Chapter 20 Physical Agent Modalities
Modalities are physical agents that are used to produce a therapeutic response in tissue. They include heat, cold, water, sound, electricity, and electromagnetic waves (including infrared, visible, or ultraviolet light; shortwaves; and microwaves) (Figure 20-1). This chapter focuses on these physical agent modalities, except for most of the therapeutic uses of electrical stimulation (which are covered in Chapter 21). These modalities are generally considered adjunctive treatments rather than primary curative interventions. This chapter reviews the physiologic effects, common uses, techniques of application, and precautions for the therapeutic use of modalities. Although there is a growing body of literature to support the use of physical agent modalities, further research is needed to more specifically define the efficacy and indications for these agents.17,159–162
Modality Prescription
The elements of a prescription for heat or cold are listed in Box 20-1. The condition for which the modality is being used should be clearly indicated. The location to be treated influences modality selection, in that large areas can preclude the use of modalities such as ultrasound or ice massage. The surface to be treated can also influence selection. If using ultrasound over an irregular surface, degassed water might be preferred over a gel coupling agent. If using superficial heat over an irregular surface, hot packs or heating pads can result in focal heating over prominences, so radiant heat might be preferred. Intensity should be indicated where appropriate (e.g., ultrasound power output, hydrotherapy, fluidotherapy, and paraffin bath temperature). Most modalities allow only qualitative dosimetry as currently used in physical medicine, and therefore rely on patient perception of thermal intensity for safety. The duration for most modalities is 20 to 30 minutes, except for ultrasound, which is typically 5 to 10 minutes per site. Frequency is based on the severity of the condition being treated and on clinical judgment. Although information about duration and intensity of treatment is noted in this chapter, it is intended only as a guideline, and should be modified by clinical experience and the clinical condition.
Modality selection is influenced by multiple factors (Box 20-2). In selecting a modality, one should recognize that there are few well-designed clinical trials demonstrating the efficacy of specific modalities in specific conditions. There are, however, numerous studies that review the physiologic effects of modalities. Having a firm understanding of the physiologic effects of a particular modality allows one to make an educated selection. One must understand the heating or cooling capabilities of the various modalities to ensure the selection of the proper modality for the target tissue. Body habitus influences modality selection in that subcutaneous adipose affects the depth of penetration of many modalities. Comorbid conditions should also be considered. For example, both cold and heat can have adverse effects in the patient with significant arterial insufficiency. Cold can have harmful effects via the production of arterial vasoconstriction, and heat can cause complications via the production of increased metabolic activity, which can exceed the potential increase in blood supply and produce ischemia. Age is also a factor in modality selection. In the pediatric population, ultrasound should generally be avoided near open epiphyses.186 In the elderly population, there can be comorbidities that affect modality choice. Gender can also play a role in modality use, because fetal malformations have been reported after ultrasound near a gravid uterus.133
Heat
Forms of heat can be broadly classified by depth of penetration and form of heat transfer (Table 20-1). Depth of penetration is arbitrarily divided into superficial and deep. Superficial heat includes hot packs, heating pads, paraffin baths, fluidotherapy, whirlpool baths, and radiant heat. Deep heating agents (or diathermies) include ultrasound, shortwave, and microwave. Mechanisms of heat transfer include conduction, convection, radiation, evaporation, and conversion (Figure 20-2). Conduction is the transfer of thermal energy between two bodies in direct contact. Convection uses movement of a medium (e.g., water, air, or blood) to transport thermal energy, although the actual transfer of thermal energy is ultimately by conduction. Radiation refers to the thermal radiation emitted from any body whose surface temperature is above absolute zero (–273.15° C or –459.67° F). Evaporation involves the transformation of a liquid into a gas, a process that requires thermal energy. Evaporation is actually a process of heat dissipation, and plays a role in cooling modalities such as vapocoolant sprays. For each gram of water that evaporates from the body surface, approximately 0.6 cal (calorie) of heat is lost.81 Conversion refers to the transformation of energy (e.g., sound or electromagnetic) to heat. Likewise, the human body converts protein, carbohydrates, and fats to thermal energy via numerous metabolic processes. The next section reviews the physiologic effects (Box 20-3) of the therapeutic use of heat, followed by discussions of the general uses of heat (Box 20-4), some general precautions (Box 20-5), and descriptions of the agents currently used in physical medicine.
Type of Heating | Depth | Main Mechanism of Energy Transfer |
---|---|---|
Hot packs or heating pads | Superficial | Conduction |
Paraffin baths | Superficial | Conduction |
Fluidotherapy | Superficial | Convection |
Whirlpool baths | Superficial | Convection |
Radiant heat | Superficial | Radiation |
Ultrasound | Deep | Conversion |
Shortwave diathermy | Deep | Conversion |
Microwave | Deep | Conversion |
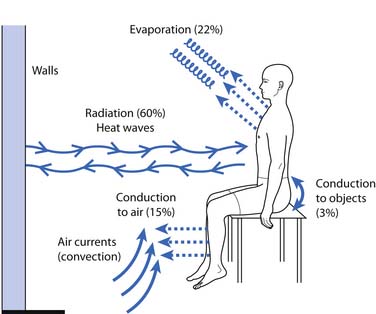
Figure 20-2 Mechanisms of heat transfer.
(Redrawn from Guyton AC: Body temperature, temperature regulation, and fever. In Guyton AC, edition: Textbook of medical physiology, 8th ed, Philadelphia, 1991, Saunders.)
