CHAPTER 7 Principles of ultrasound-guided regional anesthesia
Introduction to ultrasound
Ultrasound is a mechanical wave with frequencies over 20 000 Hz. Ultrasound used in medicine is generated and sensed by piezoelectric crystals. The ultrasound transducer incorporates a battery of piezoelectric crystals. When scanning, the transducer switches quickly between transmitter and receiver modes. When in transmitting mode, the piezoelectric crystals are stimulated by electrical energy, vibrate and emit ultrasound waves. In the receiver mode the crystals are hit by the ultrasound waves reflected from the tissues (Fig 7.1). The resultant mechanical stimulation of the crystals is converted to electrical signals, which are processed and ultimately create the image we see on the screen.
Why understanding ultrasound physics and how to use an ultrasound machine is important
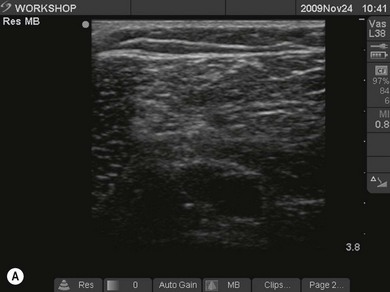
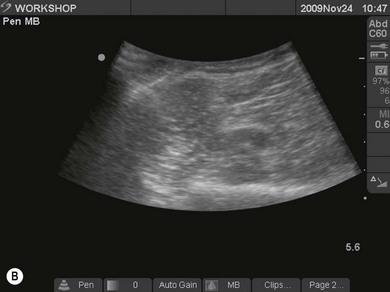
Figure 7.3 (A). High frequency transducer: deep structures do not visualize due to the absorbtion of the ultrasound. Note the good resolution at superficial level. (B) Low frequency transducer: deep structures visualized due to the better penetration of the ultrasound. Note the image has lower resolution, compared with Fig. 7.3A.
Ultrasound physics
Tissue | Acoustic impedance (g/cm2 sec × 100) |
---|---|
Air | 0.0004 |
Fat | 1.3 |
Water | 1.5 |
Blood | 1.6 |
Muscle | 1.7 |
Bone | 7 |
The ultrasound machine
The transducer
Transducers vary in size, shape, frequency range and number of piezoelectric crystals. For superficial blocks, a high frequency transducer (7–15 MHz) will provide better axial resolution (i.e. better ability to distinguish as separate structures dots lying along the path of the ultrasound beam) (Fig. 7.3). The more piezoelectric crystal elements, the better the resolution. A lower frequency transducer (1–5 MHz) is more appropriate for deeper blocks as there is less absorption and thus better signal from the deeper structures (Fig. 7.3). Transducers with a small footprint (i.e. hockey stick transducers) are useful in children or where space is limiting (Fig. 7.8). Wider (with large footprint) and curvilinear transducers (sector) allow for visualization of a bigger area and thus may be helpful in visualizing landmark structures at the same time as the nerves of interest (Fig. 7.8).
Ultrasound tissue appearance
Fat tissue is usually located most superficially (appears on top of the screen), is hypoechoic with interspersed irregular brighter lines of connective tissue (Fig 7.7).
Muscle is hypoechoic (though appears brighter than the fat tissue), with more granular texture, and is surrounded by a hyperechoic fascial sheath (Fig 7.7).
Vessels are anechoic (Fig. 7.10), compressible (veins more than arteries) (Fig 7.10), and pulsatile (usually only the arteries). Doppler will visualize flow (usually continuous for veins and pulsatile for arteries) (Fig 7.4). One can distend a collapsed vein (for example when scanning in the cervical area) by asking the patient to perform a Valsalva maneuver (Fig 7.10).
Bone is highly reflective. It visualizes as a hyperechoic band, behind which there is an acoustic shadow due to poor penetration (Fig 7.7).
Nerve imaging varies with the location. The proximal parts (i.e. nerve roots when scanning the interscalene area) are rich in nerve tissue and appear black or hypoechoic (similar to vessels) (Fig 7.10). As the brachial plexus runs distally (i.e. supraclavicular area), the proportion of connective tissue increases and the nerve has a grape-like appearance (Fig. 7.11). Further distally in the upper limb, the connective tissue dominates and the nerves appear more hyperechoic (Fig 7.7), or may display a honeycomb pattern (Fig. 7.12). The fascicles are hypoechoic dots with a surrounding hyperechoic rim (epineurium). Anisotropy can affect the ultrasound appearance of nerves (Fig. 7.13). The above comments relate to transverse imaging of nerves. On longitudinal imaging, nerves have a fascicular pattern unlike the fibrillar pattern of tendons (Fig. 7.14).
