Chapter 31 Primary, Double, and Triple Varus Knee Syndromes
Diagnosis, Osteotomy Techniques, and Clinical Outcomes
INDICATIONS
The predominant indication for HTO is lower limb osseous malalignment (Fig. 31-1) in younger patients who have medial tibiofemoral joint pain and wish to maintain an active lifestyle. The goal is to correct the mechanical abnormality of excessive loading of the medial tibiofemoral compartment by redistributing weight-bearing loads onto the lateral compartment. Varus malalignment is present when the weight-bearing line (WBL) crosses less than 50% of the mediolateral transverse width of the tibial plateau.
Unfortunately, a prior medial meniscectomy is a major risk factor for progression of arthritis in these knees. Because any underlying arthritis is expected to progress, it is advisable to perform HTO while the joint damage is in the early stages before the development of severe articular cartilage deterioration and loss of tibiofemoral joint space.52,113
Lower limb varus malalignment in these knees is not overcorrected to valgus when there is no damage to the articular cartilage in the medial tibiofemoral compartment. The goal in these knees is to correct the varus to a neutral alignment and then proceed in a staged manner (if required) with a cruciate and posterolateral reconstruction as required. Correction of the varus alignment decreases the risks of failure of the ligament reconstructive procedures.96,98,99,101
CONTRAINDICATIONS
Major concavity of the medial tibial plateau with loss of bone stock is a contraindication to HTO. On standing 45° posteroanterior (PA) radiographs,121 knees that demonstrate no remaining articular cartilage space to the medial compartment are not candidates. An arthroscopic procedure just before HTO helps to assess the amount of remaining articular cartilage and remove symptomatic meniscus fragments and other tissues.
Critical Points CONTRAINDICATIONS
A relative contraindication is a body weight over 200 pounds (91 kg) (Fig. 31-2). Although there may be some patients in whom HTO is indicated who weigh up to 225 pounds (102 kg), this operation is avoided in patients with a higher body weight because the beneficial effect of unloading the medial compartment will not be achieved.28
A relative contraindication is increased medial slope to the affected medial tibial plateau in the coronal plane due to advanced medial plateau concavity.27 This finding indicates that it will not be possible to significantly unload the medial compartment with HTO, and the joint will remain with all of the weight-bearing confined to the medial compartment. This problem can be tested prior to surgery with varus-valgus stability tests at 30° knee flexion. In these knees with advanced medial arthritis, there is no neutral point in which there is simultaneous contact of the medial and lateral compartments. The tibia behaves like a teeter-totter, with contact alternating between the medial and the lateral compartment and obvious separation of the noncontacted compartment.
The issue of concurrent patellofemoral arthritis has been addressed by prior studies.28,83,123 In general, the symptomatic state should be addressed and the patient warned preoperatively that patellofemoral symptoms might continue or progress. Marked patellofemoral symptoms would contraindicate an HTO.123 The finding of asymptomatic articular cartilage changes to the patellofemoral joint is not a contraindication to HTO, because clinicians have noted that the end result in terms of longevity of the HTO depends on the symptomatic medial tibiofemoral compartment.28,68,93
LOWER LIMB ALIGNMENT: PRIMARY, DOUBLE, AND TRIPLE VARUS KNEES
Critical Points LOWER LIMB ALIGNMENT: PRIMARY, DOUBLE, AND TRIPLE VARUS KNEES
Primary varus: amount of varus angulation due to:
Double varus: amount of varus angulation due to:
The terms primary varus, double varus, and triple varus knee were devised to classify varus-aligned knees with associated ligament deficiencies (Table 31-1).93 This classification system is based on the underlying tibiofemoral osseous alignment and the additional effect of separation of the lateral tibiofemoral compartment (due to deficiency of the posterolateral structures) on the overall varus lower limb alignment, as calculated from the WBL.
The term primary varus refers to the physiologic tibiofemoral osseous angulation and any further increase in angulation owing to altered geometry (narrowing) of the medial osteocartilagenous tibiofemoral joint (Fig. 31-3). The tibiofemoral WBL shifts into the medial tibiofemoral compartment as the narrowing progresses and the lateral compartment is unloaded. Three degrees of varus angulation approximately doubles medial compartment pressures.36,46
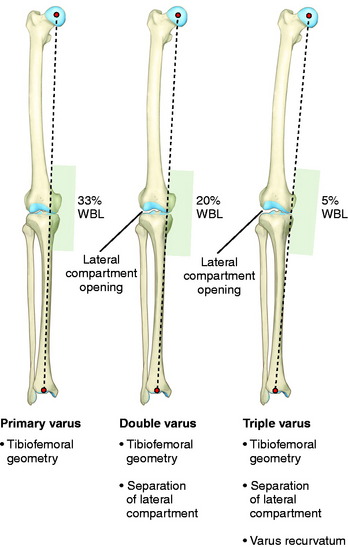
FIGURE 31-3 Schematic illustration of primary, double, and triple varus knee angulation. WBL, weight-bearing line.
As the WBL shifts into the medial compartment, there are increased tensile forces in the posterolateral soft tissues, including the iliotibial tract and ligament structures. There is corresponding separation of the lateral tibiofemoral compartment during standing, walking, and running activities (lateral condylar lift-off).75,125 This is called a double varus knee because the lower limb varus malalignment results from two factors: the tibiofemoral osseous and geometric alignment and separation of the lateral tibiofemoral compartment from deficiency of the posterolateral structures.
A combination of active and passive restraints resists separation of the lateral tibiofemoral compartment under dynamic loading conditions.45,86 The quadriceps, biceps femoris, and gastrocnemius muscles and iliotibial band act in a dynamic manner to resist adduction moments at the knee joint during gait and, with weight-bearing loads, resist lateral tibiofemoral separation. If these muscle forces do not provide a functional restraint to excessive lateral tensile forces, separation of the lateral tibiofemoral joint occurs.
In the triple varus knee, injury to the FCL and posterolateral structures produces a varus recurvatum position of the limb.54 The triple varus knee results from three causes: tibiofemoral varus osseous malalignment, increased lateral tibiofemoral compartment separation due to marked insufficiency of the FCL and PMTL, and varus recurvatum in extension. The varus recurvatum occurs because of abnormal external tibial rotation and knee hyperextension reflecting deficiency of the posterolateral structures and possibly the ACL. Owing to the increase in lateral compartment opening, the WBL shifts farther medially, as shown in Figure 31-3.
Eckhoff and coworkers36 reported important three-dimensional measurements in 90 individuals (180 limbs) and showed that there is considerable variation in coronal alignment between subjects and between right-left lower limbs (Fig. 31-4). One qualification to the data is that the hip-knee-ankle computed tomography (CT) measurements were obtained under non–weight-bearing conditions.
GAIT ANALYSIS
Although a high adduction moment may be anticipated as a result of varus malalignment, the moments and loads on the knee joint cannot be reliably predicted from the static measurement of lower limb alignment on radiographs.109 Many factors in patients with ACL deficiency, varus malalignment, and posterolateral deficiency can be assessed by gait analysis. Abnormal limb alignment, either varus or valgus in the coronal plane or hyperextension in the sagittal plane, produces substantial alterations in the moments and forces about the knee joint (Fig. 31-5).109 The analysis of external moments about the knee during gait allows the clinician to understand the effect of the altered gait dynamics on the knee joint. Abnormally high knee adduction moments increase the risk for progression of medial tibiofemoral arthritis owing to excessive loading.118 The success of HTO has been related to lowering these moments to below-normal values.118,137 In addition, gait analysis allows calculation of abnormally high tensile forces in the lateral soft tissue restraints that increase the risk of elongating these tissues from lateral condylar lift-off with activity. Abnormal tensile loads on posterolateral soft tissues preclude successful FCL and PMTL reconstruction. Markholf and associates76 reported that lateral tibiofemoral compartment loading lateral condylar lift-off had a marked effect on providing joint stability.