Physiologic Effects of Heat
Hemodynamic
Localized heating produces a variety of hemodynamic effects. A twofold to threefold increase in forearm blood flow has been demonstrated after hydrotherapy at 44° to 45° C (111.2° to 113° F) or shortwave diathermy (SWD).1 This vasodilatation results in increased ingress of nutrients, leukocytes, and antibodies, and increased egress of metabolic byproducts and tissue debris, and can facilitate resolution of inflammatory conditions.80,173 Vasodilatation with heating can unfortunately also contribute to increased bleeding and increased edema formation, and can exacerbate acute inflammatory conditions.173 A number of experimental animal models of acute and chronic inflammation support the general clinical impression that acute inflammatory conditions tend to react unfavorably to heat, whereas chronic inflammatory conditions tend to benefit from heat.173
Neuromuscular
Animal experiments of localized heating have demonstrated increased firing rates in all group 1a fibers (muscle spindle) and many group 1b (Golgi tendon organ) fibers, and decreased firing rates in most group 2 fibers (muscle spindle).136 It should be noted that all experiments on muscle spindle and Golgi tendon organ firing rates are significantly influenced by the length and tension at which they are performed. This factor probably accounts for many of the differences among investigators.63,136 In contrast, the effects of temperature change on nerve conduction velocity are much more consistent. Heating produces modest increases in conduction velocity, whereas cooling is capable of producing dramatic decreases in conduction velocity. Abramson et al.1 noted increases in conduction velocity of up to 7.5 m/s after hydrotherapy at 44° to 45° C (111.2° to 113° F) or SWD, whereas cooling produced conduction velocity decreases of up to 35.8 m/s. The effects of temperature in clinical neurophysiology are succinctly summarized elsewhere.49
Joint and Connective Tissue
In vitro experiments demonstrate the importance of combined heating and stretching to maximize tendon extensibility. Lehmann et al.119 measured tendon extensibility under a variety of temperature and loading conditions. Tendon extensibility was greater at 45° C (113° F) than at 25° C (77° F). Furthermore, simultaneous use of heating and stretching produced significantly increased tendon extensibility when compared with the isolated use of either agent. Sustaining stretching during the cooldown period also facilitated tendon elongation. Other investigators have demonstrated metacarpophalangeal joint stiffness to decrease by as much as 20% at 45° C (113° F) compared with at 33° C (91.4° F).205 Temperature also affects enzymatic activity. In vitro experiments have shown a fourfold increase in collagenase activity with a temperature increase from 33° to 36° C (91.4° to 96.8° F).82
Miscellaneous Effects of Heat
It is generally accepted that heat produces an analgesic effect. A variety of mechanisms for the analgesic effect of heat have been postulated.65,112 These include a cutaneous counterirritant effect, vasodilatation resulting in decreased ischemic pain, vasodilatation resulting in washout of pain mediators, an endorphin-mediated response, alteration of nerve conduction, and alteration of cell membrane permeability. Elevation of the pain threshold has been demonstrated after therapeutic application of ultrasound, microwave, and infrared radiation.113 Many patients also find heat to have a general relaxation effect, although the specific mechanisms are not well defined.
General Uses of Heat in Physical Medicine
The general uses of heat are summarized in Box 20-4. These uses are based on the physiologic effects previously described. Heat is used in a variety of musculoskeletal conditions because of its potential to produce analgesia and muscle relaxation, and to facilitate resolution of inflammation. Increased soft tissue extensibility and decreased joint stiffness make heat useful in contractures and a variety of arthritides.
General Precautions for the Use of Heat
The general precautions for the use of heat are listed in Box 20-5. As noted earlier, heat can exacerbate acute inflammation and should generally be avoided in the acute management stage.173 In the patient with impaired circulation, increased metabolic activity with heating can exceed the capacity of arterial supply, so heat should be used with caution in this patient population. Vasodilatation from heat can result in increased bleeding in persons with bleeding diatheses. Vasodilatation can also produce increased edema. Because scars can be relatively avascular and have a reduced ability to dissipate heat, they can be selectively heated. Heat should generally be avoided in areas of impaired sensation because of the obvious potential for thermal injury as a result of the lack of precise dosimetry in modality use. This same reason justifies caution in using heat in patients with impaired cognition or communication, which precludes reporting of pain. Lehmann112 cautioned against the use of heat over malignancies because of the potential for increased rate of tumor growth or hyperemia increasing hematogenous spread. However, this should be distinguished from the use of specific local hyperthermia in the adjunctive treatment of malignancies.155 It also does not preclude the use of heat for adjunctive analgesia in the terminally ill cancer patient.
Superficial Heat
Superficial heating agents achieve their maximum tissue temperatures in skin and subcutaneous fat. Deeper tissue heating is limited by vasodilatation (which dissipates heat) and the insulating properties of fat. Superficial heating agents can heat via conduction (hot packs, heating pads, and paraffin baths), radiation (heat lamps), or convection (fluidotherapy and whirlpool baths). Superficial heat is used in osteoarthritis, rheumatoid arthritis, neck pain, low back pain, muscle pain syndromes, and a variety of musculoskeletal conditions.112
Hot Packs
Commercially available hot packs, such as Hydrocollator packs, typically contain silicon dioxide encased in a canvas pack. They come in a variety of sizes and styles for use over different areas. Hot packs are immersed in tanks at 74.5° C (166° F) and applied over several layers of insulating towels.122 After several minutes the skin should be inspected briefly to ensure that the heating is not excessive. Total treatment time is usually 30 minutes. Lehmann et al.122 showed that the 30-minute application of a hydrocollator pack to the posterior thigh produced approximately 3.3° and 1.3° C tissue temperature elevations at depths of 1 and 2 cm, respectively. Other investigators observed a 1.1° C temperature rise 4 cm deep in the brachioradialis muscle after a 30-minute application of a hydrocollator pack.2 A 1.2° C increase in intraarticular temperature (knee) has also been demonstrated after hot pack application.199 General heat precautions should be observed. The patient should not lie on the pack. This can squeeze water from the pack, wetting the insulating towels, thereby raising their thermal conductivity and increasing the risk of burns. The focal pressure from lying on a hot pack can also produce increased heating over bony prominences. Lying on the hot pack also puts the pressure of the weight of the body on the skin next to the pack. This pressure exceeds capillary pressure and makes it difficult for blood to come into the area to carry away the heat. Hot packs are among the more common causes of burns in physical therapy. This is because heat has sedative effects, and the patient is typically not directly supervised when this modality is used.
