Chapter 22 Posterolateral Ligament Injuries
Diagnosis, Operative Techniques, and Clinical Outcomes
INDICATIONS
The primary soft tissue stabilizing structures of the lateral and posterolateral (PL) aspect of the knee joint are the fibular collateral ligament (FCL) and popliteus muscle-tendon-ligament unit (PMTL), including the popliteofibular ligament (PFL) and posterolateral capsule (PLC) shown in Figure 22-1. These structures function together to resist lateral joint opening (LJO), posterior subluxation of the lateral tibial plateau with tibial rotation, knee hyperextension, and varus recurvatum.11,12,31,32,47,54
The mechanism of injury may be contact or noncontact and usually involves a combined varus and hyperextension joint displacement. The proper management of injuries involving the PL structures requires knowledge of the complex anatomy and potential variations that may exist, the function of the major soft tissue stabilizers, appropriate diagnostic techniques, and surgical options for reconstruction. Isolated PL injuries are rare; however, on occasion, an avulsion fracture at the femoral attachment occurs requiring internal fixation.24 PL injuries are frequently accompanied by anterior cruciate ligament (ACL) or posterior cruciate ligament (PCL) ruptures.1,8,23
Although the incidence of PL injury is unknown (owing to misdiagnosis or failure to detect the injury), the consequences of untreated PL ruptures are readily apparent. Chronic deficiency of the PL structures may be a factor in the failure of cruciate reconstructions34,36,42 and may also play a role in the development of gait abnormalities and giving-way.43,45,51 The detection and proper treatment of these problems is critical, because failure to properly treat all of the abnormalities may result in a poor outcome. The patient will complain of a varus type of instability with LJO during stance phase and show either a neutral or a valgus alignment. The abnormal LJO during stance phase is always greater than that detected on the varus stress test. The patient may demonstrate the abnormal LJO by producing a varus loading at the knee joint while standing.
Knees that fulfill the double or triple varus diagnosis criteria (varus osseous malalignment with increased LJO, external tibial rotation, varus recurvatum, and knee hyperextension [see Chapter 31, Primary, Double, and Triple Varus Knee Syndrome: Diagnosis, Osteotomy Techniques, and Clinical Outcomes])40 require high tibial osteotomy (HTO) first, followed approximately 6 months later with an appropriate PL reconstruction. In many instances, an ACL or PCL deficiency also exists, which is corrected at the time of the PL reconstruction.
CONTRAINDICATIONS
Contraindications to PL reconstruction are findings of less than 5 mm of increased lateral tibiofemoral joint opening and less than 10° of increased external tibial rotation. These findings are frequently noted in knees with associated varus osseous malalignment (double varus knees) that are candidates for HTO (see Chapter 31, Primary, Double, and Triple Varus Knee Syndromes: Diagnosis, Osteotomy Techniques, and Clinical Outcomes).33 Correction of the varus malalignment promotes physiologic remodeling and shortening of the PL structures, decreasing the abnormal LJO and external tibial rotation and thus negating the need for a PL operative procedure.
Patients with varus malalignment who do not undergo HTO and have associated chronic insufficiency of the PL structures are not candidates for a PL procedure. Untreated varus osseous malalignment is a cause of failure of PL reconstructions.39 In many cases, a knee hyperextension gait abnormality also exists that must be corrected before surgery with a specific gait-retraining program described in Chapter 34, Correction of Hyperextension Gait Abnormalities: Preoperative and Postoperative Techniques.43 Failure to correct a hyperextension gait abnormality places PL reconstructions at risk for failure owing to the excessively high tensile forces placed on the PL soft tissues upon weight-bearing after surgery. Gait retraining usually decreases abnormally high knee extension and adduction moments to normal values.43
CLINICAL EVALUATION
Critical Points CLINICAL EVALUATION
History
Tibiofemoral Rotation Dial Test
One frequent patient presentation is a failed ACL or PCL reconstruction owing to untreated PL insufficiency. Another patient presentation is a chronic varus osseous malalignment and underlying ACL insufficiency in which, over time, interstitial stretching and slackening of the PL structures occurred.33,40 In these cases, HTO unloads the PL soft tissues to the extent at which physiologic remodeling and shortening occur and PL reconstruction is not required.40
A comprehensive physical examination is required, including assessment of knee flexion and extension, patellofemoral indices, tibiofemoral crepitus, tibiofemoral joint line pain, and gait abnormalities. Pain in the medial tibiofemoral compartment occurs owing to increased compressive forces related to varus osseous malalignment. Pain in the PL soft tissues may occur from increased soft tissue tensile forces due to a varus thrusting gait pattern. The abnormal knee hyperextension involves increased extension in the sagittal plane and is often accompanied by a varus alignment in the coronal plane, which has been described as a varus recurvatum alignment. Together with a varus osseous malalignment, this is referred to as a triple varus knee (see Chapter 31, Primary, Double, and Triple Varus Knee Syndromes: Diagnosis, Osteotomy Techniques, and Clinical Outcomes). Patients with chronic PL insufficiency have varying amounts of altered gait mechanics and knee hyperextension. Some individuals may present with a markedly abnormal gait that is severely disabling and limits ambulation. Other patients may have a less noticeable alteration because the abnormal knee hyperextension occurs only after prolonged walking and muscle fatigue. The abnormal gait pattern is characterized by excessive knee hyperextension during the stance phase, which does respond to gait retraining that initiates normal stance phase flexion (see Chapter 34, Correction of Hyperextension Gait Abnormalities: Preoperative and Postoperative Techniques). Subjective complaints of giving-way during routine daily activities, along with severe quadriceps atrophy, often accompany this gait abnormality.
The surgeon must determine all of the abnormal translations and rotations in the knee joint. The ligament injuries that result in knee hyperextension and varus recurvatum frequently involve not only the PL structures but also other ligament and capsular structures. The biomechanical and kinematic studies that form the basis for the interpretation and diagnosis of the manual stress tests are described in Chapter 20, Function of the Posterior Cruciate Ligament and Posterolateral Ligament Structures.
The increases in LJO and external tibial rotation shown in Table 22-1 are only approximations of what would be expected with clinical injury to the PL structures. Importantly, an increase of only a few millimeters (2–5 mm) in LJO occurs with complete rupture of the FCL, whereas an increase of 5 to 9 mm occurs with complete rupture of all the PL structures (FCL, PMTL, PFL). These values are based on biomechanical studies discussed in Chapter 20, Function of the Posterior Cruciate Ligament and Posterolateral Ligament Structures, under moderate varus loads (20 Nm). LaPrade and colleagues20 conducted a cadaveric study in which lateral stress radiography was applied at 12 Nm (on an experimental apparatus) and the increase in LJO over the intact state was compared with that measured during a clinician-applied load after an isolated FCL rupture and a combined FCL, PMTL, PFL rupture. Compared with the intact state, LJO induced by the clinician-applied load increased by 2.7 mm (isolated FCL rupture) and 4.0 mm (combined PL rupture). However, the mean values showed a wide standard deviation and variation between specimens, making extrapolation to the clinical setting difficult. In addition, the lateral joint space measurement showed wide confidence intervals. For an isolated FCL rupture, the mean lateral gap distance was 10.99 mm (confidence interval [CI], 7.8–14.3 mm) and for the combined PL rupture, the mean distance was 12.2 mm (CI, 9.3–15.2 mm). This amount of overlap indicates that it would not be possible to accurately separate an FCL rupture alone from a combined PL injury. The measurements are important and useful in providing the clinician with a baseline in interpreting lateral stress radiographs. The gap test measurement at arthroscopy described extensively in this book is based also on these types of approximations. The gap test is based on the joint separation between articular cartilage seen at arthroscopy, and not the cortical separation on a stress radiograph. Even so, the measurements are somewhat equivalent as to the increase in the amount of millimeters with PL injuries. For example, Figure 22-2 shows an approximately normal lateral gap of 4 mm at the closest point of the lateral compartment at arthroscopy. An increase of only 6 mm results in 10 mm of absolute opening at the closest point, or 12 mm at the periphery, which is viewed as a positive gap test and indicative of injury to the PL structures. Fortunately, in most knees, these are the lesser values and it is more common that the lateral gap exceeds these measurements, indicating that concurrent PL reconstruction is necessary.
An increase in external tibial rotation may occur with anterior subluxation of the medial tibial plateau, posterior subluxation of the lateral tibial plateau, or a combination of both subluxations. The dial or spin rotation test, which the senior author developed, allows a diagnosis of tibial rotatory subluxations of the medial and lateral tibiofemoral compartments at 30° and 90° of knee flexion (see Table 22-1). Other variations of this test have been described.6,44,55
The position of the medial and lateral tibial plateau is assessed at the starting position (neutral tibial rotation) with the knee flexed to 30° and 90° and at the final position with the tibia in maximal external rotation. The examiner palpates the position of the medial and lateral tibial plateau, which is compared with the normal knee to assess whether a subluxation (anterior or posterior) of the medial or lateral tibial plateau is present. An increase in internal tibial rotation occurs with medial ligament and PCL disruption (see Chapter 20, Function of the Posterior Cruciate Ligament and Posterolateral Ligament Structures). The axis of tibial rotation is observed in the involved knee and compared with the normal knee to detect a shift in the medial or lateral tibiofemoral compartment during tibial rotation. It is not recommended that this test be performed in the prone position because the tibiofemoral joint cannot be accurately palpated to distinguish an anteromedial from a PL tibial subluxation.