Authors’ Study Conclusions
ACL, anterior cruciate ligament; HTO, high tibial osteotomy.
Many studies have documented that the external moments about the knee joint and the corresponding tibiofemoral compartment loads are markedly influenced by individual gait characteristics and adaptations that occur after injury.5,8–11,16,23,62,85,118,137 ACL deficiency may produce marked abnormalities in moments about the knee joint in the sagittal plane, which is further affected by lower limb varus malalignment. Patients with ACL deficiency may show a decrease in the magnitude of the external flexion moment (quadriceps-reduced gait) or an increase in the external extension moment (hamstrings-protective muscle force).6,7 These effects are discussed in Chapter 6, Human Movement and Anterior Cruciate Ligament Function: Anterior Cruciate Ligament Deficiency and Gait Mechanics. The alignment of the foot markedly influences the knee adduction moment. Patients with toe-in, or less than normal external axial rotation of the foot during stance phase, tend to have a higher knee adduction moment as the WBL passes farther medial to the knee joint.4
Gait analyses were conducted in a study at the authors’ institution109 involving 32 patients with ACL deficiency and varus angulation. A force plate and an optoelectronic system were used to measure forces and moments of the lower limb and knee joint. Knee joint loads and ligament tensile forces were calculated using a previously described mathematical model.125 Sixty-two percent of the patients had an abnormally high magnitude of the moment, tending to adduct the affected knee (Fig. 31-6). The calculated medial tibiofemoral loads were excessively high in 66% of the patients (P < .01). Forty-seven percent of the patients had predicted abnormally high lateral ligament tensile forces (P < .05). The adduction moment showed a statistically significant (P < .05) correlation to predicted high medial tibiofemoral compartment loads and high lateral ligament tensile forces (P < .01). A shift had occurred in the center of maximal joint pressure to the medial tibiofemoral compartment, with a corresponding increase in the lateral ligament tensile forces to achieve frontal plane stability (Figs. 31-7 and 31-8). If muscle forces are not sufficient to maintain lateral tibiofemoral compressive loads, tensile forces develop in the lateral ligament tissues. The data indicate that, in knees with high lateral ligament tensile forces, separation of the lateral tibiofemoral joint occurs with “condylar lift-off” during weight-bearing.
The magnitude of the flexion moment (which is related to quadriceps muscle force) was significantly lower in 47% of the patients (P < .05), and the extension moment (related to hamstring muscle force) was significantly higher in 50% (P < .05). These findings indicated that a gait adaptation occurred that diminished quadriceps muscle activity and enhanced hamstring muscle activity hypothesized to provide anteroposterior stability of the knee joint.16
The authors99 studied patients with varus-angulated knees with insufficient posterolateral structures in whom prior posterolateral reconstructive procedures failed and an HTO was required before further soft tissue reconstructive procedures could be done. One explanation for these clinical findings is that these knees had a varus or hyperextension thrust during the stance phase of gait, which placed undue tensile forces on the deficient posterolateral structures (Fig. 31-9). Untreated varus malalignment has also been identified as a predisposing cause of failure of ACL reconstructions98,101 and posterior cruciate ligament (PCL) reconstructions96 as well.
CLINICAL EVALUATION
Subjective and Functional Outcome
Patients complete questionnaires and are interviewed for the assessment of symptoms, functional limitations, sports and occupational activity levels, and their perception of the overall knee condition according to the Cincinnati Knee Rating System (see Chapter 44, The Cincinnati Knee Rating System).13
Symptoms of pain, swelling, and giving-way are well-recognized consequences of ACL-deficiency.108 However, in the knee with combined varus malalignment and ACL deficiency, several different knee subluxations may produce symptoms of instability. These include anterior subluxation of the tibia, separation of the lateral tibiofemoral compartment on walking (varus thrust), posterior subluxation of the lateral tibial plateau (with knee flexion and external tibial rotation), and excessive hyperextension or varus recurvatum with a back-knee or feeling of the knee joint going into hyperextension. By history and asking the patient to demonstrate the knee instability, the surgeon must carefully determine the subluxations present.
Physical Examination
A complaint of medial joint line pain may or may not correlate with the degree of medial compartment articular cartilage damage.50,58 In the early stages, the patient usually complains of medial pain that occurs with sports activities, but not with daily activities. When pain occurs with daily activities, it is highly likely that extensive damage exists to the joint articular cartilage. Loss of the medial meniscus is the major risk factor for the progression of arthritis in the medial compartment.37
The physical examination of the knee joint to detect all of the abnormalities in the varus-angulated knee is comprehensive (Table 31-2) and includes assessment of (1) the patellofemoral joint, especially possible extensor mechanism malalignment due to increased external tibial rotation and posterolateral tibial subluxation; (2) medial tibiofemoral crepitus on varus loading, indicative of articular cartilage damage even if not visible on radiographs; (3) pain and inflammation of the lateral soft tissues due to tensile overloading; (4) gait abnormalities (excessive hyperextension or varus thrust) during walking and jogging104; and (5) abnormal knee motion limits and subluxations compared with the contralateral knee.106
Abnormality | Diagnostic Test |
---|---|
Tibiofemoral alignment | Full-length standing radiograph: double support (closure of lateral tibiofemoral joint required). |
Narrowing of medial tibiofemoral joint | Change in millimeters from opposite side on weight-bearing 45° posterior on stress radiograph. |
FCL insufficiency | Increase in lateral joint opening at 30° of flexion. |
FCL, PMTL, PL capsule insufficiency |
FCL, fibular collateral ligament; PL, posterolateral; PMTL, popliteal muscle-tendon-ligament unit.
From Noyes, F. R.; Simon, R.: The role of high tibial osteotomy in the anterior cruciate ligament–deficient knee with varus alignment. In DeLee, J. C.; Drez, D. (eds.): Orthopaedic Sports Medicine Principles and Practice. Philadelphia: W. B. Saunders, 1994; pp. 1401–1443.
Diagnostic Clinical Tests
The medial posterior tibiofemoral step-off on the posterior drawer test is done at 90° of flexion (Fig. 31-10). This test is performed first to identify that the tibia is not posteriorly subluxated, indicating a partial or complete PCL tear. A KT-2000 arthrometer test may be done at 20° of flexion (134 N force) to quantify total anteroposterior (AP) displacement. The Lachman test is performed at 20° of knee flexion. The pivot shift test is done and the result recorded on a scale of 0 to 3, with a grade of 0 indicating no pivot shift; grade 1, a slip or glide; grade 2, a jerk with gross subluxation or clunk; and grade 3, gross subluxation with impingement of the posterior aspect of the lateral side of the tibial plateau against the femoral condyle.
FCL insufficiency is determined by the varus stress test at 0° and 30° of knee flexion (see Fig. 31-10). The surgeon estimates the amount of joint opening (in millimeters) between the initial closed contact position of each tibiofemoral compartment, performed in a constrained manner avoiding internal or external tibial rotation, and the maximal opened position. The result is recorded according to the increase in the tibiofemoral compartment of the affected knee compared with that of the opposite normal knee. This comparison is crucial, and it is important to avoid measuring only the degrees of varus or valgus rotation in the involved knee.
