Chapter 28 Posterior Lumbar Foraminal Decompression
Since the first publication by Hijikata and colleagues [1] in 1975 on purely percutaneous intradiscal lumbar disc decompression, several developments have been made with this approach for different forms of lumbar, and later also cervical, disc pathologies. The original Hijikata technique, developed for Japanese patients, was adopted in Zurich after its presentation at the 1978 SICOT (Société Internationale de Chirurgie Orthopédique et de Traumatologie) meeting in Kyoto, where it was introduced as a unilateral posterolateral decompression technique for contained lumbar disc herniations. It became evident that the sizes of the original instruments would require adaptation to the larger dimensions of anatomical structures in European patients. Specifically, a stepwise modification of the diameter of the dilating cannulas was needed to reach a working channel of 6 mm. With the larger cannula, the introduction of arthroscopic control became possible in 1982. This arthroscopic control, when used with a biportal cannula approach [2], for the first time allowed simultaneous endoscopic control, or discoscopy [3], of intradiscal selective tissue removal in the posterior field of the intervertebral disc space. Through this process, the indirect decompression of subligamentarily contained lumbar disc herniation was standardized in its specific range of indications.
Other surgeons aimed for technical improvement of uniportal intradiscal disc decompression by higher abrasive activity, with new devices such as the automated nucleotome introduced in 1985 by Onik and associates [4]. Its ease of application made disc surgery available to a larger group, including nonsurgical physicians, explaining the somewhat uncontrolled boom of percutaneous disc decompression in the later 1980s. This overuse with unconvincing results contributed to a rapid decline in the reputation of percutaneous methods for disc decompression in general. Because the slim aspiration cannula vacuumed disc tissue from any direction, limited control of the direction of disc tissue removal was possible. The tissue removed was not necessarily aspirated from the posterior subanular zone. The same inherent problem existed with later centrodiscal ablative laser disc decompression, which clearly limited this application to indirect decompression of symptomatic contained protrusions and did not allow for calculable tissue removal in the posterior subligamentary zone. Such calculable removal was better achieved through biportal endoscopically controlled mechanical tissue extraction with pituitary forceps under endoscopic control. In this approach, direct visualization of tissue fragment removal became available and challenged conventional microdiscectomy in this range of indications. Owing to this experience, it became evident as early as the 1980s that intradiscal techniques could not be adapted for adequate removal of any transligamentary sequestrated herniation because of geometric constrictions. In these cases, conventional open techniques, such as minimal interlaminotomy or microdiscectomy [5], remained the “gold standards.”
Endoscopic applications in the area inside the anulus fibrosus were in clinical use with biportal percutaneous nucleotomy beginning in the mid-1980s, but by its nature the technique remained without direct therapeutic use for extradiscal tissue removal. In the United States, Kambin used his anuloscopy for medicolegal reasons from 1988 on for endoscopic documentation of the anular fibers before their trepanation with his trephine-cutter, which was then his standard method for uniportal entrance into the lumbar disc with decompressive forceps [13]. With this method he could avoid damage to the exiting roots during anular trepanation. His technique was further developed by Savitz [6] in the 1990s, in combination with laser-assisted fluoroscopic microdiscectomy. In our experience, the laser application did not offer convincing advantages over mechanical tissue removal with a biportal approach, but others found it advantageous for tissue shrinking in coaxial unilateral applications [7,8].
In 1990, on the basis of our experience with minimally invasive techniques such as intradiscal endoscopic control for pointed mechanical tissue removal, we tried to explore the anular and foraminal site locally at the end of our biportal interventions. However, with the 7.5-mm working cannula that was available at the time, such exploration was not successful. During the same period, we made the first pathoanatomical investigations into the use of rod-lens coaxial endoscopic devices derived from integrated cystoscopes for use in non-prefigured retroperitoneal compartments. These devices were the precursors of a 6-mm, open coaxial endoscopic technique for direct visualization of foraminal sequestrated herniations, introduced clinically for the first time worldwide in 1991 [9,10].
Development of similar methods followed in the United States in 1993 for lumbar decompressive applications. Later, the uniportal coaxial endoscopic procedure was adopted by others, such as Yeung and Chow [8], who developed a similar system with a slightly smaller working channel but a second channel for laser fiber application as an additional working tool for disc decompression and bony enlargement of the foraminal access (Fig. 28-1).
Anatomical and technical considerations
For the clinical presentation of typical dysesthetic radicular pain (e.g., pretibial burning sensation in a L4-L5 foraminal compression), the compression of the sensory ganglion beyond the pedicle by ascending foraminal disc herniation is the typical anatomical correlation (Fig. 28-2). Affected patients, without additional herniation in the canal and therefore without compression of the lateral recess, hardly ever show root tension signs, such as positive response to Lasègue maneuver.
