CHAPTER 57 Pleura, lungs, trachea and bronchi
The lungs are the essential organs of respiration and are responsible for the uptake of oxygen into the blood and the removal of carbon dioxide. The functional design of the thorax facilitates this complex process. The muscles of respiration and the diaphragm, acting together, increase the intrathoracic volume, creating a negative pressure within the pleural space which surrounds the lung and causing expansion of the lung (see Ch. 58). The resultant reduction in intra-alveolar pressure prompts the conduction of air through the upper respiratory tract into the trachea and airways and thence into the alveoli, where gaseous exchange occurs. The process of breathing exposes the lung to noxious agents, including gases, dust particles, bacteria and viruses; the mucous barrier, mucociliary escalator, branching pattern of the airways and the cough reflex are all anatomical defences against these insults. Anatomical defects may compromise respiratory function; for example, chest wall abnormalities may cause restrictive lung disease. Similarly, ultrastructural abnormalities such as ciliary dysfunction (as seen in Kartagener’s syndrome) lead to recurrent respiratory infections and airway damage.
PLEURA
The right and left pleural sacs form separate compartments and touch only behind the upper half of the sternal body (see Fig. 53.6A,B), although they are also close to each other behind the oesophagus at the midthoracic level. The region between them is the mediastinum (interpleural space). The left pleural cavity is the smaller of the two, because the heart extends further to the left. The upper and lower limits of the pleurae are about the same on the two sides, but the left sometimes descends lower in the midaxillary line.
The interlobar fissures and posterior azygo-oesophageal and retrosternal pleural reflections are the only aspects of the normal pleura that can be visualized on a chest radiograph or CT scan (Fig. 57.1A–D; see Figs 55.16, 55.17). Demonstration of significant pleural shadowing in any other regions usually implies pathological abnormalities of the pleura. Thoracoscopy allows the direct inspection of both the parietal and visceral surfaces. The parietal pleura is translucent and at thoracoscopy the underlying muscles and blood vessels are visible. The visceral pleura is also translucent and has a grey variegated appearance due to the underlying lung and the vascular network in the subpleural layer.
PARIETAL PLEURA
Costovertebral pleura
Costovertebral pleura lines the sternum, ribs, transversus thoracis and intercostal muscles and the sides of the vertebral bodies; normally it is easily separated from these structures. External to the pleura is a thin layer of loose connective tissue, the endothoracic fascia, which corresponds to the transversalis fascia of the abdominal wall. Anteriorly, the costal pleura begins behind the sternum, where it is continuous with the mediastinal pleura along a junction extending from behind the sternoclavicular joint down and medially to the midline behind the sternal angle. From here, the right and left costal pleurae descend in contact with each other to the level of the fourth costal cartilages and then diverge. On the right side, the line descends to the back of the xiphisternal joint, while on the left the line diverges laterally and descends at a distance of 2–2.5 mm from the sternal margin to the sixth costal cartilage, forming the cardiac notch. On each side, the costal pleura sweeps laterally, lining the internal surfaces of the costal cartilages, ribs, transversus thoracis and intercostal muscles. Posteriorly, it passes over the sympathetic trunk and its branches to reach the sides of the vertebral bodies, where it is again continuous with the mediastinal pleura. The costovertebral pleura is continuous with the cervical pleura at the inner margin of the first rib and below it becomes continuous with the diaphragmatic pleura along a line which differs slightly on the two sides. On the right, this line of costodiaphragmatic reflection begins behind the xiphoid process, passes behind the seventh costal cartilage to reach the eighth rib in the midclavicular line, the tenth rib in the midaxillary line, and then ascends slightly to cross the twelfth rib level with the upper border of the twelfth thoracic spine (see Fig. 53.6A,B). On the left, the line initially follows the ascending part of the sixth costal cartilage, but then follows a course similar to that on the right, although it may be slightly lower.
Cervical pleura
The cervical pleura is a continuation of the costovertebral pleura over the pulmonary apex (Fig. 57.2). It ascends medially from the internal border of the first rib to the apex of the lung, as high as the lower edge of the neck of the first rib, and then descends lateral to the trachea to become the mediastinal pleura. As a result of the obliquity of the first rib, the cervical pleura extends 3–4 cm above the first costal cartilage, but not above the neck of the first rib. The cervical pleura is strengthened by a fascial suprapleural membrane, which is attached in front to the internal border of the first rib, and behind to the anterior border of the transverse process of the seventh cervical vertebra. It contains a few muscular fibres, which spread from the scaleni. Scalenus minimus extends from the anterior border of the transverse process of the seventh cervical vertebra to the inner border of the first rib behind its subclavian groove, and also spreads into the pleural dome, which it therefore tenses: it has been suggested that the suprapleural membrane is the tendon of scalenus medius. The cervical pleura (like the pulmonary apex) reaches the level of the seventh cervical spine approximately 2.5 cm from the midline. Its projection is a curved line from the sternoclavicular joint to the junction of the medial and middle thirds of the clavicle, its summit being 2.5 cm above it. The subclavian artery ascends laterally in a furrow below the summit of the cervical pleura (Fig. 57.2).
Mediastinal pleura
The mediastinal pleura is the lateral boundary of the mediastinum and forms a continuous surface above the hilum of the lung from sternum to vertebral column. On the right it covers the right brachiocephalic vein, the upper part of the superior vena cava, the terminal part of the azygos vein, the right phrenic and vagus nerves, the trachea and oesophagus. On the left it covers the aortic arch, left phrenic and vagus nerves, left brachiocephalic and superior intercostal veins, left common carotid and subclavian arteries, thoracic duct and oesophagus. At the hilum of the lung it turns laterally to form a tube that encloses the hilar structures and is continuous with the pulmonary pleura.