An increase in medial joint opening may occur compared with the opposite knee that represents a pseudolaxity, because the increase is actually due to medial tibiofemoral joint narrowing. When the test is conducted under a varus stress, the medial joint opening returns the limb to a more normal alignment, and there is no true medial ligamentous damage. The true amount of medial and lateral tibiofemoral compartment opening is later confirmed during the arthroscopic examination with gap tests. The primary and secondary restraints that resist lateral joint opening have been described previously.45 The abnormal medial joint opening depends on the knee flexion angle at which the test is conducted and the integrity of the secondary restraints.
Tibiofemoral Rotation Test
The tibiofemoral rotation test was first described by the senior author103 and is used to estimate the amount of posterior tibial subluxation (see Fig. 31-10). The test is conducted in the following manner: (1) the tibia is positioned at 30° of knee flexion, in neutral rotation, (2) the position of the anterior aspect of the medial and lateral tibial plateaus are determined in reference to the femoral condyles by palpation, (3) the tibia is externally rotated to its maximum position, (4) the positions of the medial and lateral tibial plateaus are palpated to determine an abnormal posterior subluxation of the lateral compartment or anterior subluxation of the medial compartment, (5) the examiner observes the location of the tibial tubercle to determine any increase in external tibial rotation compared with the opposite normal knee, and (6) the test is repeated at 90° of knee flexion and may also be conducted by starting at the neutral tibial rotation position and progressing to internal tibial rotation.
Critical Points CLINICAL EVALUATION
Assessment of symptoms and functional limitations with activity: Cincinnati Knee Rating System
Tibiofemoral Rotation (Dial Test)
The advantages of the tibiofemoral rotation test over the traditional posterolateral drawer test55 are (1) the knee may be positioned at varying flexion positions (30° and 90°); (2) the tibia is less constrained because the foot is not held fixed to the examining table; and (3) the axis of tibial rotation can be observed as the tibia is rotated externally and internally. More information is gained when the tibiofemoral rotation tests are performed with the patient in a supine position. In the prone position, it is difficult to palpate the medial and lateral tibiofemoral position required to diagnose the abnormal compartment subluxations. The only indication for the prone dial test is a PCL-deficient knee in which the tibia can be gently displaced to a reduced anterior position during the rotation tests. In the supine position, the tibia can also be displaced anteriorly to prevent posterior subluxation, which makes the interpretation of the dial test more difficult.
Radiographic Assessment
Radiographic assessment of lower limb alignment is based on full-length radiographs of the extremity with the patient in the standing position.35 Separation of the lateral tibiofemoral compartment may occur, preventing a correct assessment of true tibiofemoral osseous alignment. Double-stance, full-length AP radiographs showing both lower extremities from the femoral heads to the ankle joints are obtained. The knee is flexed 3° to 5° to avoid a hyperextension position. If separation of the lateral tibiofemoral joint is observed, it is necessary to subtract the lateral compartment opening so that the true tibiofemoral osseous alignment is determined and a valgus overcorrection at surgery is avoided, as is discussed under Preoperative Planning.
Additional radiographs taken during the initial examination include a lateral at 30° knee flexion, weight-bearing PA at 45° knee flexion, and patellofemoral axial views. Telos medial or lateral stress radiographs may also be required of both knees (Fig. 31-11).
The height of both patellas is measured on lateral radiographs (Fig. 31-12) to determine whether an abnormal patella infera or alta position exists that may be a factor in selecting an opening or closing wedge osteotomy, which would further decrease or elevate the patella position.111,112
The clinical algorithm for the preoperative clinical and radiographic assessment to determine candidates for either a medial opening wedge or a lateral closing wedge osteotomy is shown in Figure 31-13.
PREOPERATIVE PLANNING
Calculations to Determine Angular Correction
The preoperative calculations for HTO involve precise measurements to determine the amount of angular correction desired to redistribute tibiofemoral forces while not altering tibial slope and tibiofemoral joint obliquity in the frontal plane.35,107 The normal tibiofemoral coronal alignment is shown in Figure 31-14. In varus angulated knees, there is a slight increase in obliquity due to medial tibiofemoral joint arthrosis principally involving the medial condyle. An opening wedge femoral osteotomy restores the joint obliquity. The more difficult issue related to joint obliquity involves correcting a valgus malalignment in which a change in joint obliquity is common. An opening wedge osteotomy corrects the joint obliquity, whereas a closing wedge tibial osteotomy increases joint obliquity and therefore is contraindicated.
Critical Points PREOPERATIVE PLANNING
Sabharwal and Zhao124 compared lower limb coronal alignment on full-length, standing radiographs with supine intraoperative hip-knee-ankle fluoroscopy measurements in 102 limbs. There was a statistically significant mean difference between the two techniques (mean, 13.4 mm deviation in the WBL, measured from the center of the joint). This difference in the two techniques is of concern in the preoperative planning and the final intraoperative osteotomy correction in the coronal plane. The reader should note that there was no description of accounting for the effect of abnormal lateral joint opening on the standing radiographs that would account for errors on full-length, standing radiograph preoperative measurements, as previously discussed. Further, at surgery there was no description of the fluoroscopy technique. The surgeon must verify medial and lateral tibiofemoral joint contact to account for possible opening of the medial or lateral tibiofemoral joint during the WBL measurement. The fluoroscopy technique involves the surgeon placing an axial load against the foot to overcome medial or lateral joint opening, which is verified by the fluoroscopic image. The lower extremity should be held in 10° of knee flexion and approximately 10° to 15° of external rotation (hip to ankle axial alignment simulating the foot progression angle). The limb alignment rod at the knee joint can be observed to move medial to lateral with internal to external limb rotation, which is another source of error in measuring the WBL intersection at the tibia. This is because the alignment rod is placed anterior to the true center of axial rotation at the ankle joint, and therefore, lower limb rotation induces this error. One advantage of the computerized navigation technique is that the WBL is centered through the hip-knee-ankle joints whereas an alignment rod or cord used at surgery is placed anterior to these respective joints.
An example of the potential effect of the failure to account for increased lateral joint opening is shown in Figure 31-15. The case represents a 38-year-old man whose full-length, standing radiograph shows a varus angular deformity (mechanical axis) of 7° and a 4-mm increase in lateral joint opening. The width of the tibial plateau is 81 mm. The equation to determine the angular deformity resulting from the lateral tibiofemoral separation distance is
S, lateral joint opening space; TW, tibial width.
Failure to account for the lateral joint opening would result in approximately 4° of valgus overcorrection after HTO, assuming closure of the lateral tibiofemoral joint (Fig. 31-16). A WBL crossing the knee lateral to the 75% coordinate has the potential for a lift-off of the medial femoral condyle. Unicondylar weight-bearing resulting from distraction of the medial compartment is undesirable and could result in subsequent arthritis of the lateral compartment, medial collateral ligament (MCL) laxity, and a progressive valgus deformity (Fig. 31-17). The WBL-tibial intersection depends on the coronal mechanical axis angle and the femoral and tibial lengths of the patient; the senior author’s published study35 showed the WBL axis to be more accurate than the anatomic axis for osteotomy correction.
Two methods are used to determine the correction wedge on preoperative radiographs.35 First, the centers of the femoral head and tibiotalar joint (center of the talus) are marked on the full-length radiograph (Fig. 31-18). The desired correction in the WBL coordinate of the tibial plateau is identified and marked. This is usually placed at 62% of the tibial width (just at the down slope of the lateral tibial spine), which allows the WBL to pass through the lateral tibiofemoral compartment, providing a 2° to 3° angular valgus overcorrection. The 62% WBL coordinate is used if there is medial tibiofemoral articular cartilage damage and the intention is to transfer major loads to the lateral compartment. A 50% WBL coordinate is chosen if there is no medial tibiofemoral joint damage and the surgeon wishes to correct to a neutral axial alignment (e.g., prior to posterolateral reconstruction). One line is drawn from the center of the femoral head to the selected percent WBL intersection at the tibia, and a second line is drawn from the center of the tibiotalar joint to the same tibial coordinate position. The angle formed by the two lines intersecting at the tibia represents the angular correction required to realign the WBL through this coordinate. An alternative method was published35 in which the previously discussed femoral and tibial axis lines intersect at the hinge point of the tibial osteotomy. This technique ends up with a similar measurement for the angular correction. However, the surgeon does not have the measured WBL tibial intersection required for intraoperative fluoroscopy verification.
