Chapter 15
Physiology of Sleep-Disordered Breathing
After reading this chapter, you will be able to:
• Explain how the brain regulates sleeping and waking cycles through neurocontrolled chemical mediators
• Explain why the upper airway anatomy plays such a major role in sleep-disordered breathing (SDB)
• Distinguish between the three stages of nonrapid eye movement sleep and rapid eye movement sleep
• Identify the six major categories of sleep disorders and the specific classification for SDB
• Differentiate between obstructive sleep apnea, central sleep apnea, mixed sleep apnea, childhood sleep apnea, and sudden infant death syndrome
• Explain the effects of SDB on the cardiovascular system
• Identify the mechanisms of central hemodynamic dysfunction secondary to SDB
• Classify SDB through the use of the polysomnogram
• Explain the physiological treatment of SDB using positive airway pressure, oral appliances, positional therapy, and airway enlargement treatment
bilevel positive airway pressure (BIPAP)
continuous positive airway pressure (CPAP)
durable medical equipment (DME)
mandibular advancement device (MAD)
radiofrequency tissue ablation
respiratory effort–related arousals (RERAs)
sleep-disordered breathing (SDB)
sudden infant death syndrome (SIDS)
Functional Anatomy and Physiology of Sleep
“To sleep: perchance to dream: ay, there’s the rub.”—William Shakespeare, Hamlet (III, I, 65-68)
Neurocontrol of Sleep
Sleep has been described as “a reversible behavioral state of perceptual unresponsiveness to the environment with documentable, distinguishable physiological patterns of neural and muscle activity.”1 The diagnostic test performed to evaluate the quality of sleep is called a sleep study, or polysomnogram (PSG). The PSG documents more than 20 individual physiological parameters during all phases of the sleep cycle. The term polysomnogram is derived from its prefix, root, and suffix definitions: “poly” means “many,” “somno” means “sleep,” and “gram” means “writing.” This quantitative diagnostic study records physiological data during each second of sleep from wakefulness through the three sleep stages of nonrapid eye movement (NREM) to the rapid eye movement (REM) stage. Through the PSG, the sleep process can be classified in an objective, quantifiable way. However, the precise mechanisms responsible for sleep onset and waking from sleep continue to be the focus of intensive investigation. Although the brain plays a definitive role in sleep regulation, the specific anatomical region of the brain responsible for the sleep control is a subject of controversy. It is now well established that the hypothalamus is the key regulator of sleep and wakefulness.2 Since sleep research is now focused on the hypothalamus, new pharmaceutical and therapeutic breakthroughs for neurological sleep disorders have been developed.
The brain is composed of five major regions: the medulla oblongata, which is the most posterior portion of the brain that fuses with the spinal cord; the pons, located superiorly to the medulla oblongata; the midbrain or mesencephalon, located just above the pons; the telencephalon or cerebrum, which consists of five paired lobes within two convoluted hemispheres; and the diencephalon, which sits superior to the brainstem nestled between the two cerebral hemispheres. The diencephalon includes the thalamus, the hypothalamus, the optic chiasm, and the pineal gland (Figure 15-1). Sleep researchers have identified more than 70 distinct sleep disorders but have yet to identify the precise neurochemical mechanisms that “turn on and turn off” sleep and wakefulness. As mentioned, the central focus of study in sleep regulation is currently the hypothalamus.
Biomedical sleep research has definitively determined that the primary biological “sleep clock” resides in the suprachiasmatic nucleus (SCN) of the hypothalamus where it controls the timing of the sleep cycle, or the circadian rhythm. When SCN function is disrupted, the circadian rhythm becomes random and sporadic. However, even though the onset of sleep may vary throughout a 24-hour period, the total sleep time remains the same. Located just above the optic chiasm, the SCN is believed to control certain neurologically secreted chemicals that regulate the sleep cycle. Light plays a very important role in the normal regulation of the SCN. Light is an environmental cue or stimulator known as the zeitgeber, or “time-giver,” that regulates the sleep/wake cycle. The role that light plays as a circadian rhythm “cue” can be observed through the disrupted sleep habits of individuals living in geographical areas of prolonged winter darkness. A decrease in the amount of daylight exposure significantly alters the normal sleep/wake cycle during the winter seasons. The optic chiasm is thought to relay light/dark cues from the eye to the hypothalamus as a reference for the circadian rhythm. It is now understood that the SCN of the hypothalamus merely tracks day and night cycles, whereas the ventrolateral preoptic nucleus (VLPO) in the hypothalamus initiates sleep by inhibiting the brain’s arousal centers in the brainstem (Figure 15-2).