The use of the dial test in knees with PCL ruptures requires maintenance of a normal anatomic tibiofemoral position. This is accomplished by applying a gentle anterior translation, loading the ACL in both limbs, during the external tibial rotation. It is still necessary to use the supine position so that the examiner can palpate the tibiofemoral position.52 The dial test is less accurate with a PCL rupture, because it is difficult to compare limbs, and other tests to be described (LJO, gap test at arthroscopy, varus recurvatum) for the integrity of the PL structures need to be carefully assessed.
Posterior stress radiographs are obtained in patients with PCL ruptures, especially those in which the distinction of a partial versus a complete PCL deficiency is difficult to determine on clinical examination.14 A lateral PCL stress radiograph is taken of each knee at 90° of flexion. The limb is placed in neutral rotation with the tibia unconstrained and the quadriceps relaxed, and 89 N force applied to the proximal tibia. Measurement is made of the millimeters of posterior tibial translation in both knees. Knees with 10 mm or more of increased posterior tibial translation are considered candidates for PCL reconstruction.
Full standing radiographs of both lower extremities, from the femoral heads to the ankle joints, are done in knees with varus lower extremity alignment. The mechanical axis and weight-bearing line are measured to determine whether HTO is indicated.10
Patients complete questionnaires and are interviewed for the assessment of symptoms, functional limitations, sports and occupational activity levels, and their perception of the overall knee condition according to the Cincinnati Knee Rating System (CKRS; see Chapter 44, The Cincinnati Knee Rating System).2
CLASSIFICATION AND TREATMENT OF PARTIAL TO COMPLETE PL INJURIES
The classification and treatment of first-, second-, and third-degree acute PL injuries is detailed in Table 22-2. It is important to diagnose partial tears of the PL structures, with a mild to moderate increase in LJO and external tibial rotation, to allow protection and maintain lateral tibiofemoral joint closure in the initial 3 weeks to allow “stick-down” and healing of lateral soft tissues. This program is similar to that recommended for medial ligament ruptures (see Chapter 24, Medial and Posteromedial Ligament Injuries: Diagnosis, Operative Techniques, and Clinical Outcomes).
PREOPERATIVE PLANNING: TIMING OF SURGERY
Acute Injuries
There is a distinct advantage for repairing completely disrupted PL structures and meniscal attachments in acute injuries (Fig. 22-3). At the time of surgery, extensive disruption of these structures is observed. Careful dissection is required to identify anatomic tissue planes and maintain an intact vascular and neural supply. The so-called golden period to perform an acute surgical repair is within 7 to 14 days of the injury. After this time, scar tissue will obliterate tissue planes and make the dissection and repair difficult.
Chronic Injuries
Patients with chronic knee injuries that present with severe muscle atrophy require several months of preoperative rehabilitation. Patients with a hyperextension gait abnormality must complete a gait-retraining program,43 described in detail in Chapter 34, Correction of Hyperextension Gait Abnormalities: Preoperative and Postoperative Techniques. This program is done in addition to lower extremity muscle strengthening exercises. In the authors’ experience, patients will convert to a more normal gait pattern after 4 to 6 weeks of training. More time is required for severe quadriceps atrophy before surgical intervention.
Varus osseous malalignment must be corrected before chronic PL reconstruction, as described previously. Failure to address this malalignment will greatly increase the risk of failure of any PL procedure (Fig. 22-4). In some cases in which the PL deficiency is due to interstitial stretching of the tissues and not a traumatic rupture, a simplified proximal advancement of the PL structures may be performed with the HTO. In anatomic PL reconstructions, the ligament surgery is staged after healing of the HTO. The indications for the various PL procedures are described in detail under the “Operative Treatment of Acute PL Ruptures” and “Operative Treatment of Chronic PL Ruptures” sections.
Patients who have undergone prior lateral meniscectomy and who demonstrate early tibiofemoral arthritis are considered for lateral meniscus transplantation.41
Cruciate Graft Reconstruction
The majority of patients who undergo PL reconstruction require a concomitant ACL or PCL reconstruction (Fig. 22-5). The appropriate grafts for the cruciate procedures should be determined; autogenous tissues with bony fixation are preferred. However, the surgeon should ensure that B-PT-B and Achilles tendon–bone (AT-B) allografts are available the day of surgery. These will be required if autogenous tissue is unavailable or not suitable for the PL or cruciate procedures.
INTRAOPERATIVE EVALUATION
All knee ligament tests are performed after the induction of anesthesia in both the injured and the contralateral limbs. The amount of increased anterior tibial translation, posterior tibial translation, LJO, and external tibial rotation is documented. A thorough arthroscopic examination is conducted, documenting articular cartilage surface abnormalities (see Chapter 47, Articular Cartilage Rating Systems) and the condition of the menisci.46
The gap test is done during the arthroscopic examination.40 The knee is flexed to 30° and a varus load applied. A calibrated nerve hook is used to measure the amount of lateral tibiofemoral compartment opening (see Fig. 22-2). Knees that have 12 mm or more of joint opening at the periphery of the lateral tibiofemoral compartment require a PL reconstructive procedure.
OPERATIVE TREATMENT OF ACUTE PL RUPTURES
Operative Setup and Patient Positioning
Identification of Ligament and Soft Tissue Rupture Pattern
A 10- to 12-cm skin incision is made in a straight line centered over the joint line and 1 cm posterior to the iliotibial band (ITB) attachment at the tibia (Fig. 22-6A). After careful mobilization of the skin flaps, the ITB, biceps tendon, and lateral structures are encountered.
In the majority of knees, the ITB will be intact or demonstrate only partial tearing. In select cases, the ITB will be completely disrupted at the joint line or avulsed off its tibial attachment at Gerdy’s tubercle. Posteriorly, there are capsular attachments of the ITB, including fascial attachments to the short head of the biceps femoris, which are identified for later repair if torn. If the ITB is intact, an incision is made along its posterior border to allow visualization of all of the underlying structures (see Fig. 22-6B).
The lateral capsular tissues and meniscal attachments are the next structures visualized. A vertical incision is made into the anterior third of the capsule and extended to the lateral meniscus just anterior to the popliteus tendon attachment. The popliteus tendon and meniscus attachments at the femoral popliteal recess are identified. Frequently, it is necessary to repair the superior and inferior meniscal fasciculi (Fig. 22-7) to restore meniscal attachments to the lateral meniscus. Careful varus stress is placed on the knee joint to allow inspection of the lateral meniscus attachments and tibiofemoral articular cartilage. On occasion, an additional anterior incision is required for visualization of underlying anatomy (see Fig. 22-6C).
The fibular head and attachments of the biceps femoris short and long head are the next structures visualized, which have been described in detail in Chapter 2, Lateral, Posterior, and Cruciate Knee Anatomy. The two tendinous components (direct and anterior arms) and one of the fascial components (lateral aponeurotic expansion) make up the key portion of the long head anatomy. The other fascial components are the reflected arm and the anterior aponeurotic expansion.
The most proximal component is the reflected arm. It originates just proximal to the fibular head and ascends anteriorly to insert on the posterior edge of the ITB. The direct arm inserts onto the PL edge of the fibula just distal to the tip of the styloid. A portion of the anterior arm inserts onto the lateral aspect of the fibular head, and the rest continues distally just lateral to the FCL. Portions of the anterior arm ascend anteriorly forming the lateral aponeurotic expansion that attach to the posterior and lateral aspects of the FCL. Here, a small bursa separates the anterior arm from the distal fourth of the FCL. The anterior arm thus forms the lateral wall of this bursa (see Fig. 2-12). This is an important surgical landmark, because a small horizontal incision can be made here, 1 cm proximal to the fibular head, to enter this bursa and locate the insertion of the FCL into the fibular head. The anterior arm then continues distally over the FCL, forming the anterior aponeurosis, which covers the anterior compartment of the leg. The primary areas of injury are tendon avulsions off of the fibula, which often have a major osseous component that can be repaired. In addition, the fascial extensions anteriorly and laterally are repaired.
The short head of the biceps courses just deep (or medial) and anterior to the long head tendon, sending a majority of its proximal muscular fibers to the long head tendon itself.53 It has six distal attachments, described in detail in Chapter 2, Lateral, Posterior, and Cruciate Knee Anatomy. The most important attachments are that of the direct arm, the anterior arm, and the capsular arm.