Indications
The target indication for foraminoscopic microdiscectomy since its introduction in 1991 has been lumbar foraminal and extraforaminal disc herniation with clinical root/ganglion compression syndrome that is resistant to conservative treatment or is rapidly evolving under conservative treatment. The incidence of this condition in our population is around 10% of all operative lumbar disc cases [11].
The diagnosis of this type of herniation is often delayed because it occurs outside the lumbar canal [5] and therefore often does not correspond to classic clinical signs, such as a positive response to Lasègue root tension maneuver, nighttime pain, and pain aggravated by lying on the side opposite the herniation site.
Radiologic Diagnosis
The best diagnostic tools are computed tomography (CT) and magnetic resonance imaging (MRI):

Operating room setup and anesthesia
Foraminoscopic microdiscectomy is performed exclusively under aseptic laminar-flow operating room conditions, like any other form of discectomy (Fig. 28-3).
Procedure
In more obese patients, a CT slice obtained with a wide window including the soft tissue and skin at the respective disc level in prone position before surgery can help in calculating the lateral distance, thus avoiding the use of instruments that are too short (Fig. 28-5).
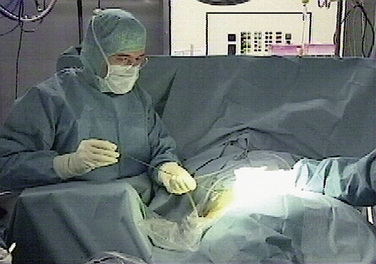
Figure 28–6 Introduction of guidewire and subsequent overslipping sleeves under fluoroscopic guidance.
Conclusion
Since its clinical introduction in 1991, endoscopic decompression of foraminal to far lateral disc sequestrations has become our treatment of choice for these specific indications. The procedure is competitive in its results with conventional open operative procedures with interlaminar or posterolateral approach [12], and it provides the added benefit of being minimally invasive with little blood loss and a very small skin incision.
The main benefit is the stability-sparing posterolateral approach with direct targeting to the sequestrated tissue fragment without touching the spinal canal. As we have seen over the years, the learning curve for preoperative patient selection and the intraoperative handling of the instruments is considerable. High-quality preoperative imaging analysis and three-dimensional knowledge of the local surrounding anatomical structures provided by a patho-anatomical workup is mandatory. In well-trained hands this procedure is today a safe surgical option for the treatment of foraminal compression syndromes and a valuable complement to existing minimal interlaminar techniques [7,11].
1 Hijikata S., Yamagishi M., Nakayama T., Oomori K. Percutaneous discectomy: a new treatment method for lumbar disc herniation [Japanese]. J Toden Hosp. 1975;39:5-13.
2 Schreiber A., Suezawa Y., Leu H.J. Does percutaneous nucleotomy with discoscopy replace conventional discotomy? Clin Orthop Relat Res. 1989;238:35-42.
3 Schreiber A., Leu H.J. Biportal percutaneous lumbar nucleotomy: development, technique and evolutions. In: Kambin P., editor. Arthroscopic Microdiscectomy. Baltimore: Urban & Schwarzenberg, 1990.
4 Onik G., Helms C.A., Ginsburg L., et al. Percutaneous lumbar discectomy using a new aspiration probe. AJR Am J Roengtenol. 1985;6:90-293.
5 McCulloch J.A. The posterolateral approach. In: Mayer H.M., Brock M., editors. Discectomy PercutaneousLumbar. Berlin: Springer, 1989.
6 Savitz M.H. Same day microsurgical arthroscopic lateral approach laser assisted (small) fluoroscopic discectomy. J Neurosurg. 1994;8:1039-1045.
7 Chiu J., Clifford T. Multiple herniated discs at single and multiple spinal segments treated with endoscopic microdecompressive surgery. J Minim Invas Spinal Tech. 2001;1:54-57.
8 Yeung A.T., Chow P.M. Posterior lateral endoscopic excision for lumbar disc herniation: surgical technique, outcome, and complications. Spine. 2002;27:722-732.
9 Leu H.J., Schreiber A. Diskoskopie: optische dimension perkutaner bandscheibenchirurgie. Arthroskopie. 1993;3:3-9.
10 Leu H.J., Hauser R. Die perkutan posterolaterale foraminoskopie: Prinzip, technik und erfahrungen seit 1991. Arthroskopie. 1996;9:26-31.
11 Benini A., Steinsieppe K.F. Die laterale intra- und extraforaminale lumbale diskushernie: Klinik und therapie. Schweiz Med Wschr. 1991;121:889-897.
12 Leu H.F. Posterolateral foraminoscopic microdiscectomy. In: Savitz M., Chiu J., Rauschning W., Yeung A., editors. The Practice of Minimally Invasive Spinal Technique. 2005 ed. New York: AAMISS Press; 2005:463-467.
13 Kambin P., Schaffer J.L. Percutaneous lumbar discectomy. Review of 100 patients and current practice. Clin Orthop Relat Res. 1989;238(Jan):24-34.