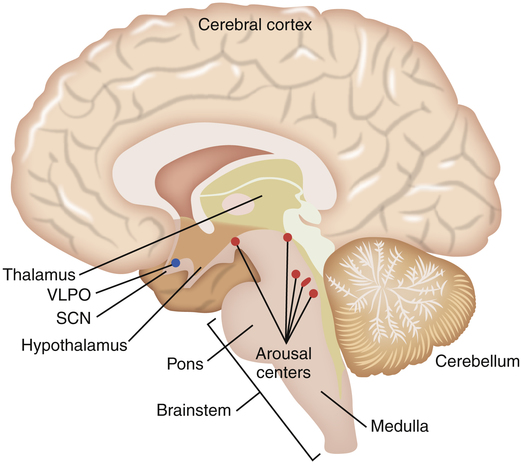
The role of the hypothalamus in controlling the regulation of sleep/wake mechanisms has been confirmed. Various neurotransmitters released by the hypothalamus are believed to produce sleep by inhibiting the brain’s arousal centers. The neurotransmitters that mediate arousal in the cortex include histamine, serotonin, norepinephrine, acetylcholine, and hypocretin (orexin) (Figure 15-3). Sleep research has shown that disruption of the VLPO and the lateral region of the hypothalamus results in arousal. The loss of the VLPO and the lateral hypothalamus structures have been associated with the symptoms of insomnia or narcolepsy respectively.3
Upper Airway Anatomy and Sleep
adulthood, humans remain obligate nose breathers during sleep, which is often the source of significant problems for individuals with sleep disorders.
Oropharynx
The upper airways comprise three primary anatomical regions: the nose, pharynx, and larynx. As discussed in Chapter 1, normal pharyngeal muscle tone prevents the base of the tongue from falling backward in the oral cavity and obstructing the oropharyngeal airway. Sleep or unconscious states result in a loss of pharyngeal muscle tone and the relaxation of the soft tissue that constitutes the oropharynx and the tongue. Under the influence of gravity, the relaxed tongue falls into the oropharynx, partially or totally occluding the airway. Partial occlusions result in low-pitched snoring sounds, whereas complete occlusion results in apnea, characterized by ventilatory efforts without airflow. Several other soft tissue anatomical structures in the oropharynx may contribute to partial or total obstruction.
Within the oral cavity, five specific structures can obstruct airflow. Mallampati was the first to categorize the amount of “open space” in the oropharynx by the visualization of five structures: the tongue, the soft palate, the hard palate, the uvula, and the tonsils.4 Originally used to classify the difficulty level of oral endotracheal intubation, the Mallampati score is determined by direct visualization of the oropharynx through the open mouth. There are four categories of decreasing airway space (Figure 15-4). A class 1 score is considered normal, in which all five anatomical structures are visible. In class 2, all five structures can be identified, but only the upper portions of the tonsils and uvula are visible. Class 3 allows only the tongue, the soft and hard plate, and the base of the uvula to be seen. Class 4 allows visualization of only the hard palate and tongue. The higher the Mallampati classification number, the more anatomical crowding with less oropharyngeal room for airflow. Sleep research has shown a positive correlation between a high Mallampati score and the risk for obstructive sleep disorders when nasal obstruction is present.5
Because the mandible anatomically supports and positions the tongue and is part of the oropharyngeal structure, the size of the mandible may limit airflow during sleep. A small, recessed lower jaw, or retrognathia, results in a more retrograde or posteriorly positioned tongue. A posterior position of the tongue makes it easier for the tongue to fall back and block the oropharynx as the pharyngeal muscles relax. An enlarged tongue, or macroglossia, as seen in individuals with Down syndrome, hypothyroidism, and acromegaly, can also crowd the oropharynx decreasing the size of the airway lumen. A shortened or widened neck may also crowd the anatomical structures, narrowing or obstructing the oropharyngeal lumen. A neck circumference greater than 17 inches in men or greater than 16 inches in women is highly correlated with the incidence of obstructive sleep apnea (OSA).6,7
Stages of Sleep
The normal pattern of sleep stages involves the cycling back and forth between NREM and REM stages every 60 to 90 minutes for four to five cycles during an 8-hour sleep period. Sleep normally begins with the NREM stages and progresses to REM sleep. During the awake phase, the electroencephalogram (EEG), or electrical waveform of brain activity, shows a pattern of small, fine waves oscillating at a high frequency, known as alpha waves (Figure 15-5). At the onset of sleep, alpha waveforms slow and change to waveforms characteristic of NREM sleep, suggesting a resting or restorative state of the brain.