The second method of determining the correction wedge involves cutting the full-length, standing radiograph horizontally through the line of the superior osteotomy cut (Fig. 31-19). A vertical cut of the lower tibial segment (opening or closing wedge) is made to converge with the first cut. The distal portion of the radiograph is aligned until the center of the femoral head, the selected WBL coordinate point on the tibial plateau, and the center of the tibiotalar joint are all colinear. With the radiograph taped in this position, the angle of the wedge formed by the overlap of the two radiographic segments is measured and compared with the value obtained using the first method. The mechanical axis is measured to determine the angular correction. If there is a discrepancy between the two correction wedge angles, the procedures should be repeated.
Calculations to Determine Tibial Slope and Clinical Indications to Change Tibial Slope
Several methods have been reported to measure the medial and lateral tibial slope based on lateral tibial radiographs (Table 31-3).17,20,24,25,41,63,79,82,146 Each method results in different slope values for the same tibia (Fig. 31-20), and all studies show high standard deviations and ranges, indicating that tibial slope may show variation among individual patients. Brazier and colleagues20 measured 83 lateral knee radiographs and concluded that the methods of slope measurement of proximal tibial anatomic axis (PTAA) and posterior tibial cortex were more reliable than other methods. Genin and coworkers41 and Julliard and associates63 recommended using the tibial mechanical axis to represent the true tibial slope. The PTAA is the authors’ preferred method for HTO, and the anterior tibial cortex measurement is used when cutting the proximal tibia during total knee arthroplasty (TKA).
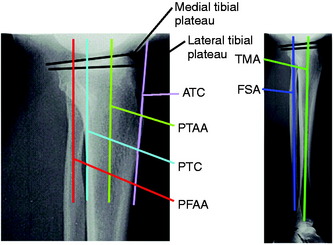
FIGURE 31-20 Some reported radiographic techniques to measure the tibial slope. The values obtained depend on the anatomic site selected on the tibia. Selected literature references for reported values are shown in Table 31-3. ATC, anterior tibial cortex; FSA, fibular shaft axis; PFAA, proximal fibular anatomic axis; PTAA, proximal tibial anatomic axis; PTC, posterior tibial cortex; TMA, tibial mechanical axis.
(Reprinted with permission from Noyes, F. R.; Goebel, S. X.; West, J.: Opening wedge tibial osteotomy: the 3-triangle method to correct axial alignment and tibial slope. Am J Sports Med 33:378–387, 2005.)
Most authors have measured the medial tibial slope; only a few have measured and provided data on the lateral tibial slope.79,146 The lateral tibial slope is less angled than the medial tibial slope. Few investigators have distinguished between the tibial plateau osseous slope and the change that would occur in slope if the articular cartilage and meniscus were added to the calculations. The osseous tibial slope is most commonly noted in the literature and is measured from sagittal radiographs. The true tibial slope, which includes the articular cartilage and meniscus, was noted to be 6° less than the osseous tibial slope, according to Jenny and colleagues.60
Increasing the tibial slope increases anterior tibial translation and, potentially, tensile loads on the ACL or an ACL reconstruction (Fig. 31-21).30 Conversely, decreasing the tibial slope theoretically would increase tensile loads on the PCL and shift the tibia to a more anterior position. Giffin and coworkers44 reported in cadaveric knees that an increase of 5° in the tibial slope produced an anterior shift in the resting position of the tibia (maximum, 3.6 ± 1.4 mm at full extension). However, under a 200-N axial load, there was only a 2-mm anterior shift in the tibiofemoral contact position (30° and 90°) and no increase in ACL forces. The authors concluded that small changes in tibial slope under simulated weight-bearing conditions would have little effect on tibiofemoral position. However, they postulated that larger changes in tibial slope, such as 10°, would cause a greater anterior shift in the tibia resting and loaded positions that might require correction by biplanar osteotomy in knees with ACL injury undergoing HTO.
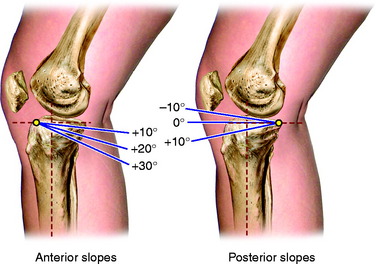
FIGURE 31-21 The effect of an increase in the tibial slope. The degree of tibial slope is based on proximal tibial anatomic axis (PTAA; Fig. 31-20).
In a second study conducted by Giffin and associates,43 a 5-mm anterior opening wedge osteotomy in PCL-sectioned cadaveric knees was performed and an anterior shift in the tibia resting position of approximately 4 mm was measured. The beneficial effect of an increase in tibial slope in a PCL-deficient knee should be viewed in terms of functional loading conditions, and it is questionable whether the resting no-load position data are applicable to in vivo loading conditions. In this study, under a combined 134-N tibial translation load and 200-N axial compressive load, there was no statistical difference in the amount of posterior tibial subluxation between the PCL-deficient and the PCL-deficient-osteotomy knees. The data show that the tibia reached a similar abnormal posterior tibial position (mean 90° AP translation: intact knee 10 mm; PCL-deficient, 20.6 mm; PCL-deficient-osteotomy, 20.3 mm). The authors concluded that “increasing the tibial slope would improve stability in the PCL-deficient knee”; however, this would be true only for conditions of no posterior tibial loading not expected in functional activities. The data in this study may be viewed from an opposite standpoint to indicate that PCL graft reconstruction is warranted in symptomatic knees, because the opening wedge osteotomy is not effective in preventing posterior tibial subluxation under posterior tibial loading conditions.
In cadaveric knees, Agneskirchner and colleagues2 studied the effect of an opening wedge osteotomy combined with a flexion osteotomy (to change the tibial slope) on tibiofemoral contact pressures and the resultant tibiofemoral position. The study reported that the flexion osteotomy shifted the tibiofemoral contact to a more anterior position (15° flexion osteotomy, ∼5 mm, 30° knee flexion), which “neutralized” the effect of sectioning the PCL and reduced pressures of the posterior half of the tibial plateau. The authors suggested in varus-angulated, PCL-deficient knees, with associated posterolateral knee ligament injury and knee hyperextension, that an associated change in tibial slope would have a beneficial effect. Only quadriceps-induced leg extension loading was used, without weight-bearing loads or posterior translational loads to determine the resultant tibiofemoral position under more physiologic loading conditions. The quadriceps loading (to resist the applied external flexion moment) changed with the quadriceps extension force, nearly doubling with the flexion osteotomy (without osteotomy, ∼550 N, with 15° flexion; with osteotomy, ∼1100 N quadriceps applied load). The increased quadriceps loading in fact increased joint contact pressures.
In contrast to the study just discussed, Rodner and coworkers120 reported in cadaveric knees that an increase in tibial slope in the ACL-deficient knee at the time of a HTO has the potential to redistribute tibiofemoral contact pressures to a more posterior position on the tibial plateau. From a theoretical standpoint, a redistribution of pressures posterior on the tibial plateau would be detrimental to the long-term success of an HTO in ACL-deficient knees with meniscectomy and posterior tibial plateau articular damage. The cadaver knee studies involved uniaxial loading knee joint loads instead of the quadriceps loading in the study by Agneskirchner and colleagues,2 thereby demonstrating the marked effect that in vitro experimental loading conditions have on joint contact pressures and study conclusions.