The interval between the posterior capsule and the gastrocnemius tendon is entered just above the fibula, similar to the exposure for a lateral meniscus repair. This exposes the popliteus muscle tibial attachments, popliteus muscle-tendon junction, PFL, popliteus tendon attachment at the femur, and fabellofibular ligament (extension of short head biceps attachments) (see Fig. 22-6D).
In dissection studies, LaPrade and coworkers19 described a fabellum (osseous or cartilagenous) present in all specimens. This structure forms an attachment for the oblique popliteal ligament and fabellofibular ligament, which along with the posterior capsule, are important restraints for limiting knee hyperextension. Although individual capsular components and structures are difficult to discern with extensive capsular ruptures, it is important to repair disrupted posterior capsular tissues after completion of the initial dissection.
Peroneal Nerve Identification
It is important at the initial stages of the dissection to palpate and determine the location of the CPN. To expose the CPN, it is safest to begin in the proximal aspect of the operative exposure. A large retractor is used to elevate the muscular portion of the biceps femoris, placing the fascial tissues beneath the biceps muscle under gentle tension. This gentle upward displacement of the biceps muscle is key to visualize and dissect the CPN, because its normal curviform undulations are removed and it assumes a straighter appearance (Fig. 22-8A). The investing crural fascia is incised over the CPN to the fibula.
The CPN and its branches are not removed from their normal anatomic position to avoid damaging the delicate blood supply, particularly in the region where the CPN approaches and then passes around the fibular neck. Kadiyala and associates16 reported measurements in cadaveric specimens of the blood supply to the CPN in the popliteal fossa and fibular neck region. These authors hypothesized that the susceptibility of the CPN to injury or lack of a response to operative treatment when injured may be related to deficiencies in intraneural and extraneural vascular supply and anastomoses.
The most common source of blood supply to the proximal portion of the CPN is a direct branch of the popliteal artery. This branch divides into proximal and distal anastomotic vessels that run in the connective tissue sheath of the nerve and anastomoses with the anterior recurrent tibial artery. The vessels, located in the epineurium, give rise to many small vessels of fine caliber, which extend 20 to 30 mm within the substance of CPN. It is important not to disturb this blood supply. Kadiyala and associates16 noted that the blood supply of the CPN was somewhat sparse with poor vascularization. A connection of the vasa nervorum was not found from the geniculate arteries, but occasional contributions from muscular branches were recognized (Fig. 22-9).
Bottomley and colleagues3 reviewed the anatomic position of the CPN in 54 patients who had damage to the PL structures. The CPN was noted to be displaced out of its normal position in 16 of 18 patients who had biceps avulsions or associated fibular head fractures. These authors advised that the surgeon should expect an abnormal nerve position on surgical exploration in knees with bone or soft tissue avulsion from the fibular head and the potential for iatrogenic damage.
Rubel and coworkers49 conducted an anatomic investigation of the CPN in 31 cadaveric limbs by dissecting the CPN to its intramuscular branches. The authors described Gerdy’s safe zone as the area where the CPN and anterior recurrent branch defined an arc with an average radius of 45 mm. The distance between the fibular head and Gerdy’s tubercle was used to determine the radius of the safe zone. Therefore, this region in the proximal aspect of the tibia is advantageous for surgical exploration, because damage to the peroneal nerve and its branches is avoided (Fig. 22-10). The CPN divides into three branches as it enters the anterolateral musculature, with the anterior recurrent branch more proximal to the superficial and deep peroneal branches.
Dellon and associates9 reported on the anatomic variations of the CPN at the fibular head in 29 cadavers (bilaterally) and 65 patients treated with a CPN decompression for symptoms. Three possible anatomic variants were described that require attention and decompression in chronic neuropathies for a successful outcome. First, the superficial fascia of the superficial head of the peroneus longus muscle is divided by a proximal and distal transection of the fascia (found in 30% of cadavers and 78% of patients; see Fig. 22-8B). Second, when the peroneus longus muscle at the fibular neck is partially incised adjacent and superior to the CPN and the peroneus muscle lifted anteriorly, a fibrous band may be found that requires release (soft tissue restriction found in 43% of cadavers and 20% of patients; see Fig. 22-8C). Third, there may be a fibrous connection between the peroneus longus and the soleus muscle requiring division (found in 9% of cadavers and 6% of patients). These authors advise that after CPN decompression, the surgeon’s index finger should be able to gently pass along the CPN and into the anterolateral compartment (see Fig. 22-8D).
Surgical Repair and Reconstruction of Acutely Disrupted Ligaments and Soft Tissues
The key to restore function to the disrupted PL structures, muscle attachments, and lateral meniscus attachments is a meticulous dissection, identification of damaged tissues, and repair of all injured structures. There does exist an unacceptably high risk of failure of primary repairs of disrupted PL structures, particularly the FCL, owing to high lateral tensile forces exerted on these tissues postoperatively.50 Therefore, it is necessary to reconstruct one or more disrupted PL structures with an autograft or allograft, as is described. This adds tissue integrity and sufficient repair strength to resist LJO and external tibial rotation in the initial healing period of 4 to 6 postoperative weeks.
Surgical Approach and Order of Surgical Repair
The surgical approach favored is a graft reconstruction of the FCL with either a B-PT-B autograft or allograft or an AT-B allograft. The authors were the first to describe a femoral-fibular reconstruction,37 which is a second option and is described later in this chapter. The FCL fibers (unless directly avulsed at their insertion) are too disrupted to perform a primary repair. The FCL reconstruction provides for secure fixation, prevents abnormal joint displacements in the immediate postoperative period, and allows for early protected knee motion. These procedures are not difficult because the attachment sites on the femur and fibula are easily identifiable. Importantly, the graft provides the cornerstone about which the remainder of the soft tissue repair of the PL structures is performed. A B-PT-B graft requires an appropriate length graft, which may not always be obtained. Alternative graft options are AT-B or quadriceps tendon–patellar bone (QT-PB). The bone portion of these grafts may be placed at either the FCL fibular attachment or the FCL femoral attachment, and the tendon may be placed within a tunnel at the other attachment site. If a soft tissue tendon graft is selected, the graft is passed through a fibular tunnel (anterior-to-posterior), the tendon is sutured back upon itself, and a soft tissue interference fibular screw may be added.
After all of the anatomic structures and rupture sites are identified and carefully exposed, the order of the operative repair starts with deeper structures and proceeds to superficial structures. Examples of an acute operative repair are shown in Figures 22-11 and 22-12.
OPERATIVE TREATMENT OF CHRONIC PL RUPTURES
Overview of Operative Options
Markolf and colleagues28–30 reported a series of cadaveric studies on the effect of a nonanatomic PL ligament reconstruction in restoring stability to a PCL-reconstructed knee. The PL reconstructions involved an FCL graft and either a popliteus tendon femoral-tibial reconstruction or a popliteus femoral-fibular PFL graft. The isometric attachment points for the graft were determined at surgery by taking the knee through flexion-extension and measuring the length changes at the attachment sites. The authors selected a femoral popliteus tendon graft placement that was 11 mm anterior and 2.7 mm proximal to the native popliteus femoral footprint. This nonanatomic placement, based on their isometric techniques, raises questions as to the application of the data to clinical surgical techniques. Each graft was tensioned in neutral tibial rotation, with 30 N of load placed on each of the grafts. This loading resulted in a markedly overconstrained internal tibial rotation and varus knee position throughout knee flexion. The authors concluded that additional studies were necessary because there is no consensus on the graft tensioning routine for PL reconstructions.
Anatomic Reconstruction of the FCL and PMTL
Patient Positioning and Surgical Approach
An operative time-out and identification of the operative limb are performed as already described. The patient is positioned on the operative table, with a high thigh tourniquet placed as previously described in the “Acute Injury” section. A leg holder is used only if a meniscus repair is anticipated to provide for limb control and opening of the medial tibiofemoral compartment. Otherwise, the lower limb is draped free with a bolster placed under the proximal thigh to allow the popliteal neurovascular structures to drop posteriorly away from the dissection plane. The initial arthroscopic evaluation is performed including meniscus repairs and drilling of tunnels for concurrent cruciate reconstructions for placement of cruciate grafts as described in Chapters 7, Anterior Cruciate Ligament Primary and Revision Reconstruction: Diagnosis, Operative Techniques, and Clinical Outcomes, and 21, Posterior Cruciate Ligament: Diagnosis, Operative Techniques, and Clinical Outcomes. There are two options with cruciate reconstructions. The grafts may be placed with the distal fixation performed after the lateral dissection. If this approached is selected, the order of final graft tensioning and fixation is (1) PCL, (2) ACL, (3) FCL, and (4) PMTL. The rationale is to restore the tibiofemoral joint in the sagittal plane and then perform the final PL graft fixation and repair of disrupted tissues. The second option is to complete the cruciate reconstruction and then repair and reconstruct the PL structures. If this sequence is followed, the surgeon must carefully control the limb during the dissection and repair steps to make sure there is not inadvertent opening of the lateral tibiofemoral joint that would disrupt the cruciate graft fixation. In general, the first option is safest.