Heating Pads
Two main types of heating pad are available: electric heating pads and circulating fluid pads. Electric heating pads usually control heat output by regulating current flow. Circulating fluid (e.g., water) heating pads usually control heat output thermostatically. Peak temperatures of nearly 52° C (125° F) were achieved with an electric heating pad set on the lowest setting.50 Periodic temperature oscillations of up to 5° C were also noted.50 General heat precautions should be observed with heating pads. There is an obvious potential for electric shock, particularly when used in conjunction with moist toweling. Many commercially available heating pads are designed to be used in conjunction with moist toweling, but caution should be taken to inspect them regularly to ensure that all insulating materials are intact. The patient should not lie on a heating pad because this can result in focal temperature increases, leading to burns. This is of particular concern in the slender or cachectic patient with minimal subcutaneous adipose over bony prominences. Figure 20-3 shows a typical heating pad burn. The patient was an elderly woman who had not received adequate instruction in home use of her modalities, and had been repeatedly lying supine on an electric heating pad. Repeated and prolonged skin exposure to heat can result in erythema ab igne, a skin condition characterized by reticular pigmentation and telangiectasia, which has been noted after the use of a variety of superficial heating modalities.52
Radiant Heat
Radiant energy, including infrared radiation, is emitted from any substance with a temperature above absolute zero. Infrared is the portion of the electromagnetic spectrum adjacent to the long-wavelength, low-frequency (red) end of the visible spectrum. Luminous infrared heat lamps emit radiation in the near-infrared spectrum (wavelength 770 to 1500 nm), and nonluminous infrared heat lamps emit radiation in the far-infrared spectrum (wavelength 1500 to 12,500 nm).112 Infrared produces heating by inducing molecular vibration.52 A 1.3° C temperature rise has been noted at a depth of 2 cm after heat lamp application.122
The main determinants of intensity of radiant heating are distance and angle of delivery. The inverse square law states that the intensity of radiation varies inversely with the square of the distance from the source (Figure 20-4). This means that doubling the distance from a heat lamp reduces the heating intensity by a factor of four. Conversely, decreasing the distance from the heat lamp by half would increase the intensity fourfold. Typical distances are 30 to 60 cm from the patient’s body, depending on heat lamp wattage.112 The angle of delivery also affects the intensity of thermal radiation. Maximal radiation is applied when the source of radiation is perpendicular to the surface. As the angle away from perpendicular increases, the intensity of radiation decreases in proportion to the cosine of the angle.
Radiant heat is often preferable in patients who cannot tolerate the weight of hot packs. Caveats for the use of radiant heat include general heat precautions, light sensitivity, skin drying, and dermal photoaging.52,112 Some laboratory data suggest a potential for infrared acting synergistically with ultraviolet radiation in cutaneous photocarcinogenesis, but the clinical significance of this, if any, remains to be determined.52
Fluidotherapy
Fluidotherapy is a superficial, dry heating modality that uses convective heating with forced hot air and a bed of finely divided solid particles.20 This solid-gas system reportedly behaves like a heterogeneous fluid of low viscosity, a phenomenon labeled fluidization.19 Reputed advantages include the massaging action of the highly turbulent solid-gas mixture and the freedom to perform range-of-motion exercises.19 Peak hand muscle and joint capsule temperatures of nearly 42° C (107.6° F), and peak foot muscle and joint capsule temperatures of approximately 39.5° C (103.1° F), have been achieved after 20 minutes of fluidotherapy at 47.8° C (118° F).20 Both the temperature and the amount of agitation can be controlled. The typical temperature range is 46.1° to 48.9° C (115° to 120° F). Decreased degrees of agitation can be used for sensitive areas. Although fluidotherapy is touted for use in a variety of conditions, further study is needed to more objectively define its role in physical medicine.89 General heat precautions should be observed, and infected wounds should probably be avoided because of the risk of cross-contamination.
Paraffin Baths
A paraffin bath is a superficial heating agent that uses conduction as the primary form of heat transfer. Paraffin wax and mineral oil are mixed in a ratio of 6:1 or 7:1.112 Treatment temperatures are 52.2° to 54.4° C (126° to 130° F). These are tolerated because of the low heat conductivity of the paraffin mixture.112 A thermometer should be used to ensure proper temperature. A thin film of unmelted paraffin on the tank walls generally indicates a safe temperature. Methods of application include dipping, immersion, and brushing. After removing all jewelry, thoroughly wash and dry the area to be treated. The dipping method involves 7 to 12 dips followed by wrapping in plastic and towels or insulated mitts to retain heat.2 The immersion method involves several dips to form a thin glove of paraffin, followed by immersion for 30 minutes.2 The brushing method involves brushing on several coats of paraffin, followed by covering with towels. The brushing method is more cumbersome, and infrequently used in the adult population. However, children often find paraffin brushing to be fun, thereby improving their treatment compliance. Paraffin brushing can also be useful for areas difficult to immerse. For home treatment, patients can use a double boiler, although commercial paraffin tanks are reasonably inexpensive, probably safer, and more convenient. The equipment safety precautions should be carefully reviewed. General heat precautions apply to paraffin use. Open wounds and infected areas should be avoided.