Brandon and associates19 measured the tibial slope in 100 ACL-deficient patients and 100 controls and reported that female and male patients had similar tibial slope measurements. ACL-deficient female and male patients had increases in tibial slope (mean values increased 3.6° and 2.4°, respectively), and the high-grade pivot-shift patient group had an increase in tibial slope (mean value increased ∼2°). The measurements show high standard deviations (∼35% of the mean values), and it is probable that the few degrees difference between patient groups is not clinically significant or would result in increased ACL load and anterior tibial displacement.
Dejour and colleagues32 evaluated chronic ACL-deficient knees with monopodal lateral weight-bearing films (knee flexed 20° to 30° with the quadriceps contracted) and compared the anterior tibial translation with the tibial slope. The tibial slope was defined as the angle between the medial tibial plateau and the long axis of the tibia, which had an average value of 6°. The anterior tibial translation was defined as the distance between a parallel line at the posterior medial tibial plateau and a posterior point on the medial femoral condyle. The authors reported a statistically significant relationship between the standing anterior tibial translation and the tibial slope in both normal and chronic ACL-deficient knees. The regression line showed a 6-mm increase in anterior tibial translation for every 10° increase in tibial slope. However, it should be noted that a wide variation was present in the data between knees. For example, in chronic ACL-deficient knees with a tibial slope of 10°, anterior tibial translation varied from approximately –2° to 12°.
Griffin and Shannon42 recently summarized the clinical role of HTO with knee ligament instability, showing the benefit of an anterior opening wedge HTO for severe recurvatum and correcting an abnormal increased tibial slope in select patients who had failed multiple ligament reconstructions.
Marti and coworkers77 recommended that knees with ACL insufficiency and associated medial arthritis with a tibial slope of 10° or more undergo correction at the time of a varus-producing osteotomy. However, no clinical data were provided to demonstrate whether a change in slope decreased instability symptoms. Other authors33 discussed increases in ACL tensile loads when tibial slope exceeded 10° and postulated the need for correction, again without clinical data.
Griffin and Shannon42 noted that the anterior tibial subluxation with ACL insufficiency results in a “copula” owing to increased damage of the medial plateau; however, the effect of a medial meniscectomy on producing the same effect should be considered.
The authors of this chapter agree with recommendations to correct tibial slope when a distinct abnormality is present (Figs. 31-22 and 31-23). The published literature does not provide objective data regarding the degrees of abnormal increase or decrease in tibial slope when corrective osteotomy is indicated when there is an associated ACL or PCL insufficiency.
A normal tibial slope should not be increased in ACL-deficient knees or decreased in PCL-deficient knees undergoing a varus-producing opening wedge osteotomy. The rule is described elsewhere in this chapter (Opening Wedge Osteotomy: Preservation of Tibial Slope) that the anterior gap at the medial opening wedge should be one half of the posteromedial gap to maintain a normal tibial slope.105 For every 1 mm of anterior gap change, an approximately 2° change in tibial slope would be produced. This is based on the angle of the anteromedial tibial cortex, tibial width, and the AP distance where the gap measurement is made (see Table 31-5). It is common in opening wedge osteotomies to incorrectly produce a symmetrical anterior and posterior gap at the osteotomy site, which results in a considerable increase in the tibial slope, potentially leading to a decrease in knee extension and an increase in ACL tensile loads. For example, a change in the tibial slope by 10° would result if an anterior gap measurement error of only 5 mm occurred. The final tibial slope is verified at surgery by lateral fluoroscopic views.
If the surgeon elects to decrease the tibial slope, a staple may be placed at the tibial tubercle while the osteotomy is carefully closed anteriorly to the desired amount. In the senior author’s experience, biplanar corrections require the use of a more secure locking plate design to maintain the correction, because it is necessary to section most of the lateral tibial cortex hinge to close the anterior osteotomy gap (Fig. 31-24). Caution is required when closing the anterior osteotomy gap to apply only gentle hyperextension pressure on the tibia. If any resistance is obtained, it is necessary to further weaken the posterolateral tibial cortex (guide pin penetration) to prevent a lateral tibial plateau fracture just anterior to the remaining posterolateral cortex.
Song and associates127 reported that the normal tibial slope would be maintained if the gap or millimeters of opening along the anteromedial cortex of the anterior plate was two thirds the gap at the location of the posterior plate. The reader should note that the authors are referring to the location of two plates placed along the anteromedial tibial cortex and not the most posterior and anterior gap of the tibial opening wedge discussed in the Noyes and colleagues’ study105 (anterior gap 50% of posterior gap). The data of the Noyes and colleagues’ study,105 shown in part in Tables 31-6 and 31-7, allows the gap to be computed along the entire anteromedial cortex. The Song and associates’ study127 does not specify where the two plates are placed, and the data in Table 31-7 are more precise to show the actual gap distance where one or two plates would be placed. Further, if the two thirds gap rule of Song and associates were mistakenly applied to the true anterior and posterior gaps along the opening wedge, an increase in tibial slope would occur.
Hohmann and coworkers51 retrospectively reviewed the radiographs of 67 patients who had a closing wedge HTO and reported a mean decrease of 4.9° in the tibial slope. They suggested that this occurred because of the triangular geometry of the tibia, with more bone removed than required from the anterolateral portion of the lateral tibia.
Timing of Procedures
The timing of HTO and ligament reconstructive procedures is based on several factors (Table 31-4 and Fig. 31-25). In primary varus knees that do not demonstrate abnormal lateral joint opening and external tibial rotation, the HTO and cruciate reconstruction may be performed at the same setting.93 In double and triple varus knees, the ligament reconstructive procedures are performed after the HTO to reduce the risk of complications.100 Prolonged rehabilitation and an increased risk of knee arthrofibrosis and motion problems have been described after HTO and ligament reconstructive procedures are performed simultaneously.18,69,133
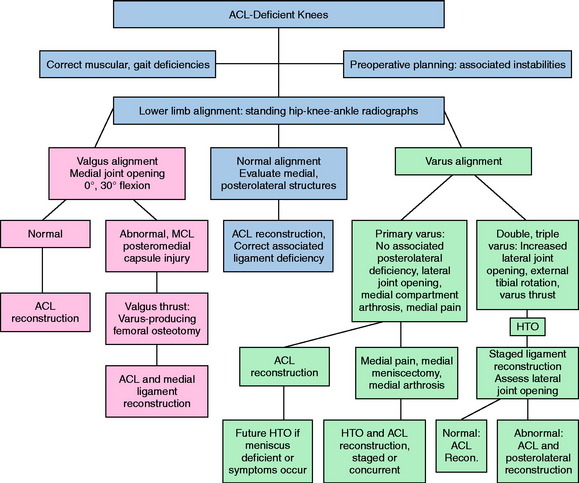
FIGURE 31-25 Timing of HTO and knee ligament reconstructive procedures.
(Reprinted with permission from Noyes, F. R.; Barber-Westin, S. D.: Revision anterior cruciate surgery with use of bone–patellar tendon–bone autogenous grafts. J Bone Joint Surg Am 83:1131–1143, 2001.)