Tendons are hyperechoic and often look similar to peripheral nerves (Fig 7.12). There are some tips that help to differentiate them. When scanning dynamically, one can follow the course of a tendon and observe it merging into a muscle. Nerves are continuous, and can be demonstrated to change course or give divisions when scanned along their course. If we ask the patient to activate the respective muscles, there is more obvious movement of the tendons compared to the nerves near them.
Fascias appear very bright (hyperechoic) (Fig 7.7).
Pleura visualizes as a hyperechoic line, while the lung tissue is hypoechoic (Fig 7.11). Occasionally, one can see a comet-tail sign under the pleura caused by reverberation (see below) at the pleural interface.
Artifacts
Anisotropy refers to the variable echogenicity of tissues when the incidence angle of the ultrasound beam is changed. By angling the transducer more distally or proximally one can significantly improve the visualization of a scanned nerve (Fig 7.13).
Loss of image. There are many reasons why an anatomical structure is not seen with ultrasound. The following are some examples. When the contact between the transducer and the skin is incomplete (because of insufficient amount of coupling agent with resultant air pockets, or when the one side of the ultrasound transducer is lifted), there will be drop-out areas (Fig. 7.15). Another reason for loss of image is insufficient depth or gain (Fig. 7.6), or usage of a high frequency ultrasound transducer for imaging deep structures (Fig. 7.3). When the impact angle of the ultrasound beam is different from 90°, the reflected waves may not reach the transducer (Fig 7.2). The ultrasound beam is extremely thin. In order to visualize longitudinally another thin structure (i.e. a needle or a nerve), the beam has to be perfectly aligned to it. This skill is difficult to master and requires a lot of practice. Minimal rotation or tilting of the transducer leads to loss of the image (Fig. 7.16).
Image resolution poor. Too much gain can cause all the tissues to appear hyperechoic and blur the differences between them (Fig. 7.6). Appropriate manipulation of the gain control will improve the image quality. Using a higher frequency ultrasound transducer may improve image resolution further (Fig. 7.3).
Bayonet artifact. Sometimes a transducer may overlie two adjacent areas that allow ultrasound to travel with different speeds. A needle passing through these two areas may visualize as broken because the ultrasound waves passing through the ‘higher-velocity’ tissue will reach the transducer faster and will give the impression that they come from a more shallow layer than the waves coming from the ‘lower-velocity’ tissues (Fig. 7.17).
Reverberation. Reverberation occurs when repeated reflection occurs between two strongly reflective surfaces (Fig. 7.18). Instead of a single reflected wave there is a series of waves reaching the receiver one after another, giving the false impression of multiple hyperechoic layers in the scanned area (Fig. 7.18). If this occurs, the operator should try to reduce the gain.
Posterior enhancement occurs when scanning over a fluid filled space (i.e. a cyst). The tissue immediately under this space may appear hyperechoic due to the significant reflection at the tissue interface and the good conductance of the reflected waves through the fluid back towards the transducer (Fig. 7.19). Care needs to be taken interpreting sonoanatomy here.
Posterior shadowing can be seen as a dropout of signal behind tissues that poorly conduct ultrasound (i.e. bone or air filled spaces like bowels) (Fig. 7.11).
Ergonomics
When performing ultrasound-guided nerve blocks, the operator, the needle transducer interface, and the ultrasound screen should be in one line if possible. The angle of the display should be adjusted for optimal visibility (Fig. 7.20). The configuration has to provide maximal comfort and stability for the patient as well as the operator. Both hands of the operator (the scanning and the needling hand) should rest on the patient to ensure stability and thus optimal control (Fig. 7.21). The necessary procedural equipment needs to be within easy reach and an assistant is desirable.
Scanning technique and anatomical survey
General considerations
Transverse (short axis, cross-sectional) scanning involves orientating the transducer perpendicular to the long axis of the anatomical structure of interest (Fig. 7.7). When using short axis views, the identification of nerves and the confirmation of the spread of local anesthetic is facilitated (Fig. 7.22). Tilting the ultrasound transducer can dramatically improve the image (Fig. 7.13), while slight tilting or sliding usually does not cause loss of the target (unlike when using longitudinal scanning).