The immersion method produces the greatest quantity and duration of temperature increase, with peak forearm subcutaneous tissue temperatures of 5.5° C over baseline, and brachioradialis temperatures of 2.4° C over baseline.2 The dip method produces a 4.4° C peak forearm subcutaneous tissue temperature rise and a 1.0° C brachioradialis temperature rise, but these temperature rises decrease significantly by 15 to 20 minutes postdipping.2 In a study of patients with scleroderma, paraffin baths in conjunction with friction massage and active range-of-motion exercise resulted in statistically significant improvement in skin compliance and overall hand function.6 In a study of patients with rheumatoid arthritis, statistically significant improvements in range of motion and grip function were noted after paraffin treatment in conjunction with active range-of-motion exercises, whereas paraffin baths alone had no statistically significant effect.47 This again emphasizes the importance of using exercise in combination with the modality.200,201
Deep Heat
Diathermy is derived from dia (“through”) and therme (“heat”), and refers to several forms of deep heating, namely shortwave, microwave, and ultrasound. Because the target tissue in physical medicine is generally muscle, tendon, ligament, or bone (rather than skin or subcutaneous fat), the goal of early investigators was to discover a mode of heating that minimized skin and subcutaneous tissue heating but maximized heating of deeper tissues.80 The challenge of diathermy developers was to discover a modality that could penetrate the skin and subcutaneous fat to produce a maximum temperature rise in underlying soft tissues. Conversely, the modality should not produce excessive temperatures in more superficial tissues (subcutaneous fat, being relatively avascular compared with muscle, is unable to adequately dissipate heat via vasodilatation). The therapeutic target temperature is generally considered to be 40° to 45° C (104° to 113° F).80 Lower temperatures might not produce adequate vasodilatation and increased metabolism, whereas higher temperatures can result in tissue damage. Because the thermal pain threshold is approximately 45° C (113° F), pain perception can be used to monitor intensity of heating in the neurologically intact and alert person.80 One gradually increases modality intensity to the earliest pain perception, then slightly decreases intensity. There is, however, only a fine line between the therapeutic temperature range and potential thermal injury.
Ultrasound
Ultrasound is defined as acoustic vibration with frequencies above the audible range (i.e., >20,000 Hz). Medical uses of ultrasound can be diagnostic or therapeutic. Diagnostic ultrasound is used for a variety of obstetric, urologic, cardiovascular, and other imaging studies, and is outside the scope of this chapter. Therapeutic ultrasound involves the use of high-frequency acoustic energy to produce thermal and nonthermal effects in tissue. Ultrasonic signals are typically generated using the reverse piezoelectric effect. Certain quartz crystals and synthetic ceramics have piezoelectric characteristics, such that when they vibrate they produce an electric current.188 The reverse happens when an electric current is passed across such crystals, causing vibration at a specific frequency.
As ultrasonic waves travel through tissue, they lose a proportion of their energy, a process called attenuation.208 Attenuation in tissue is produced by several mechanisms: absorption, beam divergence, and deflection.208 Absorption is the major cause of ultrasound attenuation.208 Ultrasonic energy is absorbed by the tissue and is ultimately converted into heat.208 For most tissues, attenuation increases as frequency increases, so a 1.0-MHz signal would penetrate deeper than a 3.0-MHz signal because of its lower attenuation by the tissue.208 Beam divergence is the amount that the beam spreads out from the transducer. Beam divergence decreases as frequency increases, so a higher frequency signal has a more focused beam.
Deflection includes the processes of reflection, refraction, and scattering.208 The angle of the reflected wave is equal to the angle of the incident wave (Figure 20-5). The magnitude of the reflected wave depends on the difference in acoustic impedance between the tissues on each side of the reflecting surface.208 Acoustic impedance is a measure of the resistance to the transmission of a sound wave, and is the product of the velocity of sound and the density of the medium.188,208 The magnitude of the reflected wave is proportional to the following formula208:
where A2 is the acoustic impedance of tissue 2 and A1 is the acoustic impedance of tissue 1. The greater the mismatch of acoustic impedance of the two tissues, the greater the magnitude of the reflected wave. Because the impedance mismatch between skin and air is extremely high, essentially all the acoustic signal is reflected if a coupling agent is not used (see below).208 Refraction is a deviation of beam direction as it is transmitted between two media (see Figure 20-5). The angle of the transmitted (refracted) wave is determined by the velocity of sound in the two media, and is given by Snell’s law208:
where sine 1 is the sine of the incident wave, sine 2 is the sine of the transmitted wave, velocity 1 is the velocity of sound in the first tissue, and velocity 2 is the velocity of sound in the second tissue. Scattering is the last mechanism of beam deflection. It occurs when surface irregularities scatter the signal. Scattering is minimized when surface irregularities are small with respect to the wavelength.