The surgeon is seated with a headlight to allow a meticulous dissection of the PL structures and CPN. The tourniquet is inflated during the initial dissection and then deflated during the remainder of the surgical procedure. A skin incision 10 to 12 cm in length is made in a straight line, centered over the joint line and 1 cm posterior to the ITB attachment at the tibia, using the same approach as already described (see Fig. 22-6).
Peroneal Nerve Dissection and Visualization
It may be beneficial to place a curved Kelly clamp in the anterolateral capsular incision, with the instrument passed beneath the popliteus tendon and FCL, to place tension into these tissues to facilitate further identification and inspection. The femoral anatomic attachments of all the PL structures are shown in Figure 2-1C and D and the surgeon should be thoroughly familiar with this anatomy because the goal of the surgical procedure is to restore normal anatomic attachment sites. Note the femoral FCL attachment is just superior to the lateral epicondyle and also that the insertion of the lateral gastrocnemius tendon is on the lateral aspect of the femoral condyle.
LaPrade and coworkers22 reported a mean distance of 18.5 mm from the FCL insertion to the popliteus tendon insertion, indicating that two separate grafts are required to anatomically reconstruct the FCL and popliteal tendon femoral attachments. Although some authors recommend a single graft placed at the femoral attachment and split into two strands to reconstruct both the FCL and the PMTL, this procedure does not reproduce the anatomic femoral attachment sites. For these reasons, a separate graft and femoral attachment for the FCL and PMTL are recommended.
FCL B-PT-B Reconstruction
Graft Choices for FCL Reconstruction
The normal anatomic attachment sites of the FCL to the lateral femur and anterolateral aspect of the fibular head are carefully identified.22 A suture is placed between the two attachment sites and the length is measured to determine the required graft size. The bone portion of each end of the graft is 22 to 25 mm in length. The fibular graft attachment is performed using a tunnel at the anatomic attachment site. The femoral graft attachment is performed by placing a femoral tunnel at the anatomic attachment site. A second option is a femoral inlay of the proximal bone portion of the graft, which is useful if there is a 5- to 8-mm discrepancy of graft length that will not allow full coverage of the bone in a femoral tunnel.
Critical Points FIBULAR COLLATERAL LIGAMENT BONE–PATELLAR TENDON–BONE RECONSTRUCTION

B-PT-B, bone–patellar tendon–bone; CPN, common peroneal nerve; FCL, fibular collateral ligament.
The patellar tendon graft must normally be 50 mm or longer to be suitable for an anatomic FCL reconstruction. The average cross-sectional area of the FCL reported by LaPrade and associates18 is 11.9 ± 2.9 mm2. The FCL graft is 8 to 10 mm × 4 mm, resulting in a 32- to 40-mm2 graft. If the patient’s own tissue is of sufficient length, an autograft harvested from the ipsilateral or contralateral patellar tendon provides the most ideal graft. In revision cases in which a prior FCL allograft reconstruction has failed, an autograft approach is favored. The hypothesis is that an autograft will heal at the fibular and femoral tunnels, with minimal remodeling and weakening of the graft in the postoperative phase. The complications of a meticulously performed B-PT-B graft harvest from the contralateral knee are less than 1% in terms of infection, scar formation, and graft site pain.36
If an allograft is chosen, as is the usual case in multiligament reconstructions, a B-PT-B allograft is favored over a soft tissue graft owing to more prompt osseous incorporation and healing at femoral and fibular attachment sites. It is recognized that soft tissue allografts require added maturation time and may incompletely remodel even under the best of circumstances.27,48 Alternative options include an AT-B or QT-PB graft. The bone portion may be placed at either the fibular or the femoral site.
Placement of Fibular and Femoral Tunnels
The attachment sites at the fibula and femur are identified. The anterior “bare area” of the fibula is exposed for 20 mm, avoiding lateral dissection that may injure the CPN. The fibular tunnel is drilled first, using a guide pin to a depth of 25 mm. The drills are gradually increased in diameter to create a final 9-mm tunnel (Fig. 22-13). Care should be taken to avoid drilling too deep, because the drill would break out the cortex distally at the fibular neck, close to the location of the CPN. The normal cortical integrity of the fibular head is not disrupted to maintain circumferential cortical fixation strength at the fibular attachment site.
B-PT-B Graft Placement
The bone portion of the graft is gently taped into the fibular tunnel so that the bone is entirely seated into the tunnel and level with the proximal fibular head to preserve graft length. The bone portion of the graft is marked with ink to define the correct depth in the fibular tunnel. The ideal graft fixation is with two small-fragment cortical screws placed from anterior-to-posterior engaging both fibular cortices, in the proximal third and distal third of the bone portion of the graft (Fig. 22-14). The angle of the screw is posterior and never lateral in order to protect the CPN. The cortical screws may be 2.7 or 3.5 mm, based on the size of the graft and fibular head. A washer is used anteriorly. Alternative graft fixation methods include an interference screw and, rarely, sutures tied over the fibular cortex.
Critical Points POPLITEUS MUSCLE-TENDON-LIGAMENT REPAIR PROCEDURE
Graft Replacement of PMTL
The graft replacement of the PMTL is shown in Figure 22-15. An AT-B allograft is favored, with the bone portion of the graft placed at the anatomic femoral insertion site and the collagenous portion of the graft is passed in the tibial tunnel. Alternative grafts to consider are a B-PT-B allograft (which is more difficult to pass through the tibial tunnel) or a semitendinosus-gracilis (STG) two-strand autograft (which is less ideal because there is no bone attachment on the femur).
A final assessment of the graft is done to determine that it is under adequate tension and is blocking abnormal external tibial rotation and knee hyperextension. With graft reconstructions of both the FCL and the PMT, it is not necessary to add additional drill holes to the fibula to perform a graft reconstruction of the PMTL. Rather, a direct suture of the PMT graft to the FCL graft at the level of the fibular head is performed (see Fig. 22-15F and G). A plication procedure is performed of the PLC at 10° of flexion, avoiding overtension, which would limit normal extension (see Fig. 22-15H and I).
PLC Graft Reconstruction for Severe Varus Recurvatum and Hyperextension
In patients who demonstrate 15° or more of knee hyperextension, severe deficiency exists of the entire posterior capsule and oblique popliteal ligament in addition to possible cruciate, FCL, and PMTL damage (Fig. 22-16). In these severe knee injuries, a PMTL reconstruction alone will not block a severe varus recurvatum deformity. A PLC reconstruction is required in addition to a reconstruction of the PMTL and FCL (Fig. 22-17). The operative approach and placement of the tibial tunnel for the capsular reconstruction using an AT-B allograft is the same as described for the PMT reconstruction. The only difference is that the bone portion of the graft is placed adjacent to the lateral gastrocnemius tendon (LGT) origin by a femoral tunnel or a bone inlay. The inlay technique is required when a concurrent ACL reconstruction is performed to avoid a second femoral tunnel. The fixation at the femoral attachment requires a portion of the LGT insertion (which is very broad) to be partially incised to expose the site for the AT-B graft fixation. The fixation of the bone inlay is by two small-fragment cancellous screws and washers (Fig. 22-18). This provides a stable bone-to-bone attachment. If a femoral tunnel is used, a 7-mm interference screw is selected. The graft lies along the PLC, which is plicated (vest-over-pants), and the graft is passed through the tibial tunnel.
Critical Points POPLITEUS MUSCLE-TENDON-LIGAMENT GRAFT REPLACEMENT
FCL, fibular collateral ligament; PMTL, popliteus muscle-tendon-ligament.
Critical Points POSTEROLATERAL CAPSULE GRAFT RECONSTRUCTION
Femoral-Fibular Reconstruction
Critical Points FEMORAL-FIBULAR RECONSTRUCTION
A femoral-fibular reconstruction has disadvantages. In cadaver studies, a femoral-fibular graft was found not to unload a concurrent PCL graft reconstruction in the same manner as a combined FCL and PMTL graft reconstruction.29 In addition, although a single femoral-fibular graft stabilizes the knee at time zero, this graft may stretch out in the long term when there is loss of the PMTL, which does not participate in load sharing. Therefore, all the load is transferred to the single graft. The goal of PL reconstruction is to restore the function of all the PL structures and not just the femoral-fibular component. A modification of a femoral-fibular technique is to cross the graft, placing the anterior femoral portion to the posterior fibula to restore PFL function. However, whether this option is superior to two parallel femoral-fibular graft arms is not known.