Nonrapid Eye Movement
NREM sleep is composed of three stages with each progressive stage transitioning to a deeper state of sleep. Stage 1 NREM represents the onset of sleep for adults and children. During stage 1 NREM, the eyes roll gently and slowly while low-amplitude EEG brainwaves are noted during the sleep study. This change in EEG brain wave pattern is associated with the transition from awake to sleep. Only 5% to 10% of the entire sleep period is spent in stage 1 NREM, and within 2 to 10 minutes, sleep usually progresses to stage 2 NREM. EEG tracings show sharp spikes called “K-complexes” and “sleep spindles,” which serve as markers for the transition from stage 1 NREM to stage 2 NREM. In adults, approximately 40% to 50% of the total sleep period is spent in stage 2 NREM.8
Stage 3 NREM is the deepest stage of sleep and represents approximately 25% of the sleep period. The EEG displays delta waves, or slow-wave sleep, characterized by high-amplitude waves. “Delta sleep” is thought to represent restorative sleep, during which the brain is in its deepest state of sleep. Stage 3 NREM is characterized by a very low level of patient responsiveness; it is difficult to awaken a person from this stage of sleep. Essential growth hormones are released during this stage.8–10
During all three stages of NREM sleep, the muscles of the body exhibit tone and movement; individuals often turn in their sleep and reposition themselves in the bed. Although control of core body temperature and regulation of respiration is maintained during NREM, the respiratory rate slows and tidal volume decreases, causing an increase of 2 to 4 mm Hg in PaCO2. Minute ventilation is approximately 13% to 15% lower in NREM sleep than during wakefulness. Systemic blood pressure may decrease by 5% to 10% during NREM stages 1 and 2 and decrease 8% to 14% during NREM stage 3 sleep. With advancing age, total time spent in stages 1 and 2 progressively increases, whereas stage 3 sleep decreases significantly.10,11
Rapid Eye Movement
EEG tracings during REM strongly resemble the level of brain activity seen in the awake state (i.e. alpha-like, low-voltage, random, high-frequency waveforms) (see Figure 15-5). As sleep progresses, adults and children cycle back to NREM stages and return to the REM stage three to five times. The time spent in REM normally increases with each cycle for a total of approximately 25% of the sleep period. Electromyography (EMG) shows skeletal muscle tone at its lowest level during the REM stage, suggesting a paralyzed state. This partial paralysis results in a further decrease of the minute ventilation in healthy adults and children, producing a few associated episodes of hypoxemia and hypercapnia, which are normal during REM. Loss of skeletal muscle tone during REM affects pharyngeal muscles; upper airway resistance increases as pharyngeal tissues relax and narrow the upper airway lumen. As previously mentioned, relaxation of the tongue and soft tissues of the oropharynx are the primary cause of increased upper airway resistance, possibly leading to upper airway obstruction. Additionally, REM sleep is associated with heart rate variability and cardiac arrhythmias.8–11
Sleep architecture refers to the pattern in which an individual moves back and forth between sleep stages throughout the night. Although the approximate percentage of sleep time spent in the three stages of NREM and the REM stage previously described represents normal sleep architecture, each person has a distinct pattern unique to his or her own sleep cycle. The graphic representation of a patient’s sleep architecture is known as a sleep histogram and is a standard part of every sleep study; it depicts the summative time spent in each phase of sleep at a glance (Figure 15-6). When a patient’s NREM and REM cycles become fragmented on a regular basis, the patient is said to have poor sleep hygiene. Poor sleep hygiene is often due to the lack of a sleep routine or the lack of appropriate sleep cues that signal the body to prepare for sleep, which is typical of the sleep habits of a night shift worker.9–11
Sleep Disorders
Approximately 1 in 6, or greater than 50 million, Americans have some form of sleep disorder. More than 84 sleep disorders have been identified and coded by the World Health Organization’s International Classification of Diseases and Related Health Problems, 10th Revision (ICD-10), which assists health care providers in categorizing sleep disturbances. The ICD-10 coding system classifies diseases, signs and symptoms, abnormal findings, complaints, social circumstances, and external causes of injury or diseases.12 In the latest edition of ICD-10, sleep disorders are classified under “Mental and Behavior Disorders” in a subcategory called “Behavior Syndromes Associated with Physiological Disturbances and Physical Factors.” The physiology and comorbidities associated with sleep disturbances are complex; a growing number of patients with SDB complain of numerous chronic ailments, including hypertension, depression, heart failure, obesity, learning disorders, and posttraumatic stress syndrome.