Parameters for therapeutic ultrasound are noted in Table 20-2. Frequency is generally in the range of millions of cycles per second (or megahertz). The most commonly used frequencies in the United States are in the range of 0.8 to 1.1 MHz, although frequencies around 3.0 MHz are also fairly common. Power is total energy per unit time, whereas intensity is power per unit area. Intensity can be expressed in terms of peak or average intensity, and spatial or temporal intensity, and is indicated in units of watts per square centimeter. These different measures of intensity are defined in Table 20-2. The World Health Organization and the International Electrical Commission both recommend limiting spatial average intensity to 3 W/cm2.88 Most clinically used intensities of therapeutic ultrasound are in the range of 0.5 to 2.0 W/cm2. Temperatures of up to 46° C (114.8° F) in deep tissues (e.g., bone-muscle interface) are easily achieved with ultrasound.115–117 If very deep heating (e.g., of the hip joint) is the goal, ultrasound appears to be superior to microwave or SWD.121
Parameter | Details |
---|---|
Frequency | Millions of cycles per second (MHz) |
Power | Total energy per unit time (W) |
Effective radiating area | The area of the transducer that actually radiates ultrasonic waves (cm2) |
Intensity Spatial average intensity Spatial peak intensity Temporal average intensity Temporal peak intensity |
May be expressed in terms of peak or average and spatial or temporal (W/cm2) Total power output divided by effective radiating area Maximal intensity anywhere within the beam Average intensity of “on” and “off” periods of a pulsed signal Maximal intensity of “on” period of a pulsed signal |
Duration | Generally 5-10 minutes per site |
Additional parameters for pulsed ultrasound Pulse duration Pulse repetition period Pulse repetition frequency Duty factor |
Time of actual ultrasound pulse (“time on”) Time interval from one pulse to the next (“time on” and “time off”) Number of pulses per second Fraction of total time during which ultrasound is emitted (calculated by dividing pulse duration by pulse repetition period) |
Ultrasound delivery can be continuous or pulsed. Pulsed delivery involves the emission of brief bursts or pulses of ultrasound, interspersed with periods of silence (Figure 20-6). For pulsed ultrasound delivery, additional parameters should be indicated.188 These include pulse duration, pulse repetition period, pulse repetition frequency, and duty factor, and are defined in Table 20-2. In most machines available for use in the United States, selecting a duty factor automatically determines the other parameters. Duty factor commonly ranges from 10% to 50%. For example, a 2-ms pulse duration might be interspersed with 8 ms of silence. The pulse repetition period would be 10 ms, the pulse repetition frequency would be 100 Hz, and the duty factor would be 20%. Pulsed delivery, especially at low duty factors, results in less heating than continuous wave ultrasound, and thereby presumably emphasizes nonthermal effects.
The physiologic effects of ultrasound can be divided into thermal and nonthermal effects. Thermal effects are produced when acoustic energy is absorbed, producing molecular vibration, which results in heat production.36 Nonthermal effects include cavitation, media motion (acoustic streaming, microstreaming), and standing waves. Cavitation is the production of gas bubbles in a sound field.36 These bubbles can expand and contract with alternating compressions and rarefactions of a sound wave.36 Stable cavitation refers to bubbles that oscillate in size within the sound field.57 Unstable cavitation refers to bubbles that continue to grow in size and then collapse. The high temperatures and pressures generated by this can produce platelet aggregation, localized tissue damage, and cell death.57 Both forms of cavitation are capable of mechanical distortion, movement of material, and alteration of cellular function, but their clinical significance is not yet clearly defined.36,138
Media motion includes acoustic streaming and microstreaming. Acoustic streaming is defined as unidirectional movement in an ultrasonic pressure field, and results from an ultrasonic wave traveling through a compressible medium.57 Acoustic microstreaming is produced by stable cavitation. As the stable cavitation bubbles oscillate in size, the surrounding fluid is set in motion, with nearby particles being attracted to the oscillating bubble.57 In addition to movement of material, acoustic streaming and microstreaming can result in cell membrane damage and accelerate metabolic processes.57
Standing waves are produced by the superimposition of incident and reflected sound waves, and can result in focal heating at tissue interfaces of different densities.57 Stasis of red blood cells at one-half wavelength intervals in a sound field has also been demonstrated in the laboratory.58 Although numerous subcellular, cellular, and tissue nonthermal ultrasound effects have been reported, their clinical significance remains to be elucidated.36,43,57,58,138 Certain measures can minimize the nonthermal effects of ultrasound. Higher frequency, lower intensity, and pulsed delivery mode minimizes acoustic cavitation.43 Stroking technique of application minimizes standing wave formation.57
As noted previously, the amount of ultrasound reflected at an interface between two media depends on the difference in acoustic impedance, so the ideal coupling medium is one with acoustic impedance similar to that of tissue. Three factors have an impact on the effectiveness of a coupling medium:
Degassed water is a commonly used coupling medium and is used to prevent bubble formation on the skin surface. Allowing tap water to stand overnight typically allows for adequate gas evaporation. In tests of acoustic transmissivity, mineral oil and several commercially available coupling gels had similar transmissivities to the reference standard of distilled degassed water.195 However, the hydrocortisone phonophoresis coupling agents tested had a significantly lower transmissivity, presumably related to microscopic air bubbles introduced into the media. Variation in transducer pressure produced dramatic differences in transmissivity, which outweighed the differences between the various coupling media. The investigators concluded that coupling media can be chosen primarily on the basis of cost and convenience, without compromising function.195 Encased silicon gel shows promise for use as a coupling agent over irregular body surfaces, sensitive skin areas, and open wounds, if the issue of impedance mismatch with the sound head can be rectified.8 In addition to accommodating for impedance mismatches, coupling media also lubricate to permit smooth movement of the transducer over the skin.