A tendon allograft or autograft, 6 to 8 mm in diameter, is prepared. The graft is measured to allow sufficient length (19–20 cm) for the anterior and posterior arms of the circle graft to overlap posteriorly, which provides additional collagenous tissue to the PL aspect of the joint (Fig. 22-19A and B). Two interlocking closed loop (baseball) sutures of No. 2 nonabsorbable suture are placed into both ends of the graft. The graft is initially stretched for 15 minutes under an 89-N load with a ligament-tensioning device. A four-strand STG autograft or comparable allograft may be used.
An incision is made vertically just behind the FCL into the PLC. The tissues of the PLC, PMTL, and FCL are carefully inspected. The popliteus tendon is inspected from its insertion site to the muscle belly. If the PLC has excessive redundancy, then a simple capsular plication can be performed. The graft is inserted through bone tunnels in the femur and fibula, with the graft strands next to the stretched and slack FCL. The graft is placed under slight tension with the knee at 30° of flexion, neutral tibial rotation, and with the lateral side of the joint closed (after repair of meniscal attachments when necessary). It is important not to internally rotate the tibia during graft tensioning, which would result in an abnormal restraint of external rotation. Multiple interrupted sutures are used through the posterior overlapped arms of the graft (see Fig. 22-19C and D). The slack FCL is thus interposed between the anterior and the posterior arms of the circled graft, and horizontal sutures are placed between both structures (see Fig. 22-19E and F). Sutures are placed between the popliteus tendon at the musculotendinous junction to restore the attachment to the fibula (PFL). If the PMT is lax, the tendon may be shortened by direct repair or the tendon is advanced and recessed at its anatomic femoral attachment into a tunnel and fixated with an absorbable interference screw.
Proximal Advancement of the PL Structures
Critical Points PROXIMAL ADVANCEMENT OF POSTEROLATERAL STRUCTURES
The new attachment site for the PL structures is prepared by elevating the periosteum proximal to the FCL over a distance of 15 mm. A curved osteotome is used to elevate an 8-mm-thick × 15-mm-wide wedge of bone at the attachment site of the PL structures, extending distally to the joint, avoiding the articular cartilage (Fig. 22-20A and B). Two Allis clamps are placed on the osteotomized bone. Any remaining soft tissue attachments are cut with a knife. The PLC attachment is incised posteriorly, avoiding the popliteus tendon (see Fig. 22-20C and D).
The goal is to advance the FCL in a proximal direction to remove excessive slackness and to use staple fixation at the normal anatomic site. The bone attachment site may be slightly rotated to adjust the tension in the posterior capsular tissues to avoid overconstraining knee extension. The advanced bone attachment is fixed with a medium four-prong staple (see Fig. 22-20E and F). The distal margin of the staple is placed directly at the anatomic site of attachment of the FCL to restore normal FCL length. A cancellous bone screw is used for additional fixation.
The function of the FCL is determined after fixation to ensure that there is only 2 to 3 mm of LJO on varus stress testing and no abnormal external tibial rotation or hyperextension. The PL tissues are tensioned to allow the knee to come to 5° of flexion and to resist further extension after this point, gradually reaching 0° to –2° hyperextension during the postoperative rehabilitation period. The ITB tension is determined. A distal advancement of the ITB at the tibial attachment may be required to restore normal tension in this structure. The ITB is closed anteriorly and posteriorly with absorbable sutures. In order to prevent lateral tethering on the patella, the ITB and lateral patellar retinaculum are loosely closed or not closed. The proximal advancement procedure is often combined with other operative procedures when the more complicated anatomic PL reconstruction is not required (Fig. 22-21).
AUTHORS’ CLINICAL STUDIES
Anatomic PL Reconstruction
A consecutive group of knees that had an anatomic PL reconstruction including an FCL B-PT-B reconstruction were prospectively followed 2 to 13.7 years postoperatively.35 All major PL structures were surgically restored as required. The procedure represented a primary reconstruction in 7 patients and a revision in 6 patients. ACL ruptures were found in 7 patients and bicruciate ruptures in 5 patients, all of which were reconstructed.
At follow-up, 13 of the 14 (93%) PL reconstructions restored normal or nearly normal LJO and external tibial rotation (Fig. 22-22). The ACL reconstructions were normal or nearly normal in 11 knees and abnormal in 1 knee. All patients achieved at least 0° to 135° of knee motion; 1 required a gentle manipulation, and 1 had an arthroscopic lysis of adhesions to achieve this range of knee motion.
Femoral-Fibular Allograft Reconstruction
Two investigations were performed on the femoral-fibular allograft reconstruction for chronic instability.37 The first study followed 20 consecutive patients from 2 to 7.8 years postoperatively. Lateral stress radiographs and a comprehensive knee examination showed that 16 knees (76%) had a functional FCL and PL reconstruction and 5 failed. All knees but 1 had at least 0° to 135° of knee motion at follow-up, and no patient required an additional operation for a limitation of motion.
Proximal Advancement of PL Structures
A proximal advancement of the PL structures was done in conjunction with a cruciate ligament reconstruction in 23 consecutive patients.38 One patient was lost to follow-up. A second patient had an early failure and required a revision PL reconstruction; this result was included in the study’s overall failure rate.
Critical Points AUTHORS’ CLINICAL STUDIES: PROXIMAL ADVANCEMENT POSTEROLATERAL STRUCTURES
Causes of Failure of PL Operative Procedures
The potential causes of failure of 57 operative procedures (30 index and 27 revisions) to the PL structures of the knee were studied in a consecutive series of 30 knees that were referred to the authors’ center.39 The index PL procedures were done for an acute knee injury in 13 knees (mean, 3 wk; range, 1–11 wk after the injury) and for chronic deficiency in 17 knees a mean of 56 months (range, 4–312 mo) after the original injury.
Overall, for all 57 failed PL operations, nonanatomic graft procedures had been done in 23 knees (77%; Table 22-3). The definition of an anatomic reconstruction was a graft placed in anatomic ligament attachment sites with secure internal fixation. Therefore, suture repairs, extra-articular ITB augmentations, and biceps tendon rerouting methods (Fig. 22-23) were not considered anatomic procedures.
Untreated varus malalignment was identified in 21 failed PL procedures (37%) or in 10 of 30 knees (Tables 22-4 and 22-5). Patients who presented with PL deficiency and varus osseous malalignment were diagnosed with triple varus knees. Associated ACL, PCL, or bicruciate deficiency was identified in 27 knees (93%). ACL deficiency was identified in 41 of the 57 (72%) failed PL procedures and associated PCL deficiency was noted in 15 of the 57 (26%) failed PL procedures.
TABLE 22-4 Analysis of Treatment of Associated Cruciate Ligament Ruptures and Varus Malalignment in 21 Failed Posterolateral Procedures in Knees Treated for Acute Injuries
TABLE 22-5 Analysis of Treatment of Associated Cruciate Ligament Ruptures and Varus Malalignment in 36 Failed Posterolateral Procedures in Knees Treated for Chronic Injuries
Critical Points AUTHORS’ CLINICAL STUDIES: CAUSES OF FAILURE OF POSTEROLATERAL OPERATIVE PROCEDURES
IKDC, International Knee Documentation Committee; PL, posterolateral.
PL deficiency subjects ACL and PCL grafts to excessive tensile loading owing to the abnormal lateral tibiofemoral joint opening that occurs with activity. Several in vitro studies reported significantly increased forces on ACL and PCL grafts in knees with sectioned PL structures (see Chapter 20, Function of the Posterior Cruciate Ligament and Posterolateral Structures), providing further evidence of the deleterious effects of FCL and PMTL insufficiency after ACL or PCL reconstruction.
OTHER OPERATIVE TECHNIQUES AND RESULTS
Several authors have reported clinical outcome data from PL reconstructive procedures (Table 22-6).4,5,7,13,15,26,50,56 Stannard and colleagues50 followed 57 patients who received either a primary repair of acutely ruptured PL structures or a graft reconstruction of chronically deficient FCL, PFL, and popliteus tendon structures. At an average of 33 months postoperatively, 37% of the primary repair procedures had failed, compared with 9% of the graft reconstructions. The authors concluded that primary repair of the FCL is indicated only for bony avulsions that are amenable to internal fixation. Otherwise, graft reconstruction of the FCL is recommended, especially if an immediate knee motion program is to be used postoperatively.
Harner and coworkers13 and Chhabra and associates5 illustrated an FCL–Achilles tendon allograft reconstructive procedure and a PFL reconstruction. The FCL reconstruction was performed in 7 patients with knee dislocations, 2 of which failed to restore normal LJO. Buzzi and colleagues4 described an FCL reconstruction using a semitendinosus tendon autograft. In a group of 13 patients studied, all had normal or nearly normal restoration of LJO and external tibial rotation postoperatively.
Cooper and Stewart7 presented two operative options for PL ruptures. One consisted of a combined FCL and PFL reconstruction (with a semitendinosus tendon autograft) with capsular imbrication. The second option consisted of reconstruction of the PFL only in knees with an intact FCL. In a group of 19 patients with combined PCL and PL ruptures, no patient had greater than 10° of external rotation or more than 1+ increase in LJO postoperatively.