VISCERAL PLEURA
The pulmonary pleura is inseparably adherent to the lung over all its surfaces, including those in the fissures, except at the root or hilum of the lung and along a line descending from this, which marks the attachment of the pulmonary ligament (Figs 57.3, 57.4).
INFERIOR PULMONARY LIGAMENTS
Below the hilum the mediastinal pleura extends as a double layer, the pulmonary ligament, from the lateral surface of the oesophagus to the mediastinal surface of the lung, where it is continuous with the parietal pleura (Figs 57.3, 57.4). It is continuous above with the pleura around the hilar structures and below it ends in a free sickle-shaped border.
PLEURAL RECESSES
The inferior border of the right costodiaphragmatic recess is an important consideration in the surgical posterior approach to the kidney. Usually the pleura crosses the twelfth rib at the lateral border of erector spinae, so that the medial region of the kidney is above the pleural reflection (see Fig. 53.6B). However, if the twelfth rib does not project beyond the muscle, the eleventh rib may be mistaken for the twelfth in palpation, and an incision prolonged to this level will damage the pleura. Whether the lowest palpable rib is the eleventh or twelfth can be ascertained by counting from the second rib (identified at its junction with the sternal angle).
PNEUMOTHORAX
Any breach of the chest wall and parietal pleura or visceral pleura leads to the accumulation of air within the pleural cavity (pneumothorax). Fluid (hydrothorax), blood (haemothorax) and rarely lymph (chylothorax) can also accumulate in this space. Pneumothoraces may occur spontaneously or following trauma (e.g. rib fractures, penetrating injuries from sharp instruments, iatrogenic injury). Significant air in the pleural space is visible on a chest radiograph: there is separation of the parietal and visceral pleurae and an absence of pulmonary vascular markings in the corresponding area. Occasionally, a ball valve-like effect occurs, so that air enters the pleural space during inspiration but cannot escape in expiration. This tension pneumothorax can be life-threatening and should be suspected whenever there are unilateral decreased breath sounds and hyperresonance on percussion, hypotension, jugular venous distension and contralateral tracheal deviation. A tension pneumothorax requires immediate decompression by the insertion of an intercostal drain or wide bore catheter. Fluid collection in the pleural space may be due to congestive cardiac failure, hypoalbuminaemia, inflammatory, infective or neoplastic conditions. A pleural effusion causes obliteration of the costophrenic angle and the diaphragm, and a lateral meniscus is visible on a frontal chest radiograph. Drainage of the fluid and subsequent analysis is required for diagnostic purposes. Where there is a collection of pus (empyema) or blood, pleural drainage is essential for therapeutic purposes. Ultrasonography is useful in assessing the size and characteristics of an effusion, such as the presence of loculation and debris. It may even demonstrate underlying consolidated lung. Computed tomography is utilized to assess the underlying hidden lung parenchyma and mediastinal glands. For further review of the radiology of the pleurae and lungs, see Armstrong (2000).
LUNGS
The lungs are the essential organs of respiration. They are situated on either side of the heart and other mediastinal contents (Figs 57.3, 57.4). Each lung is free in its pleural cavity, except for its attachment to the heart and trachea at the hilum and pulmonary ligament respectively. When removed from the thorax, a fresh lung is spongy, can float in water, and crepitates when handled, because of the air within its alveoli. It is also highly elastic and so it retracts on removal from the thorax. Its surface is smooth and shiny and is separated by fine, dark lines into numerous small polyhedral domains, each crossed by numerous finer lines, indicating the areas of contact between its most peripheral lobules and the pleural surface.
PULMONARY SURFACE FEATURES
Each lung has an apex, base, three borders and two surfaces (Figs 57.3, 57.4). In shape, each lung approximates to half a cone.
Apex
The apex, the rounded upper extremity, protrudes above the thoracic inlet where it contacts the cervical pleura, and is covered in turn by the suprapleural membrane. As a consequence of the obliquity of the inlet, the apex rises 3–4 cm above the level of the first costal cartilage; it is level posteriorly with the neck of the first rib. Its summit is 2.5 cm above the medial third of the clavicle. The apex is therefore in the root of the neck (see Fig. 35.5A). It has been claimed that, because the apex does not rise above the neck of the first rib, it is really intrathoracic, and that it is the anterior surface that ascends highest in inspiration. The subclavian artery arches up and laterally over the suprapleural membrane, grooves the anterior surface of the apex near its summit and separates it from scalenus anterior. The cervicothoracic (stellate) sympathetic ganglion, the ventral ramus of the first thoracic spinal nerve and the superior intercostal artery all lie posterior to the apex. Scalenus medius is lateral, the brachiocephalic artery, right brachiocephalic vein and trachea are adjacent to the right medial surface of the lung, and the left subclavian artery and left brachiocephalic vein are adjacent to the left medial surface of the apex of the lung.
Other impressions on the lung surface
In addition to these pulmonary features, cadaveric lungs that have been preserved in situ can show a number of other impressions that indicate their relations with surrounding structures (Figs 57.3, 57.4). On the right lung the cardiac impression is related to the anterior surface of the right auricle, the anterolateral surface of the right atrium and partially to the anterior surface of the right ventricle. The impression ascends anterior to the hilum as a wide groove for the superior vena cava and the terminal portion of the right brachiocephalic vein. Posteriorly this groove is joined by a deep sulcus which arches forwards above the hilum and is occupied by the azygos vein. The right side of the oesophagus makes a shallow vertical groove behind the hilum and the pulmonary ligament. Towards the diaphragm it inclines left and leaves the right lung, and therefore does not reach the lower limit of this surface. Posteroinferiorly the cardiac impression is confluent with a short wide groove adapted to the inferior vena cava. Between the apex and the groove for the azygos, the trachea and right vagus are close to the lung, but do not mark it.