The most common technique of ultrasound application is the stroking technique. It allows a more even energy distribution over the site being treated. The applicator is moved slowly over an area of approximately 25 cm2 (4 square inches) in a circular or longitudinal manner.112 The applicator size (usually 5 to 10 cm2) limits the size of the area that can be treated, so multiple fields of treatment might be needed for larger areas (i.e., shoulder and hip may require anterior, lateral, and posterior ports). The stationary technique generally should be avoided because of the potential for standing waves and the production of hot spots.148 Intensity is indicated by specifying the particular watts per square centimeter or by titrating to just below pain threshold. In surveys of performance of ultrasonic therapy equipment, variations in frequency and power output were common.88,182 Although the frequency of commercially available ultrasound applicators was typically within 5% of manufacturer’s specifications, the overwhelming majority (85%) of applicators tested had power output variations of over 20%.182 Consequently it is important to have ultrasound applicators recalibrated regularly.
More than 35 clinical uses of ultrasound have been described, but many of these are not well supported by experimental evidence.186 More recent systematic reviews have not conclusively shown the benefits of ultrasound in the care of a number of musculoskeletal and related conditions, for similar lack of evidential support.∗ The purported clinical uses of ultrasound continue to expand, however, as witnessed by the current clinical interest in fracture healing, especially of bony nonunions,63,64,102, 104 and in chronic wound healing.†
Ultrasound precautions are summarized in Box 20-6.133,148,186 Concern about the use of heat near malignancies was discussed previously. Deep heating over an open epiphysis could result in either increased growth (from hyperemia) or decreased growth (from thermal injury). Avoiding ultrasound near pacemakers is reasonable because of potential thermal or mechanical injury to the pacemaker. Ultrasound over laminectomy sites could theoretically result in spinal cord heating. Ultrasound at therapeutic dosage over the peroneal nerve has been shown to produce a reversible conduction block in some patients with polyneuropathy.94 There are also case reports of increased radicular pain with ultrasound.72 There are even case reports of patient abuse of ultrasound.125 The concern with ultrasound use over arthroplasties and other metallic implants is the potential for focal heating. Gersten71 reported that temperature rises near metal were actually lower than temperature rises near bone, so metal per se should not be a contraindication to ultrasound. Lehmann112 cautioned against the use of ultrasound near methyl methacrylate or high-density polyethylene because of their high coefficient of absorption. The effect of ultrasound on bony ingrowth arthroplasties is not yet well defined. Because the effects of ultrasound on arthroplasties are not yet completely delineated, the most prudent course would be to avoid ultrasound over these areas whenever possible.
Shortwave Diathermy
SWD is a modality that produces deep heating via conversion of electromagnetic energy to thermal energy. Oscillation of high-frequency electrical and magnetic fields produces movement of ions, rotation of polar molecules, and distortion of nonpolar molecules, with resultant heat generation.73,99 The Federal Communications Commission limits industrial, scientific, and medical use to 13.56 MHz (wavelength, 22 m), 27.12 MHz (wavelength, 11 m), and 40.68 MHz (wavelength, 7.5 m).101 The 27.12-MHz frequency is most commonly used. The heating pattern produced depends on the type of shortwave unit and on the water content and electrical properties of the tissue. Tissues can be grossly divided into those with high water content (muscle, skin, blood, etc.) and those with low water content (bone, fat, etc.).99
SWD units can be inductive or capacitive. Inductive applicators use induction coils that apply a magnetic field to induce circular electrical fields in the tissue.80 They achieve higher temperatures in water-rich tissues with higher conductivity.101 These applicators typically have a cable or drum configuration.101 Cables are semiflexible induction coils that can be formed to the contour of the area to be treated. Drum applicators consist of induction coils enclosed in a rigid housing or drum. For a capacitive applicator, the patient is placed between two metal condenser plates (Figure 20-7). The plates and the patient’s intervening tissue act as a capacitor (an object that stores electrical charge), and heat is generated by rapid oscillations in the electric field from one plate to the other.73 Capacitive applicators might achieve higher temperatures in water-poor tissues such as subcutaneous adipose tissue.80,101
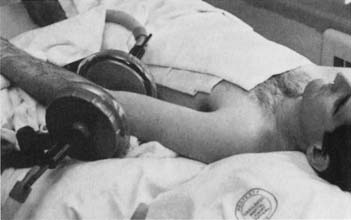
Figure 20-7 Example of shortwave diathermy application with capacitive applicator (condenser plates).
(From Kotke FJ, Lehmann JF, editors: Krusen’s handbook of physical medicine and rehabilitation, ed 4, Philadelphia, 1990, Saunders, with permission.)