Latimer and coworkers26 described an anatomic FCL replacement using a B-PT-B allograft. In a cohort of 10 patients, all but 1 had restoration of normal or nearly normal LJO and external tibial rotation an average of 28 months postoperatively.
LaPrade and coworkers21 described an anatomic PL reconstruction (Fig. 22-24A and B). Biomechanical testing of this technique demonstrated restoration of normal knee motion limits to external tibial rotation and LJO. Clinical results of this technique are pending. The operation restores the anatomic attachment sites for the FCL and popliteus tendon, which as discussed in this chapter, allows load sharing between these two structures, which appears to have a distinct advantage over a single femoral-fibular graft reconstruction. The PL technique described by Larson and Belfie25 that uses a semitendinosus autograft is shown in Figure 22-24C. The operative procedure is designed to restore FCL function, as in acute operative cases. However, it does not restore the PMTL unit, which may be required in chronic PL reconstructions. A technique demonstrated in Figure 22-24D avoids creating two femoral tunnels that may potentially weaken the lateral femoral condyle. This procedure is considered advantageous in knees that have prior tunnels as in ACL revision surgery and the surgeon wishes to avoid an additional tunnel and, instead, use a single bone inlay technique for femoral graft fixation. An alternative technique using a single graft to replace the FCL and popliteus tendon described by Kim and associates17 is illustrated in Figure 22-24E.
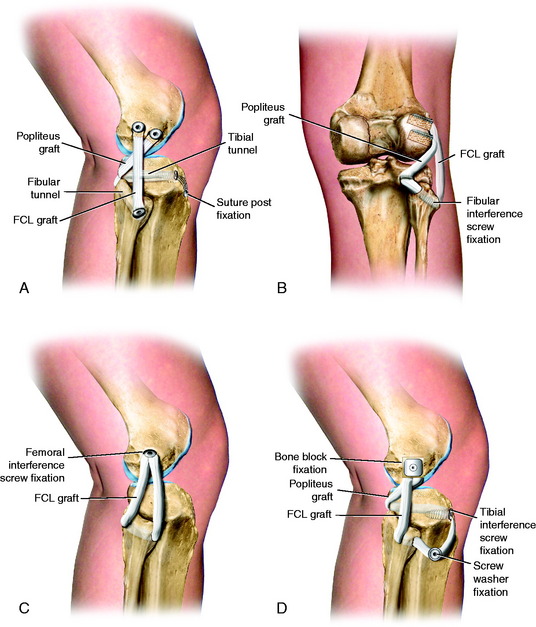
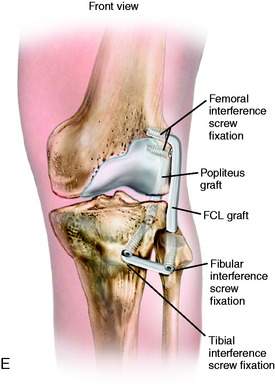
FIGURE 22-24 Alternative techniques for PL reconstructions. A and B, The anatomic PL reconstruction described by LaPrade and coworkers21 that uses Achilles tendon–bone allografts. A, The bone portion of the graft is placed at the femoral insertion of the FCL and the popliteus tendon. B, The popliteus graft is passed through a tunnel at the PL tibia. The FCL graft is passed through a fibular tunnel. C, PL technique described by Larson and Belfie25 that uses a semitendinosus autograft. D, A technique demonstrating a single Achilles tendon allograft in which a bone inlay is placed at the FCL femoral insertion. This technique avoids creating two femoral tunnels that may potentially weaken the lateral femoral condyle and is considered advantageous in knees that have prior tunnels from ACL surgery. E, Technique using a single graft to replace the FCL and popliteus tendon described by Kim and associates.17 The proximal ends of the graft are fixed by using bioabsorbable screws with EndoPearl (ConMed Linvatec, Largo, FL) devices.
Case 1 Revision of ACL and PL Failed Procedures in a Salvage Knee
Diagnostic arthroscopy performed before the revision ACL and PL procedure demonstrated an abnormal lateral tibiofemoral gap test (Fig. 22-25A), a deficient PMTL (see Fig. 22-25B), and fissuring and fragmentation of the articular cartilage (grade 2B damage; see Chapter 47, Articular Cartilage Rating Systems) in the patellofemoral, medial tibiofemoral, and lateral tibiofemoral compartments.
The patient underwent an ACL QT-PB autograft reconstruction using a notch approach owing to the close proximity of prior femoral tunnels that had not healed completely (but did not require bone grafting). In addition, an anatomic FCL B-PT-B allograft reconstruction, repair of the PFL, advancement of the popliteus tendon, and advancement of the PLC were performed (see Fig. 22-25C and D).
Case 2 Treatment of Combined Failure of ACL and PL Deficiencies in a Varus-Angulated Knee
A 35-year-old man presented 5 months after failure of a PL biceps tendon transfer procedure and ACL B-PT-B autograft reconstruction. AP (Fig. 22-26A) and lateral (see Fig. 22-26B) radiographs demonstrated abnormal expansion of both the femoral and the tibial tunnels and a vertical ACL graft. Physical examination revealed a grade III pivot shift, 7 mm of increased AP displacement on KT-2000 testing, and 10 mm of increased LJO. Full standing radiographs showed a weight-bearing line of 42%. The patient had not been able to return to work since his original injury and had moderate pain with daily activities.
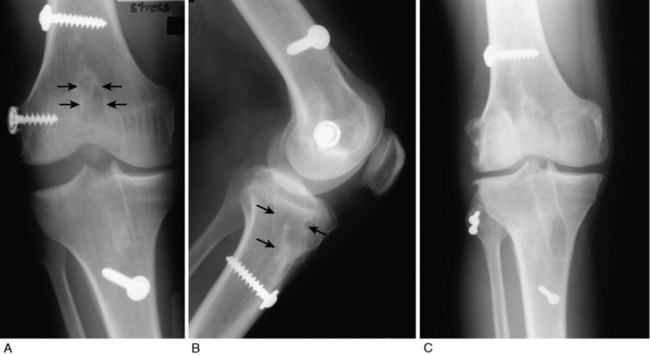
FIGURE 22-26 Case #2.
(From Noyes, F. R.; Barber-Westin, S. D.; Albright, J. C.: An analysis of the causes of failure in 57 consecutive posterolateral operative procedures. Am J Sports Med 34:1419–1430, 2006.)
The patient was treated with a staged approach of a corrective closing wedge HTO, followed 4 months later with an anatomic PL reconstruction consisting of an FCL B-PT-B allograft, a PMTL B-PT-B allograft, and a proximal advancement of the PL structures. In addition, an ACL QT-PB autograft revision reconstruction was performed that bypassed the previously placed vertical graft (see Fig. 22-26C). At the latest follow-up evaluation, 53 months postoperative, there was no increase in LJO or external tibial rotation and the pivot shift test was negative. The patient had returned to work and light recreational sports without problems.
Case 3 Treatment of Prior Multiple Failed PL Procedure in a Dislocated Knee
Physical examination revealed a grade III pivot shift, 8 mm of increased AP displacement on KT-2000 testing, 12 mm of increased LJO, and 20° of increased external tibial rotation. Stress radiographs demonstrated a severe increase in LJO (Fig. 22-27A). The patient also had 5 mm of increased medial joint opening and 5 mm of increased posterior tibial translation. He complained of pain and giving-way with daily activities and rated the overall condition of his knee as poor.
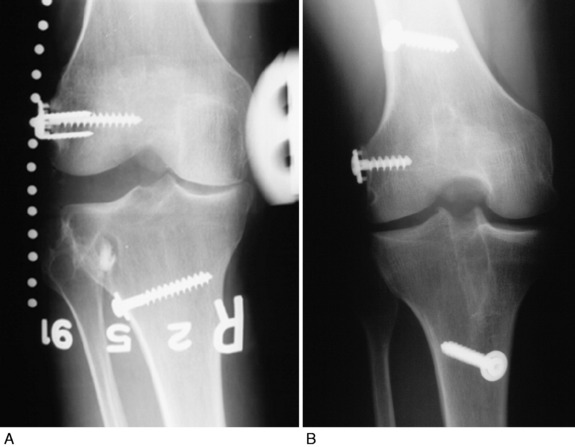
FIGURE 22-27 Case #3.
(From Noyes, F. R.; Barber-Westin, S. D.; Albright, J. C.: An analysis of the causes of failure in 57 consecutive posterolateral operative procedures. Am J Sports Med 34:1419–1430, 2006.)
The patient underwent an anatomic FCL B-PT-B autograft reconstruction (harvested from the contralateral knee) and a proximal advancement of the PL structures and popliteus tendon. An ACL QT-PB autograft was placed at the anatomic tibial and femoral attachment sites (see Fig. 22-27B). Fissuring and fragmentation (grade 2A damage) were noted in all three compartments.