On the left lung (Fig. 57.4) the cardiac impression is related to the anterior and lateral surfaces of the left ventricle and auricle. The anterior infundibular surface and adjoining part of the right ventricle is also related to the lung as it ascends in front of the hilum to accommodate the pulmonary trunk. A large groove arches over the hilum, and descends behind it and the pulmonary ligament, corresponding to the aortic arch and descending aorta. From its summit a narrower groove ascends to the apex for the left subclavian artery. Behind this, above the aortic groove, the lung is in contact with the thoracic duct and oesophagus. In front of the subclavian groove there is a faint linear depression for the left brachiocephalic vein. Inferiorly, the oesophagus may mould the surface in front of the lower end of the pulmonary ligament.
Pulmonary borders
The inferior border is thin and sharp where it separates the base from the costal surface and extends into the costodiaphragmatic recess, and is more rounded medially where it divides the base from the mediastinal surface. It corresponds, in quiet respiration, to a line drawn from the lowest point of the anterior border which passes to the sixth rib at about the midclavicular line, then to the eighth rib in the midaxillary line (usually 10 cm above the costal margin), and then continues posteriorly, medially and slightly upwards to a point 2 cm lateral to the tenth thoracic spine (see Fig. 53.5A,B). The diaphragm rises higher on the right to accommodate the liver, and so the right lung is vertically shorter (by approximately 2.5 cm) than the left. However, cardiac asymmetry means that the right lung is broader, and has a greater capacity and weight than the left. The posterior border separates the costal surface from the mediastinal surface, and corresponds to the heads of the ribs. It has no recognizable markings and is really a rounded junction of costal and vertebral (medial) surfaces. The thin, sharp, anterior border overlaps the pericardium. On the right it corresponds closely to the costomediastinal line of pleural reflection, and is almost vertical. On the left it approaches the same line above the fourth costal cartilage; below this point it shows a variable cardiac notch, the edge of which passes laterally for 3.5 cm before curving down and medially to the sixth costal cartilage 4 cm from the midline. It therefore does not reach the line of pleural reflection here (see Fig. 53.5A) and so the pericardium is covered only by a double layer of pleura (area of superficial cardiac dullness). However, surgical experience suggests that the line of pleural reflection, the anterior pulmonary margin and the costomediastinal pleural recess are all variable.
PULMONARY FISSURES AND LOBES
Right lung
The right lung is divided into superior, middle and inferior lobes by an oblique and a horizontal fissure (Fig. 57.3). The upper, oblique fissure separates the inferior from the middle and upper lobes, and corresponds closely to the left oblique fissure, although it is less vertical, and crosses the inferior border of the lung approximately 7.5 cm behind its anterior end. On the posterior border it is either level with the spine of the fourth thoracic vertebra or slightly lower. It descends across the fifth intercostal space and follows the sixth rib to the sixth costochondral junction. The short horizontal fissure separates the superior and middle lobes. It passes from the oblique fissure, near the midaxillary line, horizontally forwards to the anterior border of the lung, level with the sternal end of the fourth costal cartilage, then passes backwards to the hilum on the mediastinal surface. The horizontal fissure is usually visible on a frontal chest radiograph. The oblique fissure is usually visible on a lateral radiograph and on a high resolution CT scan as a curvilinear band from the lateral aspect to the hilum (Fig. 57.1A–D). The small middle lobe is cuneiform and includes some of the costal surface, the lower part of the anterior border and the anterior part of the base of the lung. Sometimes the medial part of the upper lobe is partially separated by a fissure of variable depth which contains the terminal part of the azygos vein, enclosed in the free margin of a mesentery derived from the mediastinal pleura, and forming the ‘lobe of the azygos vein’. This varies in size, and sometimes includes the apex of the lung. It is always supplied by one or more branches of the apical bronchus. Radiographically, a pleural effusion may be limited to the azygos fissure. Less common variations are the presence of an inferior accessory fissure, which separates the medial basal segment from the remainder of the lower lobe, and a superior accessory fissure, which separates the apical segment of the lower lobe from the basal segments. The identification of the completeness of the fissures is important prior to lobectomy, because individuals with incomplete fissures are more prone to develop postoperative air leaks, and may require further procedures such as stapling and pericardial sleeves (see Venuta et al 1998).
Left lung
The left lung is divided into a superior and an inferior lobe by an oblique fissure (Fig. 57.4) which extends from the costal to the medial surfaces of the lung both above and below the hilum. Superficially this fissure begins on the medial surface at the posterosuperior part of the hilum. It ascends obliquely backwards to cross the posterior border of the lung 6 cm below the apex, then descends forwards across the costal surface, to reach the lower border almost at its anterior end. It finally ascends on the medial surface to the lower part of the hilum. At the posterior border of the lung the fissure usually lies opposite a surface point 2 cm lateral to the midline between the spines of the third and fourth thoracic vertebrae, but it may be above or below this level. Traced around the chest, the fissure reaches the fifth intercostal space (at or near the midaxillary line) and follows this to intersect the inferior border of the lung close to, or just below, the sixth costochondral junction (7.5 cm from the midline). The left oblique fissure is usually more vertical than the right, and is indicated approximately by the medial border of the scapula when the arm is fully abducted above the shoulder. A left horizontal fissure is a normal variant found occasionally.