There are six broad classifications of sleep disorders with multiple subcategories (Box 15-1). The six major categories include dyssomnias, parasomnias, medical or psychiatric conditions producing sleep disorders, sleep sickness, snoring, and sudden infant death syndrome (SIDS). The largest and most common category of sleep disorders is the dyssomnia group. Dyssomnias (“difficult sleep”) are primarily characterized either as excessive daytime sleepiness (hypersomnolence) or as the inability to fall asleep (insomnia). SDBs in the dyssomnia category include obstructive sleep apnea (OSA), central sleep apnea (CSA), and mixed sleep apnea (MSA)
Obstructive Sleep Apnea
of suffocation (Figure 15-7). The increase in PaCO2 prompts arousal of the medullary chemoreceptors, which is documented by the EEG; the patient often gasps, is aroused from sleep, and sits up in bed to open the airway. Often the patient does not completely awaken; however, deep REM sleep is disrupted, bringing the patient into stage 1 or 2 NREM sleep. Most patients are unaware that they sit up in bed, gasp, or cough; they simply lay down again, only to repeat the process throughout the night. As soon as the individual returns to REM sleep, the next obstruction begins. Because of anatomy and gravity, the most vulnerable sleeping position for the patient with OSA is supine. Turning to either side or the prone position may significantly decrease the degree of airway obstruction at REM onset.
If airway obstruction is partial, a hypopnea may occur, and the process just described is repeated except that ventilations are shallow rather than absent. Hypopneas frequently cause EEG arousals, and the patient may reposition in the bed before resuming sleep (Figure 15-8). Such arousals, as depicted on the PSG, are known as respiratory effort–related arousals (RERAs) (Figure 15-9). During RERAs, the thorax and abdomen move in a diminished, synchronous manner, although airflow does not appear to be reduced, and the SpO2 remains stable. However, RERAs usually result in an EEG arousal, and sleep architecture may be disrupted.
OSA occurs most frequently in elderly obese men with a short neck greater than 17 inches in circumference. Heavy snoring that begins soon after falling asleep is the classic picture of a patient with OSA. Typically, the snoring becomes louder and is interrupted by a period of apnea followed by a loud snort or gasp. After reestablishing the airway, snoring returns, and the cycle repeats. The snoring and gasping events may drive the sleep partner from the bed to another room. Risk factors associated with worsening of OSA include a body mass index 25 or greater, alcohol consumption before bedtime, smoking, nasal congestion at night, and large tonsils. The consequences of uncorrected OSA include decreased physical performance, decreased mental performance owing to a reduced ability to concentrate, psychosocial problems, impotence, decreased libido, hypertension, exacerbation of coronary artery disease, myocardial infarction, heart failure, and stroke.9,10
Central Sleep Apnea
The second frequently encountered classification of SDB is central sleep apnea (CSA). CSA is characterized by lack of airflow accompanied by the total absence of thoracoabdominal effort for at least 10 seconds. The cause of apnea is not obstruction of the airway but a lack of normal brain activity associated with the physiological drive to breathe. CSA is responsible for only about 10% of all adult SDB but is more common in children and infants. CSA is observed most often during stage 1 NREM or REM sleep. The cause of CSA is varied and complex. It is thought that CSA may be linked to a loss of the neurological control of breathing secondary to injury, stroke, brainstem lesion, encephalitis, neurodegeneration, radiation treatments to the cervical spine region, or congestive heart failure.10,11,13
Individuals with CSA are most often not obese and have a normal neck circumference. Snoring is not usually present; however, excessive daytime somnolence, or sleepiness, may occur because of frequent awakenings and lack of restorative sleep. Because CSA is closely associated with neurological issues, patients may report symptoms such as difficulty swallowing, a change in the ability to use their voices, or other body weakness or numbness. Any of these neurological symptoms should be immediately brought to the attention of a physician for complete assessment and diagnostic investigation. The most common sign of CSA is the presence of Cheyne-Stokes respirations (see Chapter 11).9 Figure 15-10 shows a PSG study of a patient with CSA.
