CHAPTER 53 Posterior Dynamic Stabilization*
Degenerative disorders in the lumbar spine can lead to an abnormal pattern of motion causing segmental instability and low back pain, although the precise relationship between instability and low back pain with or without leg pain has not been established. The standard of care for the surgical treatment of degenerative instability causing low back pain has been fusion with or without instrumentation.1 More recent advances in fusion techniques have increased the rate of successful fusion, without an equivalent improvement in relief of pain. The fusion rate with modern technology approaches 100%, but successful pain relief does not exceed 60% to 70%. A solid fusion does not always result in a successful clinical outcome.2,3 A fusion can have long-term effects on unfused segments, leading to accelerated degeneration of the adjacent segments, engendered by abnormal forces and motion at those segments.4,5 Because of these limitations of fusion, dynamic stabilization was introduced in the treatment of activity-related mechanical low back pain.
Instability
The rationale for fusing the painful motion segment has been the concept of instability.6 Understanding and diagnosis of clinical instability remains controversial. When abnormal motion is present on flexion-extension radiographs, especially in the setting of spondylolisthesis, fusion is accepted as a reasonable option.7 By this standard, relatively few patients with low back pain have overt subjective or objective evidence of instability, however. Pope and Panjabi8 suggested that instability is a mechanical entity and is defined as a loss of stiffness to a given load. Biomechanical and radiologic studies using open magnetic resonance imaging (MRI) in flexion and extension have shown, however, that segmental motion either does not change significantly with disc degeneration9–11 or may decrease except during early stages of disc degeneration.12
Mulholland and Sengupta13 suggested that spinal instability does not mean “increased motion” as commonly misunderstood,8,14 but rather it indicates abnormal load distribution across the vertebral endplate. The pathologic changes within the disc space may result in abnormal transmission of load across the endplates. It has been well established in other weight-bearing joints that abnormal load transmissions result in degenerative changes, and the resultant pain may be diminished by a properly placed osteotomy to realign the joint forces and redistribute the loads.15 The normal disc consists of a homogeneous gel of collagen and proteoglycan. The normal disc is isotropic, similar to a fluid-filled bag, a property that allows it to transmit load uniformly across the vertebral endplates.13
Disc degeneration alters the isotropic properties of the disc. The disc becomes nonhomogeneous, with areas of fragmented and condensed collagen, cartilage fragments, fluid, and gas.16 Load transmission over the endplates becomes uneven. The nucleus becomes depressurized, and an increasingly larger load is transmitted through the anulus, which leads to splitting and inward folding of the anulus.17 The central area of the endplate overlying the depressurized nucleus now transmits lesser load, and corresponding endplate changes, such as destruction, thinning of the trabeculae, and thinning of the cartilaginous endplate,18,19 may be noted. Focal loading of the endplate cartilage and subchondral bony trabeculae can occur with certain positions, leading to a sharp increase in back pain. This situation is analogous to a “stone in the shoe” causing high spot loading and pain, a concept proposed by Mulholland and Sengupta.13
This concept explains the clinical sign of instability catch as experienced by patients with mechanical back pain secondary to disc degeneration during flexion-extension movement. This hypothesis also explains why manipulation of the lumbar spine by a chiropractor may occasionally dislodge the fragment from its weight-bearing position, bringing an immediate relief of acute low back pain.13 Mulholland and Sengupta’s hypothesis of abnormal loading as the primary cause of mechanical back pain was supported by a close association of abnormal disc pressure profiles with positive discography with pain provocation.20 A pressure profilometry study by McNally and Adams21 revealed the anisotropic properties of the degenerated disc. This study showed that the pattern of loading, rather than the absolute levels of loading, was related to pain generation in the degenerated spine. The concept may also help explain the lack of correlation between degrees of disc degeneration and back pain because individual anatomic and consequential load transmission changes vary from one person to another. With advancing age, the continued progression of these changes results in the disc becoming more collagenized and homogeneous. The very aged disc may distribute loads more evenly again, resulting in a degree of spontaneous relief of pain with time.22
Panjabi23 described spinal stability in vivo as a function of three subsystems: (1) the spinal column, (2) the spinal muscles, and (3) the neural control unit. In vivo, spinal muscle action and neural control may be equal or more important spinal stabilizers, but the focus of surgical treatment of back pain has been the passive stability provided by the spinal column itself. Instability in the spinal column may result from damage or degeneration of bone, joints, or ligaments, which may be characterized by an increased motion in its early stages. By the time the pain becomes severe enough to consider surgical intervention, the total range of motion is often diminished or abnormal in quality.24 Panjabi23 redefined spinal instability as an abnormal motion often accompanied by an increased neutral zone motion caused by ligament laxity, even when the range of motion is diminished. Panjabi used the analogy of a marble rolling on a soup bowl (Fig. 53–1).