At follow-up, 4 years postoperative, the patient had a full range of knee motion, no effusion, a grade 0 pivot shift, an increase of 5 mm of AP displacement on KT-2000 testing (at 20° of knee flexion), 2 mm of increase in posterior tibial translation on posterior stress radiographs (Fig. 22-28A and B), and no increase in lateral tibiofemoral opening on lateral stress radiographs (see Fig. 22-28C and D). He had successfully returned to skiing and mountain climbing without symptoms, had run a marathon, and rated the overall condition of his knee as very good.
At follow-up, 4 years postoperative, the patient had a normal range of knee motion, an increase of 3 mm in AP displacement on KT-2000 testing, no increase in LJO on stress radiographs (Fig. 22-29A and B), and a grade 0 pivot shift. He had returned to basketball, baseball, and football without symptoms or limitations and rated the overall condition of his knee as normal.
At follow-up, 12 years postoperative, the patient had a normal range of knee motion, no effusion, 4 mm of increased AP displacement on KT-2000 testing, 2 mm of increase in LJO on stress radiographs on the involved left knee (Fig. 22-30A) compared with the right knee (see Fig. 22-30B), and a grade 0 pivot shift. Weight-bearing PA radiographs showed preservation of tibiofemoral joint space in both compartments compared with the contralateral limb (see Fig. 22-30C). He had no symptoms with low-impact athletic activities or with his occupation and rated the overall condition of his knee as good.
The patient underwent a multiligament reconstruction consisting of an ACL B-PT-B allograft, a femoral-fibular FCL Achilles tendon allograft, a proximal advancement of the PLC, and a repair of a complex medial meniscus tear. She did well, but sustained a reinjury 16 years later that required a medial meniscus repair. At the most recent follow-up, 19 years postoperative, the patient had a normal range of knee motion, no tibiofemoral compartment pain, an increase of 3 mm of AP displacement on KT-2000 testing, a grade I pivot shift, and 2 mm of increase in LJO on stress radiographs (Fig. 22-31A) compared with the contralateral limb (see Fig. 22-31B). She had no symptoms with low-impact athletic activities and rated the overall condition of her knee as good.
At follow-up, 18 years postoperative, the patient had a normal range of knee motion, no tibiofemoral compartment pain, 4 mm of increased AP displacement on KT-2000 testing, no increase in medial joint opening, and no increase in LJO on stress radiographs on the right knee (Fig. 22-32A) compared with the left knee (see Fig. 22-32B). He had returned to basketball and construction work without limitations and rated the overall condition of his knee as normal.
Authors’ comment: This case demonstrates an unexpected good functional outcome despite articular cartilage damage at the index procedure. This patient was part of a prospective study initiated 18 years previously on ACL allografts. An autograft approach discussed in Chapter 7, Anterior Cruciate Ligament Primary and Revision Reconstruction: Diagnosis, Operative Techniques, and Clinical Outcomes, has proved to have a higher success rate, and as a result, ACL allografts are used only under select situations.
At follow-up, 16 years postoperative, the patient had no effusion, a normal range of knee motion, 1 mm of increase in AP displacement on KT-2000 testing, a grade 1 pivot shift, 5 mm of increase in LJO on stress radiographs on the left knee (Fig. 22-33A) compared with the right knee (see Fig. 22-33B), and no increase in medial joint opening. He had no symptoms or limitations with basketball, running, and his occupation as a chef and rated the overall condition of his knee as very good.
A 15-year-old male presented 3 weeks after a knee dislocation sustained during football. The patient was placed into an immobilizer but had not undergone any further medical treatment for his injury. Physical examination revealed moderate swelling, only 20° of knee motion, a loss of sensation over the lateral aspect of the leg as well as the dorsum of the foot, and a complete foot drop with 0 to 5 dorsiflexion and 0 to 5 extension of the great toe. His vascular status was intact. MRI revealed a torn ACL, PCL, FLC, and PL structures. He underwent 2 months of supervised physical therapy. A staged global reconstruction was then performed involving first an ACL B-PT-B allograft and arthroscopic PCL two-strand B-PT-B allograft reconstruction. One week later, an FCL B-PT-B allograft reconstruction and a PFL and popliteus tendon reconstruction were done (Fig. 22-34A and B).
Three years postoperative, the patient had a negative pivot shift, no increase in anterior tibial translation, no increase in external tibial rotation, and no increase in medial or LJO. He had a neurovascularly intact lower extremity and a full range of knee motion. Lateral (see Fig. 22-34C) and posterior (see Fig. 22-34D) stress radiographs showed no increase in LJO or posterior tibial translation. The patient had returned to recreational basketball and running without symptoms. He rated the overall condition of his knee as normal.
1 Baker C.L.Jr., Norwood L.A., Hughston J.C. Acute posterolateral rotatory instability of the knee. J Bone Joint Surg Am. 1983;65:614-618.
2 Barber-Westin S.D., Noyes F.R., McCloskey J.W. Rigorous statistical reliability, validity, and responsiveness testing of the Cincinnati Knee Rating System in 350 subjects with uninjured, injured, or anterior cruciate ligament-reconstructed knees. Am J Sports Med. 1999;27:402-416.
3 Bottomley N., Williams A., Birch R., et al. Displacement of the common peroneal nerve in posterolateral corner injuries of the knee. J Bone Joint Surg Br. 2005;87:1225-1226.
4 Buzzi R., Aglietti P., Vena L.M., Giron F. Lateral collateral ligament reconstruction using a semitendinosus graft. Knee Surg Sports Traumatol Arthrosc. 2004;12:36-42.
5 Chhabra A., Cha P.S., Rihn J.A., et al. Surgical management of knee dislocations. Surgical technique. J Bone Joint Surg Am. 2005;87(suppl 1 pt 1):1-21.
6 Cooper D.E. Tests for posterolateral instability of the knee in normal subjects. Results of examination under anesthesia. J Bone Joint Surg Am. 1991;73:30-36.
7 Cooper D.E., Stewart D. Posterior cruciate ligament reconstruction using single-bundle patella tendon graft with tibial inlay fixation: 2- to 10-year follow-up. Am J Sports Med. 2004;32:346-360.
8 DeLee J.C., Riley M.B., Rockwood C.A.Jr. Acute posterolateral rotatory instability of the knee. Am J Sports Med. 1983;11:199-207.
9 Dellon A.L., Ebmer J., Swier P. Anatomic variations related to decompression of the common peroneal nerve at the fibular head. Ann Plast Surg. 2002;48:30-34.
10 Dugdale T.W., Noyes F.R., Styer D. Preoperative planning for high tibial osteotomy: the effect of lateral tibiofemoral separation and tibiofemoral length. Clin Orthop Relat Res. 1992;274:248-264.
10a Fanelli G.C., Edson C.J. Arthroscopically assisted combined anterior and posterior cruciate ligament reconstruction in the multiple ligament injured knee: 2- to 10-year follow-up. Arthroscopy. 2002;18:703-714.
10b Fanelli G.C., Edson C.J. Combined posterior cruciate ligament-posterolateral reconstructions with Achilles tendon allograft and biceps femoris tendon tenodesis: 2- to 10-year follow-up. Arthroscopy. 2004;20:339-345.
11 Gollehon D.L., Torzilli P.A., Warren R.F. The role of the posterolateral and cruciate ligaments in the stability of the human knee. A biomechanical study. J Bone Joint Surg Am. 1987;69:233-242.
12 Grood E.S., Noyes F.R., Butler D.L., Suntay W.J. Ligamentous and capsular restraints preventing straight medial and lateral laxity in intact human cadaver knees. J Bone Joint Surg Am. 1981;63:1257-1269.
13 Harner C.D., Waltrip R.L., Bennett C.H., et al. Surgical management of knee dislocations. J Bone Joint Surg Am. 2004;86:262-273.
14 Hewett T.E., Noyes F.R., Lee M.D. Diagnosis of complete and partial posterior cruciate ligament ruptures. Stress radiography compared with KT-1000 arthrometer and posterior drawer testing. Am J Sports Med. 1997;25:648-655.
15 Jung Y.B., Tae S.K., Jung H.J., Lee K.H. Replacement of the torn posterior cruciate ligament with a mid-third patellar tendon graft with use of a modified tibial inlay method. J Bone Joint Surg Am. 2004;86:1878-1883.
15a Jung Y.B., Jung H.J., Kim S.J., et al. Posterolateral corner reconstruction for posterolateral rotatory instability combined with posterior cruciate ligament injuries: comparison between fibular tunnel and tibial tunnel techniques. Knee Surg Sports Traumatol Arthrosc. 2008;16:239-248.
16 Kadiyala R.K., Ramirez A., Taylor A.E., et al. The blood supply of the common peroneal nerve in the popliteal fossa. J Bone Joint Surg Br. 2005;87:337-342.