Children with Central Sleep Apnea
Although CSA is much less common in children than adults, it is often found in premature infants born before 37 weeks of gestation. CSA is associated with congenital cardiac disorders that cause an elevation of the PaCO2. As with adults, hypercapnic drive centers in the brainstem may be insensitive or too immature to trigger corrective breathing stimuli to respiratory muscles. Multiple disorders may precipitate CSA in children and infants, including neuromuscular, neurological, metabolic, gastrointestinal, and hematological abnormalities and infections with an accompanying fever.8,11
Sudden infant death syndrome (SIDS) is thought by some experts to be associated with CSA, but there is no clinical evidence that the two are linked. The Centers for Disease Control and Prevention defines SIDS as “the sudden death of an infant less than one year of age that cannot be explained after a thorough investigation is conducted, including a complete autopsy, examination of the death scene and review of the clinical history.”14 Although the etiology is unknown, most reported SIDS cases occur in the first 6 months of life with a peak incidence between 2 and 4 months of age. Previously thought to be linked to CSA pathophysiology, SIDS is now believed to have a familial relationship to OSA and positional sleeping and to be associated with families that have a history of apparent life-threatening events.14 Research during the last decade has linked SIDS to risk factors such as the prone sleeping position, bed sharing, maternal substance abuse, and maternal cigarette smoking. Despite a 38% decrease in SIDS incidence in the United States, it remains the leading cause of death in children younger than 1 year.
Mixed Sleep Apnea
As the name implies, mixed sleep apnea (MSA) is a combination of OSA and CSA in the same patient. A PSG study of the patient with MSA typically reveals an initial CSA event followed by an OSA phase (Figure 15-11). During the CSA portion of the MSA sequence, there is no thoracic wall or abdominal effort associated with loss of airflow and declining SpO2. Following the CSA event, thoracic and abdominal efforts return, but the effort is met with an upper airway obstruction for the remainder of the MSA sequence. As is often the case, support of the collapsing airway through the use of CPAP may eliminate the OSA aspect of the sequence only to allow the CSA component and continued apnea to persist. Many times, as positive airway pressure (PAP) is titrated in the patient with OSA, an underlying CSA episode is discovered when the OSA issue is resolved. Without the PSG diagnostic/titration study, MSA is virtually impossible to confirm. When the OSA component is treated with PAP, the CSA component can be addressed by providing a backup respiratory rate controller on the PAP device. The use of rate controller PAP devices quickly resolves any CSA issues, and the patient can sleep without apneic episodes.10,11
Cardiovascular Effects of Untreated Sleep-Disordered Breathing
SDB is a significant risk factor in the development of hypertension and multiple cardiovascular conditions. Effective therapy can eliminate or reverse the detrimental cardiovascular effects of SDB; nevertheless, cardiovascular disorders are increased in these patients either because SDB is undiagnosed or because patients fail to comply with prescribed PAP therapy. The simple act of “breathing while asleep” is key to the restoration of cardiovascular health and a decreased rate of morbidity and morality.15
Central Hemodynamic Factors
Regardless of the cause of SDB, apneas and hypopneas cause arterial oxygen desaturation, hypercapnia, fragmented sleep, and sleep arousals. Hypoxia and hypercapnia have a powerful effect on the cardiovascular system. As discussed in Chapter 6, hypoxia and hypercapnia cause pulmonary vasoconstriction. Pulmonary venous constriction impedes blood flow to the left atrium and ventricle and coupled with pulmonary arteriolar constriction increases right atrial and ventricular pressures. In SDB, variation in respiratory rate interspersed with apneic periods causes PaCO2 and PaO2 to vacillate between normal and abnormal values, which produce a physiological “seesaw” dynamic in ventricular pressures. As a result, systemic blood pressure decreases and rebounds with a parallel decrease and increase in heart rate. Cardiac pressures can increase and then decrease to the point that blood flow momentarily stops; the resulting blood stagnation and stasis clotting may lead to potential stroke or myocardial infarction.15,16
During periods of airway obstruction in OSA and MSA, thoracic inspiratory expansion creates large negative intrathoracic pressures, which decrease left ventricular stroke volume and cause a momentary decrease in systemic blood pressure. Blood flow through the already constricted pulmonary vasculature is decreased further, exacerbating cardiac function, as manifested by diminished cardiac output, stroke volume, and systemic blood pressure. When airway obstruction is resolved through sleep arousal or a change in position, stroke volume, heart rate, and blood pressure transiently increase. This physiological “seesaw” of hemodynamics in patients with SDB occurs during each cycle of apnea. Because episodes of apnea commonly result in some level of arousal, sleep is further fragmented as the patient swings between REM and NREM or is awakened by the event.17,18