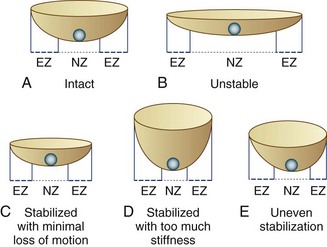
FIGURE 53–1 Panjabi’s23 analogy of a “marble on a soup bowl,” explaining relationship between spinal instability and change in range of motion. A, Intact spine. The marble can move with little resistance across neutral zone (NZ) but faces increasing resistance toward elastic zone (EZ). B, Unstable motion segment. The bowl is flat allowing the marble to move to and fro with little resistance—that is, increased NZ with no increase in EZ. C, Stabilized segment. A smaller cup reduces primarily NZ, with minimal reduction of EZ movement. D, Stabilized segment—but too much loss of motion, which is undesirable. E, Uneven stabilization—with different stiffness in two directions, which is again undesirable.
Definitions
Soft fusion is a newer term that refers to posterior spinal fusion with minimal stiffness. Some biomechanical and clinical studies indicate the rigidity of lumbar fusion may vary depending on fusion techniques.25,26 The theory behind the concept of soft fusion is that intertransverse process fusions using PDS devices may achieve a less rigid fusion compared with conventional rigid fusion with fusion rods or interbody devices and may be less detrimental to the adjacent segment. At this time, there is no clinical evidence supporting the concept of soft fusion. This concept has evolved from the fact that many PDS devices obtain U.S. Food and Drug Administration (FDA) approval under 510K for spinal fusion only and not for nonfusion stabilization. Use of these devices as dynamic stabilization devices without fusion represents off-label use.
Biomechanical Goals for Posterior Dynamic Stabilization
Load Transmission
The mechanism of pain relief with dynamic stabilization may be unloading the disc and the facet joints by load sharing, preventing abnormal load distribution and high spot loading.13 How much load should be shared by the device is unclear. It may be assumed, however, that the device would reduce the intradiscal pressure by the magnitude of load sharing.
Resistance to Fatigue Failure
The motion restriction should be uniform throughout the range of motion. If the device may cause too much restriction of motion in any direction, most commonly acting like an extension stop, the device may be exposed to repeatedly unusually high stress in extension and may eventually fail (Fig. 53–2). Similarly, the load sharing should be uniform throughout the range of motion. If the device may unload the disc too much in one direction, often in extension, the device has to bear unusually high load during that motion and is likely to fail (Fig. 53–3).
Normally, the disc pressure increases in flexion and extension and is lowest in neutral position. A PDS device ideally should permit an increase in the disc pressure in flexion and extension but to a smaller magnitude owing to load sharing (see Fig. 53–3). If the disc pressure does not increase at all, particularly in extension, it may indicate that the device is acting like a total load-bearing structure during extension rather than as a load-sharing structure. Similarly, a mismatch in the location of the instant axis of rotation of the device and the motion segment is another predictor of fatigue failure of the device.
Pedicle-to-Pedicle Distance Excursion
A normal pedicle-to-pedicle excursion during flexion-extension may be 6 to 9 mm, less in lateral bending, and minimal in rotation (Fig. 53–4). The device should permit a normal pedicle-to-pedicle distance excursion to permit normal range of motion and ensure that the device should not act as a “motion stopper” in any direction. Only a few dynamic stabilization devices can accommodate such a large degree of flexibility.
Other Design-Related Factors
Other design-related factors are as follows:
Development of any new device should preferably have an option for an easy salvage in case of failure. Top-loading pedicle screws, accepting regular fusion rods, make implantation simpler and conversion to a fusion more straightforward. Need for in situ tensioning of the device often requires a longer exposure. Posterior devices are inherently kyphogenic, and active lordosis must be built into the design (Fig. 53–5).
Dynamic Stabilization Devices
The classification of dynamic stabilization devices is difficult because new devices are always being introduced, and devices are being constantly withdrawn. As defined earlier, only PDS and IPD devices are included in the classification presented in Table 53–1. The semirigid fixation devices and prosthetic devices, including facet replacement devices, were excluded.
TABLE 53–1 Classification of Posterior Dynamic Stabilization Devices
Pedicle Screw–Based Posterior Dynamic Stabilization Devices |
Buy Membership for Orthopaedics Category to continue reading. Learn more here
|