Bronchopulmonary segments
Each of the principal bronchi divides into lobar bronchi. Primary branches of the right and left lobar bronchi are termed segmental bronchi because each ramifies in a structurally separate, functionally independent, unit of lung tissue called a bronchopulmonary segment (Figs 57.5–57.7).
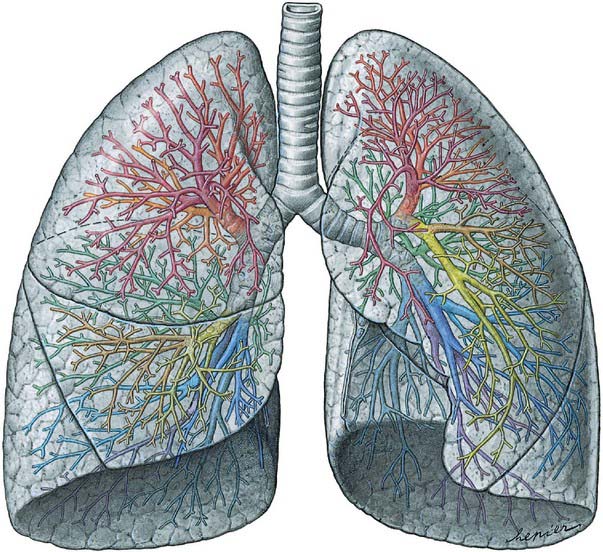
Fig. 57.5 Lungs and bronchi, ventral aspect. The lobar and segmental bronchi are projected onto the lung in different colours (compare with Fig. 57.6).
(From Sobotta 2006.)
Right lung | |
Superior lobe: | I, apical; II, posterior; III, anterior |
Middle lobe: | IV, lateral; V, medial |
Inferior lobe: | VI, superior (apical); VII, medial basal; VIII, anterior basal; IX, lateral basal; X, posterior basal |
Left lung | |
Superior lobe: | I, apical; II, posterior; III, anterior; IV, superior lingular; V, inferior lingular |
Inferior lobe: | VI, superior (apical); VIII, anterior basal; IX, lateral basal; X, posterior basal |
Each segment is surrounded by connective tissue that is continuous with the visceral pleura, and is a separate respiratory unit. The vascular and lymphatic arrangements of the segments are described on pages 996–998.
PULMONARY HILA
The pulmonary root connects the medial surface of the lung to the heart and trachea and is formed by a group of structures which enter or leave the hilum (Figs 57.3, 57.4; see also Fig. 55.4B). These are the principal bronchus, pulmonary artery, two pulmonary veins, bronchial vessels, a pulmonary autonomic plexus, lymph vessels, bronchopulmonary lymph nodes and loose connective tissue, all of which are enveloped by a sleeve of pleura. The pulmonary roots, or pedicles, lie opposite the bodies of the fifth to seventh thoracic vertebrae. The phrenic nerve, pericardiacophrenic artery and vein, and anterior pulmonary plexus are common anterior relations of both hila, and the vagus nerve and posterior pulmonary plexus are common posterior relations. The pulmonary ligament is a common inferior relation. The major structures in both roots are similarly arranged, so that the upper of the two pulmonary veins is anterior, the pulmonary artery and principal bronchus are more posterior, and the bronchial vessels are most posterior. The arrangement of bronchopulmonary segments and the pulmonary hila permit the resection of abscesses and localized lesions caused by lung cancer.
Right hilum
The right root is situated behind the superior vena cava and right atrium and below the terminal part of the azygos vein. The usual sequence of hilar structures from above downwards is: superior lobar bronchus, pulmonary artery, principal bronchus, and lower pulmonary vein (Fig. 57.3).
Left hilum
The left root lies below the aortic arch and in front of the descending thoracic aorta. The usual vertical sequence of structures at the left hilum is pulmonary artery, principal bronchus, and lower pulmonary vein (Fig. 57.4). The pulmonary artery is longer on the left side, and each of its branches from the hilum to the oblique fissure must be identified in pulmonary resections.
VASCULAR SUPPLY AND LYMPHATIC DRAINAGE
Pulmonary arteries
The right pulmonary artery divides into two large branches as it emerges behind the superior vena cava (Fig. 57.8). A lymph node usually occupies the bifurcation. The superior branch, which is the smaller of the two, goes to the superior lobe and usually divides into two further branches, which supply the majority of that lobe. The inferior branch descends anterior to the intermediate bronchus and immediately posterior to the superior pulmonary vein. It provides a small recurrent branch to the superior lobe, then at the point where the horizontal fissure meets the oblique fissure, it gives off the branch to the middle lobe anteriorly, and the branch to the superior segment of the inferior lobe posteriorly. It then continues a short distance before dividing to supply the rest of the inferior lobe segments.
The left pulmonary artery emerges from within the concavity of the aortic arch and descends anterior to the descending aorta to enter the oblique fissure (Fig. 57.8). The branches of the left pulmonary artery are extremely variable. The first and largest branch is usually given off to the anterior segment of the left superior lobe. Before reaching the oblique fissure, the artery gives off a variable number of other branches to the superior lobe, and as it enters the fissure it usually supplies a large branch to the superior segment of the inferior lobe. Lingular branches arise within the fissure, and the rest of the lower lobe is supplied by many varied branching patterns: it was a surgical aphorism of the late Lord Brock that when performing a left upper lobectomy, … ‘there was always one more branch of the pulmonary artery than you thought!’.