Causal Role in Cardiovascular Disease
Studies have clearly documented the relationship between long-standing SDB and the predisposition for cardiovascular disease; sympathetic nervous system overactivity is thought to be a critical mechanism in the pathogenesis of hypertension. Repetitive airway occlusion, hypercapnia, and hypoxia, accompanied by vacillations in intrathoracic pressures elicit autonomic, neural, and humeral responses. A night of fragmented sleep may adversely affect cardiovascular function during waking hours the next day, although daytime breathing is normal.18 Nocturnal hypoxemia results in endothelial cell dysfunction, producing vasoconstriction and elevated blood pressure during both sleeping and waking hours. Urinary catecholamine levels, an indicator of sympathetic stress and overstimulation, are elevated in patients with untreated SDB, manifesting the persistence of OSA-induced physiological stress throughout the day. Studies have also shown that when patients with OSA are treated with PAP, and sleep apnea is resolved, urinary catecholamine levels return to normal. Hypertension is frequently resolved or diminished through PAP treatment of OSA.19
Patients with untreated OSA have a higher risk of hypercoagulability (i.e., increased tendency to develop intravascular blood clots) and subsequent thrombosis than their PAP-treated counterparts. Hypercoagulability mechanisms are still under investigation, but current research suggests changes in clotting factors might be due to the increased number of neutrophils seen in patients with SDB, along with increased catecholamine levels and compromised blood flow.17 Hypercoagulability increases the risk of coronary artery disease when it coexists with other conditions present in patients with OSA, including obesity, metabolic syndrome, insulin resistance, diabetes, and hyperlipidemia. Fluctuations in blood flow caused by repeated blood pressure surges in OSA result in additional myocardial work, which is associated with left ventricular hypertrophy, often a precursor of adverse cardiovascular events.
Heart failure, or systolic dysfunction (ejection fraction <40%), is asymptomatic in about 2% of the general population.18 Additionally, diastolic dysfunction (failure of the ventricle to fill properly) is a major risk factor in the development of congestive heart failure, or left ventricular failure. Patients with OSA and CSA commonly have coexisting heart failure and its associated increase in mortality rate. Decreased blood flow leads to myocardial ischemia during periods of negative intrathoracic pressure, which may lead to an increased left ventricular afterload, a decreased left ventricular preload, and a subsequent reduction in stroke volume. The use of PAP produces immediate cardiovascular improvement by relieving hypoxia and hypercapnia; the associated improvement in myocardial oxygenation decreases left ventricular end-systolic volume and improves ejection fraction.19
SDB and cardiac arrhythmias are also significantly linked, although the association is not well understood. Atrial and ventricular premature ectopic beats are the most common arrhythmias in SDB. Separating cardiac arrhythmias that result from preexisting heart disease from the effects of SDB is difficult; however, successful treatment of SDB seems to decrease arrhythmias.20 The role of SDB as an independent risk factor for cardiac arrhythmias is yet to be substantiated in the scientific literature.
Physiological Diagnosis of Sleep-Disordered Breathing
Polysomnogram
The treatment of SBD begins with a definitive diagnosis based on a PSG sleep study, which is the “gold standard” diagnostic tool in determining the presence of a sleep disorder. The guidelines for conducting and evaluating sleep studies are established by the Association of Sleep Disorders Center and further defined by Medicare Practice Guidelines. The configuration of the various physiological tracings on the PSG display is called a montage (Figure 15-12). The montage for a typical sleep study includes a minimum of 17 channels of physiological tracings. Electrodes are strategically placed on the patient’s head, face, chest, abdomen, and legs to collect the physiological data (Figure 15-13). All data are recorded continuously from “lights off” to “lights on” including four EEG channels to identify NREM or REM sleep stages, two channels recording right and left eye movement, one channel monitoring chin movement, two channels recording right and left leg movement, and nine more channels documenting snoring, nasal and oral airflow, thoracic and abdominal movement, SpO2, heart rate, electrocardiogram (ECG), and body position.8