The authors prefer to perform the HTO first and, after adequate healing of the osteotomy, the required ligament reconstructive procedures. In ACL-deficient double varus knees, an increase in the lateral tibiofemoral joint gap test contraindicates ACL reconstruction. HTO is effective in decreasing abnormal loads in the lateral and posterolateral tissues, allowing physiologic remodeling and shortening to occur, particularly when the deficiency of the posterolateral structures is only moderate (5–8 mm increased lateral joint opening, 10° increased external tibial rotation).93,100 In a study conducted by the authors,93 described later in this chapter (Authors’ Clinical Studies), 22 of 41 double varus knees (54%) had abnormally increased lateral joint opening on varus stress testing before osteotomy. Of these, only 5 (12%) had this abnormal joint opening at follow-up. A FCL and posterolateral reconstruction was avoided in these patients. In this same study, a subgroup of 11 patients (27%) did not require ACL reconstruction, because instability symptoms did not exist after the HTO.93
Cruciate Graft Options
A bone–patellar tendon–bone (B-PT-B) autograft is preferred if there is gross anterior subluxation (grade III pivot shift). In knees undergoing revision ACL reconstruction in which the ipsilateral patellar tendon was previously harvested, the contralateral B-PT-B graft or the ipsilateral quadriceps tendon–patellar bone (QT-PB) graft is a preferred substitute.94,98 However, care must be taken when harvesting these autografts from the same knee at the time of the osteotomy. The opposite knee may be used for graft harvest.
The use of allografts for ACL reconstruction is controversial owing to the higher graft stretch-out and failure rates compared with B-PT-B autografts, as discussed in detail in Chapter 7, ACL Primary and Revision Reconstruction: Diagnosis, Operative Techniques, and Clinical Outcomes.90–92,101 If an allograft is required, the B-PT-B has an advantage of more secure bone fixation.
Graft preferences for PCL reconstruction are a two-strand QT-PB autograft or allografts, further described in Chapter 21, Posterior Cruciate Ligament: Diagnosis, Operative Techniques, and Clinical Outcomes.95,97 The options for surgical restoration of deficient posterolateral structures are described in Chapter 22, Posterolateral Ligament Structures: Diagnosis, Operative Techniques, and Clinical Outcomes.
Opening versus Closing Wedge Osteotomy
Several opening wedge plate designs are available. The authors prefer a tibial plate with locking screws to achieve secure osseous fixation.129 The reader is referred to the appropriate literature by the respective manufacturer, because each locking plate design is different and the recommended technique must be followed. There are rules that must be followed to avoid changing the tibial slope with the use of any fixation plate. A greater chance of increasing the posterior tibial slope exists if an opening wedge buttress plate is placed in an anterior position.21 With attention to this problem at surgery, the normal tibial slope in the sagittal plane can be preserved with any of the available plate designs.105
Brouwer and associates22 conducted a prospective clinical trial in 92 patients randomized to either an opening wedge (Puddu plate) or a closing wedge osteotomy. At 1 year postoperatively, a valgus alignment (defined as 0°–6° valgus) was noted in 79% of the closing wedge osteotomies compared with 56% of the closing wedge osteotomies. The authors concluded that the Puddu plate had insufficient strength to maintain the operative correction. The investigation had several flaws because it involved four surgeons and hip-knee-ankle radiographs were not obtained in the initial postoperative period. The number of opening wedge osteotomies that received autogenous bone grafting was not provided. The results emphasize the importance of determining that the appropriate valgus correction is achieved at surgery and maintained in the early postoperative period until union has occurred. The failure to achieve the desired valgus alignment would be expected to decrease long-term success rates. Full-standing weight-bearing hip-knee-ankle radiographs may be obtained by 3 to 4 weeks postoperative after either opening or closing wedge osteotomy to verify that correction has been achieved. In a small percentage of cases in which the correction is found not to be ideal on early postoperative radiographs, a revision angular correction may then be performed.
The use of an external fixator has been advocated with tibial osteotomy, with the advantage of allowing adjustments in lower limb alignment that is helpful in difficult or biplanar corrections.130,139 A disadvantage of an external fixator is the risk of pin tract infection74,130,139 and the 12-week period of time that the fixator is typically used. The use of an external fixator is ideal when both coronal lower limb alignment and tibial rotation need to be corrected.
Opening Wedge Osteotomy: Preservation of Tibial Slope
A study was conducted using three-dimensional analysis of the proximal tibia to determine how the angle of the opening wedge along the anteromedial tibial cortex influences the tibial slope (sagittal plane) and valgus correction (coronal plane) when performing a medial opening wedge osteotomy.105
The data in this study on the obliquity of the anteromedial cortex of the proximal tibia relative to the posterior tibial cortex (Fig. 31-26) are presented from 35 magnetic resonance imaging (MRI) films in healthy young individuals (mean age, 32.7 yr).105 Serial CT images of a cadaveric tibia were made in 1.25-mm slices and digitized using a computer-aided design package to create a solid model of the proximal tibia.
A medial opening wedge osteotomy was created along the AP axis to include a hinge axis on the lateral margin of the tibia. The open wedge osteotomy was just proximal to the tibial tubercle, ending 20 mm below the cortical margin of the lateral tibial plateau. The distal portion of the tibia was rotated on the virtual model about the hinge axis through the lateral point of the osteotomy, maintaining the anatomic tibial slope (sagittal plane). Measurements of the width of the wedge angle and gap angle (Fig. 31-27) along the anteromedial tibial cortex were made from the resulting computerized model. Standard algebraic calculations were made using the law of triangles to determine the effect of different degrees of opening wedge osteotomy on coronal (valgus) and sagittal (tibial slope) alignment.
The MRI measurement of the anteromedial tibial cortex oblique angle at the site of the opening wedge osteotomy was 45° ± 6° (range, 34°–56°). The opening wedge angle, along the anteromedial tibial cortex to maintain the tibial slope, was found to be dependent on the angle of coronal valgus correction (HTO coronal angle) and the angle of obliquity of the anteromedial tibial cortex. In Figure 31-28, the results are shown for the calculation of the opening wedge angle (along the anteromedial tibial cortex) for five different osteotomy corrections in the coronal valgus plane (2.5°–12.5°).
The gap angle is perpendicular to the anteromedial oblique surface of the tibia with a vertex on the hinge axis posterior to the tibia. This is shown in Figure 31-29, in which the gap angle in degrees is shown for five different osteotomy corrections as a function of the obliquity of the anteromedial tibial cortex.
As an example, a 10° coronal valgus correction (assuming a normal 45° obliquity of the anteromedial tibial cortex) would result in a 7° gap angle at the osteotomy site. An error in the anteromedial tibial cortex opening wedge angle would result in an error in the tibial slope. An example of this is shown in Figure 31-30, which represents the desired wedge angle for a 10° coronal correction. An equal anterior and posterior opening or gap at the osteotomy site would result in an increased tibial slope and loss of normal knee extension.
These variables are shown in Table 31-5. For example, an error of 5 mm in the Y2 gap (Fig. 31-31) at the tibial tubercle, assuming the length of the anteromedial cortex (L) is 45 mm, would result in an unexpected change in the tibial slope of 10°. As a general rule in this example, every millimeter of alteration in the gap height induces a change of 2° in the tibial slope.
The opening wedge angle can be set at surgery by measuring and altering the vertical gap (width) at two points along the osteotomy site, Y1 and Y2 (Fig. 31-32). This has importance in determining that the correct wedge angle is obtained prior to internal fixation at the osteotomy site. The site at which the vertical gap measurement is taken depends on the coronal distance from the hinge axis, obliquity of the anteromedial tibial cortex, and the distance along the osteotomy site on the anteromedial surface (Tables 31-6 and 31-7).
In Table 31-6, the millimeters of opening at the osteotomy site are based on the width of the tibia and the angle of correction. In Table 31-7, an average 45° oblique angle of the anteromedial cortex and a tibial width of 60 mm are assumed. This allows the surgeon to calculate at the time of surgery the desired gap height at two points along the osteotomy to maintain the tibial slope.