Pulmonary veins
In the pulmonary hilum, the usual distribution of veins is as follows. The superior pulmonary vein is anteroinferior to the pulmonary artery, and the inferior is the most inferior hilar structure and also slightly posterior. On the right, the union of apical, anterior and posterior veins (draining the upper lobe) with a middle lobar vein (formed by lateral and medial tributaries; Fig. 57.8) forms the superior right pulmonary vein. The inferior right pulmonary vein is formed by the hilar union of superior (apical) and common basal veins from the lower lobe. The union of superior and inferior basal tributaries forms the common basal vein. Occasionally the three right lobar veins remain separate. The right superior pulmonary vein passes posterior to the superior vena cava, the inferior behind the right atrium. On the left, the superior left pulmonary vein, which drains the upper lobe, is formed by the union of apicoposterior (draining the apical and posterior segments), anterior and lingular veins (Fig. 57.8). The inferior left pulmonary vein, which drains the lower lobe, is formed by the hilar union of two veins, superior (apical) and common basal, the latter formed by the union of a superior and an inferior basal vein. Both superior and inferior left pulmonary veins pass anterior to the descending thoracic aorta. Sometimes the two left pulmonary veins form a single trunk, or they may be augmented by an accessory lobar vein from each lobe, which unite to form a third left pulmonary vein.
The right and left pulmonary veins perforate the fibrous pericardium and open separately in the posterosuperior aspect of the left atrium (see Figs 56.1, 56.2B). Their terminations are separated centrally by the oblique pericardial sinus, and laterally by smaller and variable pulmonary venous pericardial recesses that are directed medially and upwards. The pulmonary veins are devoid of valves.
INNERVATION
The autonomic nervous system controls many aspects of airway function, including regulation of airway smooth muscle tone, mucus secretion from submucosal glands and surface epithelial goblet cells, vascular permeability and blood flow (see Belvisi 2002).
Pulmonary plexuses
The pulmonary plexuses lie anterior and posterior to the other hilar structures of the lungs (see Fig. 56.20). The anterior plexus is small and is formed by rami from vagal and sympathetic cervical cardiac nerves via connections with the superficial cardiac plexus. The posterior pulmonary plexus is formed by the rami of vagal and sympathetic cardiac branches from the second to fifth or sixth thoracic sympathetic ganglia. The left plexus also receives branches from the left recurrent laryngeal nerve. Further details of the pulmonary plexuses are given in the description of the cardiac plexuses in Chapter 56.
MICROSTRUCTURE OF RESPIRATORY SURFACES
Alveolar structure
The alveoli are thin-walled pouches which provide the respiratory surface for gaseous exchange (Figs 57.9–57.11). Their walls contain two types of epithelial cell (pneumocytes) and cover a delicate connective tissue within which a network of capillaries ramifies. Since the walls are extremely thin, they present a minimal barrier to gaseous exchange between the atmosphere and the blood in the capillaries. Adjacent alveoli are frequently in close contact and then the intervening connective tissue forms the central part of an interalveolar septum. Alveolar macrophages are present within the alveolar lumen, and migrate over the epithelial surface.
Interalveolar septum
The alveolar lining epithelium varies in thickness, but extensive areas of it are as little as 0.05 μm thick (see Fig. 57.11). The epithelium lies on a basal lamina, which, in the thin portions of a septum, is fused with the basal lamina surrounding the adjacent capillaries. The total barrier to diffusion between air and blood in these thin portions may be as little as 0.2 μm. The thick portions of a septum contain connective tissue elements, including elastic fibres, collagen type III fibres, resident and migratory cells (Fig. 57.10).
Alveolar epithelial cells (pneumocytes)
The smaller type II cells are often more numerous than type I cells, but they contribute less than 10% of the alveolar surface area. They are rounded cells and protrude from the alveolar surface, particularly at the angles between alveolar profiles. In the human lung they are often associated with interalveolar pores of Kohn. Their cytoplasm contains numerous characteristic secretory lamellar bodies consisting of concentric whorls of phospholipid-rich membrane, the precursors of alveolar surfactant (Fig. 57.11C), which they can recycle.
Alveolar macrophages
Alveolar macrophages can be recovered from sputum, and are of diagnostic importance if they appear abnormal. For instance, whenever erythrocytes leak from pulmonary capillaries, the macrophages that engulf the extravasated cells become brick red, and are detectable in ‘rusty’ sputum. They are typical of congestive heart failure, and often termed heart-failure cells. Macrophages that migrate back into the connective tissue of the lung settle in patches that are visible beneath the visceral pleura, e.g. carbon-filled cells give the lungs a mottled appearance. However, if the inhaled particles are abrasive or chemically active, they may elude macrophage removal and damage the respiratory surface, producing fibrosis and a concomitant reduction in the respiratory area. This occurs in many industrial diseases, e.g. pneumoconiosis, caused by coal dust, or asbestosis, where the long thin fibres of asbestos can cause considerable damage and may trigger fatal mesothelioma in the pleural lining.
Alveolar surfactant
The alveolar surface is normally covered by a film of pulmonary surfactant, which is a complex mixture, mainly of phospholipids (particularly dipalmitoylphosphatidylcholine and phosphatidylglycerol), with some protein and neutral lipid (Devendra & Spragg 2002). Surfactant is stored in lamellar bodies and secreted in the form of tubular myelin (unrelated to myelin of the nervous system) by type II pneumocytes. It is recycled by type II pneumocytes, or cleared (i.e. phagocytosed) by alveolar macrophages. Clara cells of the bronchiolar epithelium are believed to secrete surfactant of a different composition.