Sleep clinicians must adhere to strict criteria to conduct the PSG study properly and titrate the appropriate pressure needed to resolve apneas and hypopneas. Apneas and hypopneas must be counted during each hour of the sleep study and serve as the basis for certain calculations. The primary index defining the presence of apneas or hypopneas or both is the apnea-hypopnea index (AHI), or the number of hypopneas and apneas per hour of sleep time. For adults, an AHI greater than 5 per hour with a 4% decrease in SpO2 from baseline is considered abnormal and requires treatment. For infants and children, an AHI greater than 1 is considered abnormal when end-tidal PCO2 is greater than 53 mm Hg or SpO2 is less than 92%.8–10 Whether SDB is anatomical or neurological, the AHI is the primary criterion on which the diagnosis of SDB is based. Ideally, the prescriptive pressure derived from a PSG study is the PAP in cm H2O pressure required to keep the patient’s airway patent. The goal in titrating PAP is to reduce AHI to 5 or less per hour while the patient is in the supine position. When PAP is correctly adjusted, the patient’s apnea and hypopnea are immediately resolved. If the correct prescriptive PAP is applied, the patient is able to sleep through the night without sleep fragmentation.10
than 40 is “severe” sleep apnea in an adult. Other criteria and symptoms must also be considered in the evaluation of a sleep study. Patients with SDB and preexisting pulmonary or cardiac disease present a complicated clinical problem that requires additional assessment for treatment options.11
PAP devices, such as continuous positive airway pressure (CPAP) and bilevel positive airway pressure (BIPAP) machines, provide the anatomical “cure” for the presence of OSA, CSA, or MSA. The “titration night” in the sleep laboratory is critically important to provide the correct mask fit for the patient and to find the correct PAP required to resolve the obstruction. For CSA, BIPAP with a guaranteed back-up respiratory rate ensures that the patient remains appropriately ventilated during periods of CSA. Some physicians may prefer that the diagnostic and titration procedures be completed in one night, which is called a split-night sleep study. However, split-night studies are not recommended for optimal diagnosis and treatment of SDB because of time limitations. Many patients have a difficult time falling asleep soon enough to allow the sleep clinician to quantify the sleep disturbance and implement intervention protocols. Titration of the correct PAP and selection of a properly fitting mask can be difficult and time-consuming. The patient must fall asleep before a supine REM state can be documented with the level of PAP required to resolve the airway obstruction.9,10
Anatomical Treatment of Sleep-Disordered Breathing
Positive Airway Pressure
SDB caused by soft tissue obstruction of the oropharynx can be immediately resolved without surgery or pharmaceuticals through the use of properly administered PAP therapy. Judicious titration of PAP is required to identify the precise pressure that alleviates sleep apnea, snoring, and hypopneas. Each titration sleep study is individually tailored to meet the specific needs of the patient, which are related to the degree of obstruction present. PAP treatment begins with a properly sized and fitted mask. With more than 50 brands and types of masks available, the sleep clinician must be an expert in finding the properly designed mask that accommodates each patient’s particular facial features and anatomical structures. The clinician chooses a nasal or oronasal mask after assessing the patient’s facial anatomy. If the patient requires high PAPs, nasal masks are not suitable, and an oronasal or full facial mask is needed to provide the appropriate pressure without leaking. Additionally, patient comfort is a critical factor affecting continued patient use or compliance with PAP therapy. Overtightening the mask can cause leaks or patient discomfort and is usually a sign of improper sizing. Without the proper mask fit, applied pressures do not reliably keep the airways patent (Figure 15-14).21
More than 50 models of PAP, CPAP, and BIPAP generators exist, and it is beyond the scope of this chapter to detail their differences. Various PAP modes and delivery systems are available to meet specific patient needs, including CPAP, BIPAP, average volume assured pressure support (AVAPS), auto-CPAP, BIPAP-autoSV (support ventilation), BIPAP S/T (spontaneous/timed), volume positive airway pressure (VPAP), Smart CPAP, and demand positive airway pressure (DPAP). Each mode has specific advantages that must be matched to the patient’s specific SDB needs. For this reason, a titration sleep study must be completed in the sleep laboratory under direct supervision of a trained clinician to allow titration and adjustment of PAP equipment during the night. Some physicians and medical equipment companies attempt to provide treatment for documented SDB without a titration study, but patient compliance and continued use is threatened by this practice. Current manufacturers of PAP devices provide multiple-mode devices that are small, quiet, and efficient. Patients using PAP must be able to transport the device and all associated equipment safely when traveling away from home; the size of the device has a significant impact on patient compliance during travel.8,11,21