Orientating the transducer parallel to the long axis of the anatomical structure of interest gives a longitudinal (long axis) view. This sometimes can be helpful to confirm that a structure is a nerve (Fig 7.14). This scanning orientation is more difficult as the ultrasound transducer and anatomical structure have to be perfectly aligned in order to produce an image of the structure.
Scanning technique
The basic movements when manipulating the transducer are sliding, rotation and tilting.
Sliding allows one to position the transducer over the area of interest. It encompasses moving the transducer on the proximal/distal or medial/lateral axis (Fig. 7.23).
Following this, the depth of imaging should be adjusted, thus enlarging the target while keeping the necessary landmark structures in view. Consider adjusting the gain and focus. Next, one can rotate the transducer to achieve optimal orientation (short or long axis) against the object of interest (Fig 7.23). The latter should be in the centre of the screen. The last step is tilting (Fig 7.23). These maneuvers are important in needle identification.
Anatomical survey and target identification
Clinical pearls
Once you have done some theoretical study you should practice scanning in logical steps.
Practicalities of ultrasound-guided regional anesthesia
Point of entry and depth. Ultrasound helps selection of needle entry and outlines depth required. The point of needle entry should be a distance of 1 cm from the ultrasound transducer. This facilitates asepsis and the free hand technique of needle intervention. Ultrasound can also be used to facilitate blocks without guidance. Here ultrasound is used to identify anatomy and provide valuable information, such as depth of target structures. For example, one can visualize the position of the spinous and transverse processes and measure the depth of the epidural and the paravertebral spaces (Fig. 7.24).
Needle visualization. The key to visualizing needles in long axis is perfect alignment of ultrasound beam and needle. The ultrasound beam is narrow and thus practice cannot be replaced. Never advance the needle without visualization. Adjust the transducer (sliding, tilting, rotation, pressure) rather than the needle, as this is safer and causes less discomfort for the patient. The more parallel the needle is to the ultrasound beam, the easier it is to see. Beam steering and compound imaging may be of help. Large bore needles visualize better. The industry is working on solutions to improve needle shaft and needle tip visibility (e.g. echogenic coating). Mechanical needle guides help to maintain the transducer – needle alignment (Fig. 7.25). A free hand technique rather than the use of needle guides is favored, due to the greater flexibility it allows.
Local anesthetic spread. Proper placement of local anesthetic is paramount to achieve fast and sufficient nerve block. The solution should encircle the nerve, thus outlining it (the donut sign) (Fig. 7.22). Avoid injecting air bubbles, as these cause acoustic shadowing and deteriorate the image (Fig. 7.26).
Wind K, Smith H, Jacob A, et al. Ultrasound machine comparison: an evaluation of ergonomic design, data management, ease of use, and image quality. Reg Anesth Pain Med. 2009;34:349-356.
Sites B, Brull R, Chan V, et al. Artifacts and pitfall errors associated with ultrasound-guided regional anesthesia. Part I: Understanding the basic principles of ultrasound physics and machine operations. Reg Anesth Pain Med. 2007;32:412-418.
Sites B, Brull R, Chan V, et al. Artifacts and pitfall errors associated with ultrasound-guided regional anesthesia. Part II: A pictorial approach to understanding and avoidance. Reg Anesth Pain Med. 2007;32:419-433.
Manickam B, Perlas A, Chan V, Brull R. The role of a preprocedure systematic sonographic survey in ultrasound-guided regional anesthesia. Reg Anesth Pain Med. 2008;33:566-570.
Hopkins R, Bradley M. In-vitro visualization of biopsy needles with ultrasound: a comparative study of standard and echogenic needles using an ultrasound phantom. Clinical Radiology. 2001;56:499-502.
Chin K, Perlas A, Chan V, Brull R. Needle visualization in ultrasound-guided regional anesthesia: challenges and solutions. Reg Anesth Pain Med. 2008;33:532-544.
Cheung S, Rohling R. Enhancement of needle visibility in ultrasound-guided percutaneous procedures. Ultrasound in Med & Biol. 2004;30:617-624.
Sites B, Chan V, Neal J, et al. The American Society of Regional Anesthesia and Pain Medicine and the European Society of Regional Anaesthesia and Pain Therapy Joint Committee recommendations for education and training in ultrasound-guided regional anesthesia. Reg Anesth Pain Med. 2009;34:40-46.