TRACHEA AND BRONCHI
The trachea is a tube formed of cartilage and fibromuscular membrane, lined internally by mucosa. The anterolateral portion is made up of incomplete rings of cartilage, and the posterior aspect by a flat muscular wall. It is 10–11 cm long, and descends from the larynx (Fig. 57.7) from the level of the sixth cervical vertebra to the upper border of the fifth thoracic vertebra, where it divides into right and left principal (pulmonary) bronchi. It lies approximately in the sagittal plane, but its point of bifurcation is usually a little to the right. The trachea is mobile and can rapidly alter in length; during deep inspiration, the bifurcation may descend to the level of the sixth thoracic vertebra. Its external transverse diameter is typically 2 cm in adult males, and 1.5 cm in adult females. In children it is smaller, more deeply placed and more mobile. The lumen in live adults has an average transverse diameter of 12 mm, although this increases after death because the smooth muscle making up its posterior aspect relaxes. In the first postnatal year, the tracheal diameter does not exceed 4 mm, while during later childhood its diameter in millimetres is approximately equal to age in years. The transverse shape of the lumen is variable, especially in the later decades of life, and may be round, lunate or flattened. At bronchoscopy the posterior wall of the trachea bulges into the lumen and this is exaggerated in expiration and coughing. The distal end of the trachea is visible as a concave spur. A tracheal bronchus may occasionally arise from the lateral wall of the trachea, more frequently from the right side: it may be supernumerary or it may represent a displaced upper lobe airway.
TRACHEAL RELATIONS
RIGHT MAIN BRONCHUS
The right principal bronchus is approximately 2.5 cm long, and is wider, shorter and more vertical than the left (Figs 57.7, 57.8, 57.12). These differences explain why inhaled foreign bodies enter the right principal bronchus more often than the left (these events are more common in children and they may present with breathlessness, unilateral wheeze or recurrent aspirations: a chest radiograph may show air trapping in the affected lobe). The right main bronchus gives rise to its first branch, the superior lobar bronchus, then enters the right lung opposite the fifth thoracic vertebra. The azygos vein arches over it, and the right pulmonary artery lies at first inferior, then anterior, to it. After giving off the superior lobar bronchus, which arises posterosuperior to the right pulmonary artery, the right main bronchus crosses the posterior aspect of the artery, enters the pulmonary hilum posteroinferior to it, and divides into a middle and an inferior lobar bronchus. Normal variants in the bronchial anatomy are occasionally seen and consist of either displaced or supernumerary airways (see Ghaye et al 2001). Abnormalities include a common origin of the right upper and middle lobe bronchi; an accessory cardiac bronchus; and a right lower lobe bronchus that may arise from the left main stem bronchus. These anatomical variants are largely asymptomatic, but occasionally may cause haemoptysis, recurrent infection and development of bronchiectasis of the airway.
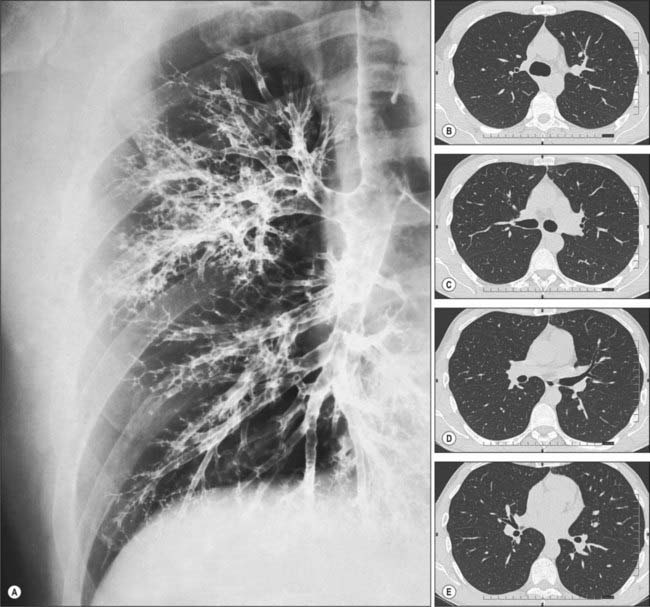
Fig. 57.12 A, Bronchogram showing the branching pattern of the trachea and bronchi of the right lung, in a slightly oblique anteroposterior view. In this procedure, a radiopaque contrast medium has been introduced into the respiratory tract to coat the walls of the respiratory passages. For identification of the major branches (compare with Fig. 57.5. B–E and Fig. 57.6) CT images demonstrating lobar and segmental bronchial branching. (B–E correspond to the 1st, 2nd, 3rd and 4th intercostal spaces, respectively.)
Right superior lobar bronchus
Segmental anatomy
The apical segmental bronchus continues superolaterally towards the apex of the lung, which it supplies, and divides near its origin into apical and anterior branches. The posterior segmental bronchus serves the posteroinferior part of the superior lobe, passes posterolaterally and slightly superiorly, and soon divides into a lateral and a posterior branch. The anterior segmental bronchus runs anteroinferiorly to supply the rest of the superior lobe, and divides near its origin into a lateral and an anterior branch of equal size (Figs 57.5, 57.6).
Right inferior lobar bronchus
Segmental anatomy
At or a little below its origin from the principal bronchus, the right inferior lobar bronchus gives off a large superior (apical) segmental bronchus posteriorly. This runs posteriorly to the upper part of the inferior lobe, and then divides into medial, superior and lateral branches; the first two usually arise from a common stem. After giving off the superior segmental branch, the right inferior lobar bronchus descends posterolaterally. The medial basal segmental bronchus branches from its anteromedial aspect, and runs inferomedially to serve a small region below the hilum. The inferior lobar bronchus continues downwards and then divides into an anterior basal segmental bronchus, which descends anteriorly, and a trunk that soon divides into a lateral basal segmental bronchus, which descends laterally, and a posterior basal segmental bronchus, which descends posteriorly. In more than half of all right lungs a subsuperior (subapical) segmental bronchus arises posteriorly from the right inferior lobar bronchus 1–3 cm below the superior segmental bronchus, and is distributed to the region of lung between the superior and posterior basal segments (Figs 57.5, 57.6).