Currently available shortwave applicators do not allow precise dosimetry, so initial pain perception is used to monitor intensity. Terrycloth towels are used for spacing and to absorb sweat, which is highly conductive and could result in potentially severe focal heating.112 Typical treatment time is 20 to 30 minutes. Specific applicator configuration can greatly affect the distribution of heating.101,120 In a review of several different induction applicators, the ratio of muscle heating versus fat heating ranged from 0.39 to 2.67 for the various applicators tested.120
Lehmann et al.114 evaluated the effect of subcutaneous fat thickness and technique of SWD application. In comparing distance from the applicator, 2 cm of air space between patient and applicator produced a more desirable heating pattern than did 3 mm of terrycloth between patient and applicator. Depth of subcutaneous fat also had a significant effect on temperature distribution. Muscle temperature rise in subjects with less than 1 cm of subcutaneous fat was 9.5° C, compared with 5.6° C in subjects with more than 2 cm of subcutaneous fat.114 After application of pulsed SWD, Draper et al.54 noted mean temperature increases of 1.36° C at 5 minutes, 2.87° C at 10 minutes, 3.78° C at 15 minutes, and 3.49° C at 20 minutes, at a depth of 3 cm in the gastrocnemius muscle. SWD may also decrease musculotendinous stiffness and facilitate soft tissue extensibility.139,168 SWD has been purported to be useful in a variety of musculoskeletal conditions, although, as with most of the physical agent modalities, there are conflicting data regarding efficacy.∗ SWD precautions are listed in Box 20-7. Metal can result in focal heating and should be strictly avoided. All jewelry should be removed, and the treatment table ideally should not contain metal. Likewise, electromagnetic energy can seriously interfere with pacemaker function, so a pacemaker is an absolute contraindication. Severe central nervous system damage has been reported after use of SWD near a deep brain stimulator.147 Contact lenses are a concern because of the potential for focal heating.174 The other precautions listed are self-explanatory. Regular maintenance and calibration of equipment should be performed to ensure safe operation of SWD.5 Special attention should also be given to ensure operator safety during SWD use.177,179
Microwave Diathermy
Microwave diathermy is another form of electromagnetic energy that uses conversion as its primary form of heat production. Thermal energy is produced by increased kinetic energy of molecules within the microwave field.153 Federal Communications Commission–approved frequencies for therapeutic microwave are 915 MHz (wavelength, 33 cm) and 2456 MHz (wavelength, 12 cm).112 The lower frequency has the advantage of increased depth of penetration but also the disadvantages of greater beam dispersion and the requirement of larger applicators.153
Temperature distribution in a particular tissue is affected largely by its water content. The fraction of power absorbed in a particular tissue depends on several factors, including the frequency of the electromagnetic wave, the dielectric constant, and the electrical conductivity of the tissue.153 In general, tissues with high water content absorb greater amounts of energy and are selectively heated.112 If muscle heating is a primary objective, 915-MHz applicators are preferable to 2456-MHz applicators.118 Nonthermal effects of microwave diathermy have been documented, but Lehmann112 points out that there is no evidence that these are of any therapeutic significance. Average temperatures of approximately 41°C (105.8°F) at a depth of 1 to 3 cm have been demonstrated.46 Microwave, although once quite popular, has been largely replaced by other modalities.177
General heat precautions should be observed with microwave. Metal implants, pacemakers, sites of skeletal immaturity, reproductive organs and brain, and fluid-filled cavities (eye, bullae, effusions, etc.) should be avoided.100,112
Cryotherapy
All forms of cryotherapy (therapeutic use of cold) are considered superficial cooling agents, usually transferring thermal energy by conduction. Exceptions include convective cooling in the whirlpool and evaporative cooling with vapocoolant sprays (Table 20-3). This section reviews the physiologic effects (Box 20-8), general uses (Box 20-9), and general precautions (Box 20-10) for cryotherapy, followed by a discussion of the agents currently used in physical medicine.
Type of Cooling | Depth | Main Form of Energy Transfer |
---|---|---|
Cold packs | Superficial | Conduction |
Ice massage | Superficial | Conduction |
Cold water immersion | Superficial | Conduction |
Cryotherapy-compression units | Superficial | Conduction |
Vapocoolant spray | Superficial | Evaporation |
Whirlpool baths | Superficial | Convection |
Physiologic Effects of Cold
Hemodynamic
Application of cold to the skin results in immediate cutaneous vasoconstriction through sympathetically mediated reflex mechanisms and by directly stimulating smooth muscle contraction.81,156 Lewis127 observed phasic oscillations of temperature in the fingers after initial vasoconstriction during cold water immersion. The initial vasoconstriction is thought to be due to a cold-induced increase in the affinity of the postjunctional α-adrenergic receptors for existing norepinephrine in vascular smooth muscle.178 Reactive vasodilatation occurs as further cooling interrupts noradrenaline release. Vasodilatation warms the tissues, again releasing norepinephrine to sensitized receptors, and the cycle repeats. This “hunting” of temperature is believed to be a mechanism by which peripheral exposed parts of the body are protected from cold injury. Others have demonstrated vasodilatation after cooling of the hand and forearm, without the phasic oscillations observed by Lewis.35,67 The effects of localized cooling on heart rate and blood pressure are variable and have been summarized elsewhere.134 Cryotherapy has also been shown to moderate inflammation, more effectively in the acute phase than in the chronic phase.173
Neuromuscular
The initial response of peripheral nerves to cold application is a marked slowing of conduction velocity.1,49 With more prolonged exposure, there is conduction block, cessation of axoplasmic transport, and eventual axonal degeneration.146 Decreased muscle spindle (both group 1a and 2 fibers) and Golgi tendon organ (group 1b fibers) firing rates have been demonstrated after local cooling in animals.62 The clinical neuromuscular effects of cooling include a decrease in gastrocnemius muscle stretch reflex amplitude,17,109 an increase in maximal isometric strength,34,132 and slowing in the rate of muscle fatigue.34 Cold has also been shown to temporarily reduce spasticity in patients with hemiplegia and multiple sclerosis, improving exercise tolerance and enhancing function.87,137 Other investigators, however, have noted increased spasticity after cold application in some subjects.31,164
