbala S., Vangala D., Sampson U.K., Limaye A., Kwong R., Di Carli M.F. Value of vasodilator left ventricular ejection fraction reserve in evaluating the magnitude of myocardium at risk and the extent of angiographic coronary artery disease: a 82Rb PET/CT study. J Nucl Med. 2007;48:349-358.
104. Weiner D.A., Ryan T.J., McCabe C.H., Chaitman B.R., Sheffield L.T., Fisher L.D., Tristani F. The role of exercise testing in identifying patients with improved survival after coronary artery bypass surgery. J Am Coll Cardiol. 1986;8:741-748.