LEFT MAIN BRONCHUS
Left superior lobar bronchus
Segmental anatomy
The superior division of the left superior lobar bronchus ascends 1 cm, gives off an anterior segmental bronchus, continues a further 1 cm as the apicoposterior segmental bronchus, and then divides into apical and posterior branches. The apical, posterior and anterior segmental bronchi are largely distributed as they are in the right superior lobe. The inferior division descends anterolaterally to the anteroinferior part of the left superior lobe (the lingula) and forms the lingular bronchus, which divides into superior and inferior lingular segmental bronchi. This is different from the pattern in the right middle lobe, where the corresponding distribution is lateral and medial (Figs 57.5, 57.6).
Left inferior lobar bronchus
Segmental anatomy
The superior (apical) segmental bronchus arises from the inferior lobar bronchus posteriorly 1 cm from its origin. After a further 1–2 cm, the inferior lobar bronchus divides into an anteromedial and a posterolateral stem. The latter divides into lateral and posterior basal segmental bronchi. The anterior basal segmental bronchus is an independent branch of the inferior lobar bronchus occasionally. A subsuperior (subapical) segmental bronchus arises posteriorly from the left inferior lobar bronchus sometimes (Figs 57.5, 57.6).
VASCULAR SUPPLY AND LYMPHATIC DRAINAGE
Bronchi
Bronchial arteries
The bronchial arteries supply oxygenated blood to maintain the pulmonary tissues. They are derived from the descending thoracic aorta either directly or indirectly (Baile 1996). The right bronchial artery is usually a branch of the third posterior intercostal artery. There are normally two left bronchial arteries (upper and lower) that branch separately from the thoracic aorta. The bronchial arteries accompany the bronchial tree and supply bronchial glands, the walls of the bronchial tubes and larger pulmonary vessels. The bronchial branches form a capillary plexus in the muscular tunic of the air passages which supports a second, mucosal plexus that communicates with branches of the pulmonary artery and drains into the pulmonary veins. Other arterial branches ramify in interlobular loose connective tissue and most end in either deep or superficial bronchial veins; some also ramify on the surface of the lung, forming subpleural capillary plexuses. Bronchial arteries supply the bronchial wall as far as the respiratory bronchioles, and anastomose with branches of the pulmonary arteries in the walls of the smaller bronchi and in the visceral pleura. These bronchopulmonary anastomoses may be more numerous in the newborn and are subsequently obliterated to a marked degree. In addition to the main bronchial arteries, smaller bronchial branches arise from the descending thoracic aorta: one of these may lie in the pulmonary ligament and may cause bleeding during inferior lobectomy.
Bronchial veins
Usually two on each side, the bronchial veins drain blood from larger bronchi and from hilar structures. They form two distinct systems. Deep bronchial veins commence as intrapulmonary bronchiolar plexuses that communicate freely with the pulmonary veins and eventually join a single trunk that ends in a main pulmonary vein or in the left atrium. Superficial bronchial veins drain extrapulmonary bronchi, visceral pleura and the hilar lymph nodes. They also communicate with the pulmonary veins and end in the azygos vein on the right and in the left superior intercostal or the accessory hemiazygos veins on the left. Bronchial veins do not receive all the blood conveyed by bronchial arteries, some enters the pulmonary veins (see pp. 996–997). The main bronchial arteries and veins run on the dorsal aspect of the extrapulmonary bronchi.
INNERVATION
The anterior and posterior pulmonary plexuses innervate the trachea and the bronchi. The two plexuses are interconnected. The trachea is innervated by branches of the vagi, recurrent laryngeal nerves and sympathetic trunks. The nerves enter the lung as networks that travel along branches of the bronchi and pulmonary and bronchial vessels as far as the visceral pleura. Efferent vagal preganglionic axons synapse on small ganglia within the walls of the tracheobronchial tree: these may act as sites of integration and/or modulation of the input from extrinsic nerves, or permit some local control of aspects of airway function by local reflex mechanisms. The parasympathetic nervous system is the dominant neuronal pathway in the control of airway smooth muscle tone. Stimulation of cholinergic nerves causes bronchoconstriction, mucus secretion and bronchial vasodilation. Sympathetic nerves may control tracheobronchial blood vessels, but innervation of human airway smooth muscle by sympathetic-adrenergic fibres has yet to be convincingly demonstrated.
MICROSTRUCTURE OF THE CONDUCTING AIRWAYS
Epithelium
The epithelia of the trachea, bronchi and bronchioles are, in general, similar to each other, with graded variations in the numbers of different cell types. The extrapulmonary and larger intrapulmonary passages are lined with respiratory epithelium, which is pseudostratified, predominantly ciliated, and contains interspersed mucus-secreting goblet cells. There are fewer cilia in terminal and respiratory bronchioles, and the cells are reduced in height to low columnar or cuboidal. The epithelium of smaller bronchi and bronchioles is folded into conspicuous longitudinal ridges, which allow for changes in luminal diameter (Fig. 57.9). The epithelium in the respiratory bronchioles progressively reduces in height towards the alveoli, and is eventually composed of cuboidal, non-ciliated cells. Respiratory bronchioles have lateral pouches in their walls, which are lined with squamous cells, so providing an accessory respiratory surface.