TABLE 31-6 Opening at the Osteotomy Site (mm) Based on the Width of the Tibia and the Angle of Correction
For example, the measurement of the posterior tibial width is made at the osteotomy site (X1). The opening height at the most posteromedial point (Y1) and the distance between the two measurement points (Y1–Y2) are used in Table 31-7 to determine the second opening height at a defined distance (L) along the osteotomy site to maintain tibial slope.
If the tibial plate is placed just anterior to the superficial MCL on the anteromedial tibial cortex, approximately 20 mm anterior to the most posteromedial point of the osteotomy, the osteotomy gap at this site can be determined. In Table 31-7, with a 10-mm posteromedial opening (Y1), the correct width at the tibial plate would be 7.6 mm (tibial width, 60 mm).
For a 12-mm posteromedial opening, the correct width of the gap at the tibial plate would be 9.2 mm. A wider gap at the tibial plate would result in excessive valgus alignment and altered tibial slope. The change in the oblique angle of the anteromedial cortex, within the standard deviations reported, would have only a small and negligible effect on the width measurements of the opening wedge (see Table 31-7). To maintain the proper anterior and posterior gaps at the osteotomy site, appropriate distraction instruments at surgery are required to maintain the desired coronal and sagittal angular correction.
Final Recommendations to Obtain Correct Coronal and Slope Alignment
INTRAOPERATIVE EVALUATION
An operative “time-out” and identification of the operative limb by the surgeon and patient is performed as described in Chapter 7. All knee ligament subluxation tests should be performed after the induction of anesthesia in both the injured and the contralateral limbs. The amount of increased anterior tibial translation, posterior tibial translation, lateral joint opening, and external tibial rotation should be documented, as previously described.
A thorough arthroscopic examination should be conducted, documenting articular cartilage surface abnormalities110 and the condition of the menisci. The gap test is done during the arthroscopic examination.100 The knee is flexed to 30° and a varus load of approximately 89 N is applied. A calibrated nerve hook is used to measure the amount of lateral tibiofemoral compartment opening (Fig. 31-33). Knees that have 12 mm or more of joint opening at the periphery of the lateral tibiofemoral compartment will usually require a staged posterolateral reconstructive procedure.
OPERATIVE TECHNIQUE: OPENING WEDGE TIBIAL OSTEOTOMY
Step 2: Knee Arthroscopy
Arthroscopy is performed at the time of the HTO to evaluate articular surfaces of the medial and lateral tibiofemoral compartments and patellofemoral joint to confirm that the patient is an appropriate candidate for the osteotomy. ACL-deficient knees may have associated meniscus tears that require repair122 or partial removal. Appropriate débridement of tissues, inflamed synovium, and notch osteophytes limiting knee extension is performed. In particular, a secondary weight-bearing area is established in varus-angulated knees in which the lateral tibial spine produces a bone erosion over the inner margin of the lateral femoral condyle. The femoral condylar bone erosion may be débrided to remove the painful weight-bearing site.
Step 3: Anterior Iliac Crest Bone Graft Harvest
A 4-cm incision is made over the anterior iliac crest and deepened to the periosteum (Fig. 31-34), which is sharply incised and reflected medially only to the inner cortex. Laterally, meticulous subperiosteal dissection is carried along the outer table of the pelvis, avoiding the lateral muscles. The graft size is defined on the outer cortex using electrocautery. In most patients, the graft will be 40 mm in length, 10 mm in width, and 30 mm in depth. However, in smaller patients, the graft may be smaller in width and approximately 8 mm in depth. Patients undergoing large osteotomies may require a longer graft of approximately 45 mm to 50 mm.
Critical Points OPERATIVE TECHNIQUE OPENING WEDGE OSTEOTOMY
HTO, high tibial osteotomy; SMCL, superficial medial collateral ligament.
Step 4: Initial Anteromedial Tibial Approach
The operative technique is shown in Figure 31-35. A 5-cm vertical skin incision is made medially midway between the tibial tubercle and the posteromedial tibial cortex, starting 1 cm inferior to the joint line. A vertical incision is preferred over an oblique or transverse incision because vertical incisions are subsequently used in knees progressing to partial or complete TKA. The sartorius fascia is incised in line with its fibers, proximal to the gracilis tendon, exposing the distal SMCL attachment.
Step 5: Proximal Osteotomy and Placement of Tibial Guide Pins
A commercial guide system (Arthrex Opening Wedge Osteotomy System, Arthrex Inc., Naples, FL) may be used to facilitate guide wire placement (Fig. 31-36). A 2-mm guide pin is placed at the posteromedial cortex at the marked line and advanced across the tibia at an oblique angle. The anterior and posterior guide pins are placed at 15° of obliquity to the tibial shaft and verified by intraoperative fluoroscopy. An alternative technique is to place the site of the osteotomy directly above the posterior guide pin, maintaining the oblique angle of the pin across the osteotomy site without using the osteotomy guide system.
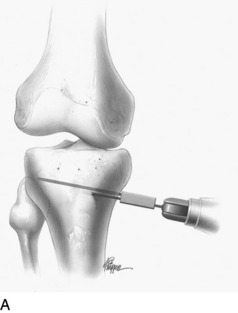
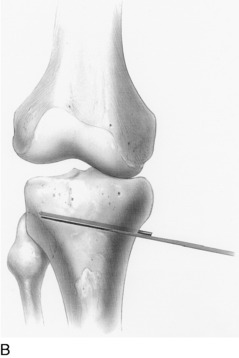
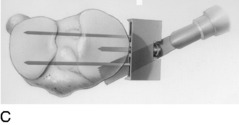
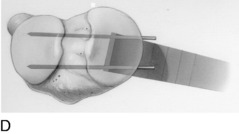
FIGURE 31-36 A–D, Correct placement for guide pin and subsequent osteotomy with a thin osteotome. The lateral cortex is preserved.
(A–D, Reprinted with permission from Noyes, F. R.; Barber-Westin, S. D.; Roberts, C. S.: High tibial osteotomy in knees with associated chronic ligament deficiencies. In Jackson RW [ed.]: Master Techniques in Orthopaedic Surgery, Reconstructive Knee Surgery. Philadelphia: Lippincott Williams & Wilkins, 2003; pp. 229–260.)
At this point, it is imperative to ensure that the medial osteotomy line (from anterior to posterior) is in line with the tibial slope based on the radiographs and prior anteromedial cortex joint line measurements by the Keith needles. A measurement of the perpendicular cut from the joint line confirms the distance of each guide pin from the articular surface of the tibia. The length of the posterior pin (medial to lateral cortex) is measured and used following the law of triangles105 to determine tibial width and the millimeters of osteotomy opening to obtain the desired angular correction (see Table 31-6).
Step 6: Opening Wedge Technique and Measurement of Gaps to Confirm Tibial Slope
Commercially available calibrated opening wedges are gently inserted into the osteotomy site to achieve the desired angular correction with the opening medial gap hinging on the intact posterolateral cortex (Fig. 31-37). This step requires several minutes to prevent fracture of the lateral tibial pillar. The spreader bars are inserted entirely across the osteotomy site to prevent a fracture extending into the lateral tibial plateau. If there is a rigid nonyielding response to the spreader bars, the lateral cortex is weakened with three to four guide pin perforations.
The anterior gap of the osteotomy site should be one half of the posterior gap, following rules previously described to maintain the tibial slope.105 The width of the tibial plate along the anteromedial cortex and just anterior to the SMCL is measured and is always less than the millimeters at the posterior medial gap owing to the angular inclination of the anteromedial tibial cortex.