17 Kim S.J., Park I.S., Cheon Y.M., Ryu S.W. New technique for chronic posterolateral instability of the knee: posterolateral reconstruction using the tibialis posterior tendon allograft. Arthroscopy. 2004;20(suppl 2):195-200.
18 LaPrade R.F. Anatomic reconstruction of the posterolateral aspect of the knee. J Knee Surg. 2005;18:167-171.
19 LaPrade R.F., editor. Posterolateral Knee Injuries. Anatomy, Evaluation, and Treatment. New York: Thieme; 2006:238.
20 LaPrade R.F., Heikes C., Bakker A.J., Jakobsen R.B. The reproducibility and repeatability of varus stress radiographs in the assessment of isolated fibular collateral ligament and grade-III posterolateral knee injuries. An in vitro biomechanical study. J Bone Joint Surg Am. 2008;90:2069-2076.
21 LaPrade R.F., Johansen S., Wentorf F.A., et al. An analysis of an anatomical posterolateral knee reconstruction: an in vitro biomechanical study and development of a surgical technique. Am J Sports Med. 2004;32:1405-1414.
22 LaPrade R.F., Ly T.V., Wentorf F.A., Engebretsen L. The posterolateral attachments of the knee: a qualitative and quantitative morphologic analysis of the fibular collateral ligament, popliteus tendon, popliteofibular ligament, and lateral gastrocnemius tendon. Am J Sports Med. 2003;31:854-860.
23 LaPrade R.F., Terry G.C. Injuries to the posterolateral aspect of the knee. Association of anatomic injury patterns with clinical instability. Am J Sports Med. 1997;25:433-438.
24 LaPrade R.F., Wentorf F.A., Fritts H., et al. A prospective magnetic resonance imaging study of the incidence of posterolateral and multiple ligament injuries in acute knee injuries presenting with a hemarthrosis. Arthroscopy. 2007;23:1341-1347.
25 Larson R.V., Belfie D.J. Lateral collateral ligament reconstruction utilizing semitendinosus tendon. Tech Knee Surg. 2003;2:190-199.
26 Latimer H.A., Tibone J.E., ElAttrache N.S., McMahon P.J. Reconstruction of the lateral collateral ligament of the knee with patellar tendon allograft. Report of a new technique in combined ligament injuries. Am J Sports Med. 1998;26:656-662.
27 Malinin T.I., Levitt R.L., Bashore C., et al. A study of retrieved allografts used to replace anterior cruciate ligaments. Arthroscopy. 2002;18:163-170.
28 Markolf K.L., Graves B.R., Sigward S.M., et al. Effects of posterolateral reconstructions on external tibial rotation and forces in a posterior cruciate ligament graft. J Bone Joint Surg Am. 2007;89:2351-2358.
29 Markolf K.L., Graves B.R., Sigward S.M., et al. How well do anatomical reconstructions of the posterolateral corner restore varus stability to the posterior cruciate ligament-reconstructed knee? Am J Sports Med. 2007;35:1117-1122.
30 Markolf K.L., Graves B.R., Sigward S.M., et al. Popliteus bypass and popliteofibular ligament reconstructions reduce posterior tibial translations and forces in a posterior cruciate ligament graft. Arthroscopy. 2007;23:482-487.
31 Nielsen S., Ovesen J., Rasmussen O. The posterior cruciate ligament and rotatory knee instability. An experimental study. Arch Orthop Trauma Surg. 1985;104:53-56.
32 Nielsen S., Rasmussen O., Ovesen J., Andersen K. Rotatory instability of cadaver knees after transection of collateral ligaments and capsule. Arch Orthop Trauma Surg. 1984;103:165-169.
33 Noyes F.R., Barber S.D., Simon R. High tibial osteotomy and ligament reconstruction in varus-angulated, anterior cruciate ligament–deficient knees. A two- to seven-year follow-up study. Am J Sports Med. 1993;21:2-12.
34 Noyes F.R., Barber-Westin S.D. Posterior cruciate ligament revision reconstruction, part 1: causes of surgical failure in 52 consecutive operations. Am J Sports Med. 2005;33:646-654.
35 Noyes F.R., Barber-Westin S.D. Posterolateral knee reconstruction with an anatomical bone–patellar tendon–bone reconstruction of the fibular collateral ligament. Am J Sports Med. 2007;35:259-273.
36 Noyes F.R., Barber-Westin S.D. Revision anterior cruciate surgery with use of bone–patellar tendon–bone autogenous grafts. J Bone Joint Surg Am. 2001;83:1131-1143.
37 Noyes F.R., Barber-Westin S.D. Surgical reconstruction of severe chronic posterolateral complex injuries of the knee using allograft tissues. Am J Sports Med. 1995;23:2-12.
38 Noyes F.R., Barber-Westin S.D. Surgical restoration to treat chronic deficiency of the posterolateral complex and cruciate ligaments of the knee joint. Am J Sports Med. 1996;24:415-426.
39 Noyes F.R., Barber-Westin S.D., Albright J.C. An analysis of the causes of failure in 57 consecutive posterolateral operative procedures. Am J Sports Med. 2006;34:1419-1430.
40 Noyes F.R., Barber-Westin S.D., Hewett T.E. High tibial osteotomy and ligament reconstruction for varus-angulated anterior cruciate ligament–deficient knees. Am J Sports Med. 2000;28:282-296.
41 Noyes F.R., Barber-Westin S.D., Rankin M. Meniscal transplantation in symptomatic patients less than fifty years old. J Bone Joint Surg Am. 2004;86:1392-1404.
42 Noyes F.R., Barber-Westin S.D., Roberts C.S. Use of allografts after failed treatment of rupture of the anterior cruciate ligament. J Bone Joint Surg Am. 1994;76:1019-1031.
43 Noyes F.R., Dunworth L.A., Andriacchi T.P., et al. Knee hyperextension gait abnormalities in unstable knees. Recognition and preoperative gait retraining. Am J Sports Med. 1996;24:35-45.
44 Noyes F.R., Grood E.S., Torzilli P.A. Current concepts review. The definitions of terms for motion and position of the knee and injuries of the ligaments. J Bone Joint Surg Am. 1989;71:465-472.
45 Noyes F.R., Schipplein O.D., Andriacchi T.P., et al. The anterior cruciate ligament–deficient knee with varus alignment. An analysis of gait adaptations and dynamic joint loadings. Am J Sports Med. 1992;20:707-716.
46 Noyes F.R., Stabler C.L. A system for grading articular cartilage lesions at arthroscopy. Am J Sports Med. 1989;17:505-513.
47 Pasque C., Noyes F.R., Gibbons M., et al. The role of the popliteofibular ligament and the tendon of popliteus in providing stability in the human knee. J Bone Joint Surg Br. 2003;85:292-298.
48 Rodeo S.A., Arnoczky S.P., Torzilli P.A., et al. Tendon-healing in a bone tunnel. A biomechanical and histological study in the dog. J Bone Joint Surg Am. 1993;75:1795-1803.
49 Rubel I.F., Schwarzbard I., Leonard A., Cece D. Anatomic location of the peroneal nerve at the level of the proximal aspect of the tibia: Gerdy’s safe zone. J Bone Joint Surg Am. 2004;86:1625-1628.
50 Stannard J.P., Brown S.L., Farris R.C., et al. The posterolateral corner of the knee: repair versus reconstruction. Am J Sports Med. 2005;33:881-888.
51 Staubli H.U. Posteromedial and posterolateral capsular injuries associated with posterior cruciate ligament insufficiency. Sports Med Arthrosc Rev. 1994;2:146-164.
52 Strauss E.J., Ishak C., Inzerillo C., et al. Effect of tibial positioning on the diagnosis of posterolateral rotatory instability in the posterior cruciate ligament-deficient knee. Br J Sports Med. 2007;41:481-485. discussion 485
53 Terry G.C., LaPrade R.F. The biceps femoris muscle complex at the knee. Its anatomy and injury patterns associated with acute anterolateral-anteromedial rotatory instability. Am J Sports Med. 1996;24:2-8.
54 Veltri D.M., Deng X.H., Torzilli P.A., et al. The role of the cruciate and posterolateral ligaments in stability of the knee. A biomechanical study. Am J Sports Med. 1995;23:436-443.
55 Veltri D.M., Warren R.F. Anatomy, biomechanics, and physical findings in posterolateral knee instability. Clin Sports Med. 1994;13:599-614.
55a Wang C.J., Chen H.S., Huang T.W., Yuan L.J. Outcome of surgical reconstruction for posterior cruciate and posterolateral instabilities of the knee. Injury. 2002;33:815-821.
56 Zhao J., He Y., Wang J. Anatomical reconstruction of knee posterolateral complex with the tendon of the long head of biceps femoris. Am J Sports Med. 2006;34:1615-1622.