Connective tissue and muscle
Broad, longitudinal bands of elastin (Fig. 57.13) within the submucosa follow the course of the respiratory tree and connect with the elastin networks of the interalveolar septa (see Fig. 57.9). This elastic framework is a vital mechanical element of the lung, and is responsible for elastic recoil during expiration.
In the trachea and extrapulmonary bronchi, the smooth muscle is mainly confined to the posterior, non-cartilaginous part of the tracheal tube (Fig. 57.14). Along the entire intrapulmonary bronchial tree, smooth muscle forms two opposed helical tracts which become thinner and finally disappear at the level of the alveoli. The tone of these muscle fibres is under nervous and hormonal control: groups of muscle cells are coupled by gap junctions to spread excitation within fascicles.
Cartilaginous support
The trachea and extrapulmonary bronchi contain a framework of incomplete rings of hyaline cartilage which are united by fibrous tissue and smooth muscle (Fig. 57.14). Intrapulmonary bronchi contain discontinuous plates or islands of hyaline cartilage in their walls.
Tracheal cartilages
The first and last tracheal cartilages differ from the rest (Fig. 57.7). The first cartilage is the broadest. It often bifurcates at one end and is connected by the cricotracheal ligament to the inferior border of the cricoid cartilage, with which it may blend. It may also blend with the second cartilage. The last cartilage is centrally thick and broad and its lower border, the carina, is a triangular hook-shaped process which curves down and backwards between the bronchi (Fig. 57.9). It forms an incomplete ring on each side, and encloses the start of a principal bronchus.
PULMONARY DEFENSIVE MECHANISMS
Inhaled particles may be removed via the mucociliary rejection current. Cilia sweep the fluid overlying the surfaces of bronchioles, bronchi and trachea upwards at 1 cm/min, and much of the inhaled matter trapped in the viscous fluid may be removed in this way. Particles small enough to reach the alveoli may be removed by alveolar phagocytes (see p. 998). Alveolar epithelium has limited powers of regeneration but normally is continually replaced. The lifespan of alveolar squamous cells is approximately 3 weeks, and that of alveolar phagocytes is 4 days. Inhaled particles may also be cleared by the cough reflex.
Anatomy of coughing
Coughing is initiated when mechanically and chemically sensitive vagal afferents innervating the airways are activated. Data from experimental studies suggest that the receptors innervated by these afferents may be broadly divided into three groups: slowly (SAR) and rapidly (RAR) adapting stretch receptors and bronchopulmonary C fibres. The vagal afferents terminate centrally in largely nonoverlapping regions of the caudal half of the nucleus of the solitary tract; second order neurones from this nucleus terminate in respiratory-related regions of the medulla, pons and spinal cord. Afferent nerves innervating other viscera, and somatosensory nerves innervating the chest wall, diaphragm and anterior abdominal musculature are also likely be involved in regulating cough.
The identity of the afferent terminals in the airways is uncertain; receptors similar to taste buds are considered to be responsible at the laryngeal aditus, and some of the ‘brush cells’ of the respiratory epithelium appear to have neural contacts, and may therefore represent sensory cells with basal synaptic outputs (Widdicombe 2002). Other than the larynx, the carina and branching points of the tracheobronchial tree appear to be the most sensitive areas of the epithelium lining the airways.
For further details, see Kubin et al (2006) and Canning (2006).
BRONCHOSCOPY
Bronchoscopy allows the direct visualization of the vocal cords, trachea and major airways as far as the first division of the subsegmental airway. Occasionally bronchoscopy can also provide some information about structures adjacent to the airways, for example, significant subcarinal lymphadenopathy may cause splaying or widening of the carina. Bronchoscopy enables the acquisition of samples (e.g. bronchial lavage, bronchial brushings, bronchial and transbronchial biopsies), provides information about staging in lung cancer, and facilitates therapeutic procedures (e.g. foreign body removal, ablation of tumours and insertion of airway stents). The information obtained by continuous (spiral) volume CT scanning can be reconstructed to provide three-dimensional images which can be used to create endobronchial views (virtual bronchoscopy, Fig 57.15) or to demonstrate the pulmonary arteries.
Armstrong P. The normal chest. In: Armstrong P, Wilson AG, Dee P, Hansell DM. Images of the Diseases of the Chest. London: Mosby; 2000:21-62.
Baile EM. The anatomy and physiology of the bronchial circulation. J Aerosol Med. 1996;9:1-6.
Belvisi MG. Overview of the innervation of the lung. Curr Opin Pharmacol. 2002;2:211-215.
Canning BJ. Anatomy and neurophysiology of the cough reflex: ACCP evidence-based clinical practice guidelines. Chest. 2006;129:33S-47S.
Devendra G, Spragg RG. Lung surfactant in subacute pulmonary disease. Respir Res. 2002;3:19-22.
Ghaye B, Szapiro D, Fanchamps JM, Dondelinger RF. Congenital bronchial abnormalities revisited. Radiographics. 2001;21:105-119.
Jeffery PK. Microscopic structure of the lung. In: Gibson GJ, Geddes DM, Costabel U, Sterk PJ, Corrin B. Respiratory Medicine. 3rd edn. London: Elsevier Science; 2003:34-50.
Kubin L, Alheid GF, Zuperku EJ, McCrimmon DR. Central pathways of pulmonary and lower airway vagal afferents. J Appl Physiol. 2006;101:618-627.
Venuta F, Rendina EA, De Giacoma T, et al. Technique to reduce air leaks after pulmonary lobectomy. Eur J Cardiothorac Surg. 1998;13:361-364.
Widdicombe J. Neuroregulation of cough: implications for drug therapy. Curr Opin Pharmacol. 2002;2:256-263.