Step 7: Intraoperative Verification of Desired Limb Alignment Correction
Obtaining an alignment correction in which the WBL is transferred to the lateral tibiofemoral compartment within the desired 50% to 62% range is the key to achieving short- and long-term decreases in pain in the medial tibiofemoral compartment. The surgeon cannot simply rely on the preoperative calculations because the intraoperative hip-knee-ankle fluoroscopic measurements may indicate that the desired correction of the tibial WBL has not been achieved. This problem was demonstrated in a report by Marti and coworkers,77 who described the accuracy of obtaining the desired alignment correction using preoperative and postoperative double-stance radiographs after opening wedge osteotomy in 32 knees. In their series, only 50% of the limbs demonstrated the desired correction on postoperative radiographs (±5° of calculated correction). An undercorrection was found in 31% and an overcorrection in 19%. The authors did not report on the use of intraoperative fluoroscopy to verify the lower limb correction. Many authors have described problems in obtaining the desired angular correction at surgery, with a substantial percentage of limbs remaining in varus or overcorrected into excessive valgus. The development of computerized navigation techniques to increase the accuracy of obtaining the desired tibial WBL and slope correction is, in the authors’ opinion, distinctly advantageous. Both fluoroscopy and computerized navigation techniques require defining accurate anatomic landmarks and precision to obtain accurate measurements.
Step 8: Placement of Bone Graft and Internal Fixation
The senior author recommends structural corticocancellous wedge bone grafts (autograft or allograft) and believes that synthetic grafts or cancellous grafts require further clinical evaluation before being accepted into practice. The clinical studies using these grafts have shown an unacceptable nonunion rate, as is discussed later in this chapter. The author prefers autograft iliac crest grafts or freeze-dried tibia or iliac crest corticocancellous allografts supplemented with a platelet gel.29
Management of the SMCL
The need to surgically reconstruct the SMCL is infrequent; however, the surgical techniques presented allow for restoration of function when required. At the conclusion of the SMCL reconstruction, plication of the posteromedial capsule anteriorly to the SMCL is performed to remove any abnormal capsular laxity with the knee at full extension. Overtightening of the SMCL and posteromedial capsules is avoided. The repair allows for full knee extension and a normal range of flexion. The technique is described in further detail in Chapter 24, Medial and Posteromedial Ligament Injuries: Diagnosis, Operative Techniques, and Clinical Outcomes.
OPERATIVE TECHNIQUE: CLOSING WEDGE TIBIAL OSTEOTOMY
Step 1: Initial Operating Room Preparation and Arthroscopy
Critical Points OPERATIVE TECHNIQUE CLOSING WEDGE OSTEOTOMY
Step 2: Initial Anterolateral Tibial Approach
A sterile tourniquet is inflated, and an oblique incision is made 1 cm distal to the head of the fibula in a line directed to the center of the tibial tubercle (Fig. 31-38). The subcutaneous tissues are incised down to the fascia of the anterolateral tibial musculature. A fascial incision is made from the lateral aspect of the tibial tubercle sloping up proximally to the distal aspect of Gerdy’s tubercle and is extended posteriorly and laterally to the anterior bare area of the fibula. The anterior bare area of the fibula is an important and safe anatomic landmark. The FCL and peroneal nerve are safely avoided when one identifies this area, which is used to initiate the limited subperiosteal exposure of the fibular neck for the fibular osteotomy.
Step 3: Fibular Osteotomy
The preferred procedure is a proximal fibular osteotomy through the fibular neck region (see Fig. 31-38). Meticulous surgical technique with protection and palpation of the peroneal nerve is essential. The peroneal nerve is always identified and partially dissected. In order to protect the fibers of the peroneal nerve, the lateral and posterior periosteal sleeve is carefully preserved and not retracted under tension. If there is any question as to the position of the nerve or chance of nerve damage, exposure of the entire nerve is indicated with direct visualization and protection.
The surgeon should be well aware of the anatomic variability of the superficial peroneal nerve (SPN) in the middle third of the leg. Barrett and colleagues14 reported on this factor in 35 nonpaired and 40 paired cadaver lower extremities. The SPN was identified in the lateral compartment adjacent to the fascial septum in 72%, in the anterior compartment in 23%, or with a branch in both the anterior and the lateral compartments in 5% of the specimens. Ducic and coworkers,34 in a study of 111 cadaver and clinical specimens, described a similar variability of the location of the SPN in the middle third of the leg. The SPN traveled to the lateral compartment in 70% of the specimens or split with branches in both the lateral and the anterior compartments in 16%. In 6% of the specimens, the SPN was found within the intermuscular septum, and in 8%, traveled only within the anterior compartment.
Step 4: Proximal Osteotomy: Removal of Tibial Wedge
After the preoperative measurements and based on tibial width (see Table 31-6), the entry point of the second guide pin distally is marked with proper positioning of the guide pins confirmed with fluoroscopy. These guide pins determine the osteotomy triangular bone wedge that is removed to achieve the desired correction. It is important to determine whether the lateral cortex is entirely perpendicular at the osteotomy site. In some knees, there will be a slight triangular anterior slope where it is necessary to downsize the osteotomy gap anteriorly in order not to decrease the tibial slope. The width of the tibia anteriorly is measured and the anterior gap determined (see Table 31-6).
It is important that the HTO does not increase or decrease the normal posterior tibial slope. The sagittal plane of the osteotomy should be perpendicular to the long axis of the tibia with the width of posterolateral cortex removed based on the coronal angular corrections required (see Table 31-6). In select cases, a biplanar osteotomy for correction of an abnormal tibial slope may be required, as already described. An opening or closing gap in the sagittal plane is performed to the necessary degrees of slope correction.
Step 5: Fluoroscopic Verification of the WBL for Corrected Alignment
Internal fixation of the osteotomy is achieved using an L-shaped plate. Two 6.5-mm cancellous screws are placed in the proximal tibia and three cortical screws are placed distal to the osteotomy (Fig. 31-39). Rarely, a 6.5-mm cancellous screw is placed in a lag fashion across the osteotomy site into the medial aspect of the proximal tibial bone for additional fixation. Newer locking plates and screws provide the advantage of more secure fixation. The final WBL is determined after fixation.
The lower limb is wrapped with cotton and additional padding is placed posteriorly and over the peroneal nerve, followed by an Ace bandage, postoperative hinged brace, and bilateral ankle-foot compression boots. A commercial ice delivery system with the bladder incorporated over the initial cotton wrapping, a few layers from the wound, is always used. The neurovascular status is checked immediately in the operating room and carefully monitored in the initial postoperative period. A calf or foot compression system is used for the first 24 hours to promote venous blood flow. Aspirin is prescribed and, rarely in high-risk patients, low-molecular-weight heparin (LMWH) or warfarin sodium (Coumadin; Bristol-Myers Squibb Co., Plainsboro, NJ). Early rehabilitation emphasizes early quadriceps isometrics, range of knee motion, ankle pumping, and ambulation (see Chapter 33, Rehabilitation after Tibial and Femoral Osteotomy). The surgeon should have a high suspicion for venous thrombosis and a low threshold for ordering ultrasound evaluation in the postoperative period, which is obtained in the majority of patients in the first few days after surgery.
With the meticulous dissection technique described, a postoperative compartment syndrome should be an exceedingly rare occurrence, if at all. The operative limb is always elevated for the 1st postoperative week, except for periods of ambulation with crutches. The maximum swelling of limb tissues after HTO is usually present 48 to 96 hours postoperatively. The postoperative rehabilitation program is discussed in Chapter 33, Rehabilitation after Tibial and Femoral Osteotomy.