As previously mentioned, the length of the REM stage normally increases through the night with the longest phase of REM typically just before waking. Because the REM state represents skeletal muscle paralysis and decreased ventilation efforts, the PAP device must be adjusted to meet all ventilation needs and supply complete support of flaccid or collapsing soft tissue in the oropharyngeal cavity. With the CPAP or BIPAP adjunct in place with the appropriate PAP device, the PSG titration study documents the PAP setting required to stent open the airways while the patient transitions through each stage of sleep. PAPs are adjusted in each sleeping position until all sleep parameters are normalized and documented with an AHI less than 5. Because the supine sleeping position is the patient’s most vulnerable position with regard to airway collapse, the therapist must ensure the PAP is titrated to the correct pressure during REM while the patient is supine. When this pressure is determined, all other stages of sleep and sleeping positions are likely to be supported with enough PAP for uninterrupted sleep.10
Oral Appliances
Known also as dental appliances, oral appliances are acrylic devices that fit over the upper and lower teeth similar to a mouth guard. An oral appliance is designed to move the lower jaw, or mandible, slightly forward. Because the tongue is attached to the mandible, the tongue is also advanced forward to provide a larger oropharyngeal lumen. A dentist experienced in the treatment of snoring and sleep apnea must customize the oral appliance for each patient. Two of the oral appliances in current use are the mandibular advancement device (MAD) and the tongue retaining device (TRD). The MAD device is designed with an adjustable mechanism that allows the patient to advance the mandible gradually daily, eventually increasing the oropharyngeal airway opening. The TRD fits around the tongue and holds it forward by means of suction; it is used most often by patients with lower dentures that are removed at night or by patients in whom the mandible cannot be effectively advanced. Both devices require the patient to be able to breathe through the nose adequately; otherwise, the oral appliance will not be tolerated (see Figure 15-15, A and B). Although oral appliances are an option for the treatment of OSA, results are marginally successful, and these appliances are effective only in patients diagnosed with mild OSA.22,23
Positional Therapy
Another approach to positional therapy involves pillows registered by the U.S. Food and Drug Administration (FDA) and specifically designed to prevent snoring and mild sleep apnea. These pillows are designed to reposition the neck so that the oral airway is more likely to remain patent. The foam wedge-shaped pillows are designed at angles of various degrees and presumably reduce positional collapse of the airways and reduce apneas and snoring. However, the manufacturer recommends that the patient sleep in the supine position on the positional pillows. At best, positional therapy may prove beneficial in reducing the number of apneic periods and snoring intensity in mild sleep apnea, but it has not been found to be effective in eliminating moderate or severe obstructive apnea.24
Airway Enlargement
The treatment of last resort for obstructive SDB is surgical enlargement of the oropharyngeal cavity to provide a more patent airway. Surgery entails the reduction of soft tissue structures and may be effective in some OSA conditions, but the patient should be fully aware of potentially adverse outcomes. A common surgical procedure for sleep apnea is uvulopalatopharyngoplasty (UPPP), which involves removal or shortening of the uvula, removal of the tonsils and adenoids, and excision of portions of the soft palate or roof of the mouth; the goal is enlargement of the airway lumen. The benefits of UPPP are controversial. Although 90% of patients experience symptomatic improvement from habitual snoring, studies have shown that only 41% to 66% of patients undergoing UPPP see improvement or elimination of OSA, with results worsening over time.25 The efficacy of UPPP cannot be predicted, and nonsurgical options should be considered before resorting to the procedure. Children with OSA should be assessed for enlarged tonsils and adenoids. Simple tonsillectomy/adenoidectomy has been effective in relieving snoring and sleep apnea in children.26,27
Other surgical treatment of OSA includes midline glossectomy and lingualplasty, in which part of the tongue is removed to enlarge the oral cavity. In addition, maxillomandibular advancement (MMA), which moves the mandible anteriorly, has been successful, but this procedure requires the jaw to be broken, extended, and wired shut until the mandible heals.28 Radiofrequency tissue ablation (RFTA) was approved by the FDA in 1998 and is used to shrink the size of the tongue and palate with high-frequency waves; the procedure can be performed in the physician’s office.29 The most effective surgical treatment of OSA is a tracheotomy, although it is deemed unacceptable by most patients. When a tracheotomy is performed for this purpose, the stoma is closed or plugged during the day and unplugged during the night.
The least invasive method to promote airway enlargement is weight loss. Multiple studies have shown that when patients with OSA lose a significant amount of weight, the lumen of the oropharyngeal airway is increased, and the reduced weight on the chest, airways, and abdominal cavity decreases work of breathing during sleep. Obese patients who lose more than 100 lb may see a reduction in PAPs needed; in some cases, OSA may completely resolve with weight loss.30 OSA is an anatomical issue not always associated with obesity; thin individuals may also experience OSA because of their airway anatomy.