Joint and Connective Tissue
Topical cold application (approximately 4° C) to the knee in dogs induced significant and sustained depression of intraarticular temperature without significant effect on core temperature.18 Synovial collagenase activity also decreases with temperature. In vitro experiments demonstrate negligible synovial collagenase activity after cooling to 30° C (86° F), suggesting that therapy that decreases intraarticular temperature in inflammatory arthropathies could slow the rate of collagenolysis.82 It should be noted, however, that in one study of the use of cryotherapy after cruciate ligament surgery, skin temperature had to be lowered to 20° C to produce significant intraarticular temperature declines.41 Potentially negative effects such as decreased tendon extensibility and increased joint stiffness have also been demonstrated after cooling.119,205
General Uses of Cryotherapy in Physical Medicine
The general uses for therapeutic cold are summarized in Box 20-9. Cryotherapy is most commonly used acutely after musculoskeletal injury to minimize formation of edema, and for symptomatic relief in painful soft tissue and articular inflammatory states.75,92,134,135,189 Two recent reviews nicely summarize the use of modalities in arthritis.83,151 Studies of the use of cryotherapy after orthopedic procedures have produced mixed results: some investigators report favorable effects on factors such as range of motion, pain, analgesic use, swelling, postoperative blood loss, and hospital length of stay, while others demonstrate no significant effect from cryotherapy.∗ There have also been mixed results with the use of cryotherapy in spasticity management.31,87,137,164,196 Cryotherapy in the form of immediate ice water immersion is advocated as emergent primary treatment for minor burns.181
General Precautions for the Use of Cold
The general precautions for the use of cold are outlined in Box 20-10. The most common relative contraindication to the use of cold is simple cold intolerance. A patient who does not tolerate cold application will tend to increase muscle guarding and cocontraction, which is directly counterproductive to the therapeutic goals. Simple cold intolerance should be distinguished from true cold hypersensitivity, which is discussed below. Caution should also be used when applying cold over the course of superficial nerves, because peroneal and ulnar palsies have been reported after cryotherapy.55 Application of cold over areas of compromised arterial vascularity can theoretically produce further ischemia from local vasoconstriction and should be avoided. Likewise, because of the potential for cold injury, cryotherapy should be used with caution in areas of impaired sensation or in patients who have cognitive or communication deficits that preclude reporting of pain.
The cryopathies are also contraindications to the use of cold.167 Cryoglobulinemia is a condition that results in the precipitation of immune complexes at low temperatures. Paroxysmal cold hemoglobinuria is a rare disorder resulting from an antibody directed against a red blood cell surface antigen, with hemolysis precipitated by cold exposure. Cold hypersensitivity is a mast cell–mediated process producing urticaria and angioedema on exposure to cold. Raynaud disease is an idiopathic condition characterized by arteriolar spasm precipitated by cold exposure or stress. Raynaud phenomenon is secondary to other rheumatologic conditions (rheumatoid arthritis, scleroderma, systemic lupus erythematosus, etc.) and is classically manifested by digital pallor, followed by cyanosis and eventual reactive hyperemia.
Cryotherapy Agents
Cold packs
Cold packs include hydrocollator packs, endothermic chemical gel packs, and ice packs. Hydrocollator packs are cooled in a freezer to –12° C (10° F) and applied over a moist towel.109 Endothermic chemical gel packs have separate compartments with compounds such as ammonium nitrate and water that when mixed, undergo a heat-absorbing reaction. They are portable, pliable, and easily used in the field. Although many endothermic chemical gel packs are designed for one-time use, some have the advantage that they can be refrozen and reused as a simple cryogel pack. Ice packs are easily used at home and might be best applied by elastic bandage or tape, as external compression appears to increase their cooling effectiveness.12 The duration of application is typically 20 to 30 minutes. With application of a cooled hydrocollator pack, the skin is cooled immediately, subcutaneous tissues are cooled within minutes, and muscle at a depth of 2 cm is cooled by approximately 5° C after 20 minutes.109 Precautions for cold packs are as listed in Box 20-10.
Ice Massage
Ice massage is the direct application of ice to the skin using gentle stroking motions. It combines the therapeutic effects of cooling with the mechanical effects of massage. Water is frozen in a paper cup, with the ice being exposed by tearing the top rim of paper off as the ice melts (Figure 20-8). Alternatively, a wooden tongue depressor can be placed in the water, to be used as a handle after the water is frozen. Ice massage is generally used for localized symptoms and applied for 5 to 10 minutes per site, depending on the amount of subcutaneous adipose tissue.129 There is typically a phasic response to ice massage, beginning with an initial perception of coolness, followed by a burning or aching, then hypoesthesia and analgesia.75 Intramuscular temperatures at a depth of 2 cm after 5 minutes of ice massage have been reported to be reduced by 4.1° C in the posterior thigh197 to as much as 15.9° C in the biceps brachii muscle.129 In his initial description of ice massage, Grant75 reported a “satisfactory treatment result” in over 80% of a series of more than 7000 patients with a variety of painful musculoskeletal conditions treated with ice massage and mobilization exercises, although no statistical analysis was reported. Precautions for ice massage are as listed under general cold precautions.
Cold Water Immersion
Immersion in cold water is best suited for circumferential cooling of the limbs, usually at temperatures of 5° to 13° C (41° to 55.4° F).181 It is often uncomfortable and poorly tolerated, although reportedly effective with localized burns, as skin temperature rapidly approaches water temperature.181 Gastrocnemius muscle temperature decreases to approximately 6° C below baseline after 30 minutes of cold water immersion.137,158 Precautions for cold water immersion are listed in Box 20-10.
Cryotherapy-Compression Units
Cryotherapy-compression units consist of a cuff or boot through which cold water is circulated, and can be pneumatically compressed statically or in a serial, distal-to-proximal pumping action. They are designed to combine the beneficial effects of cryotherapy with the advantages of pneumatic compression. They are used primarily after acute musculoskeletal injury with soft tissue swelling, and after some surgical procedures.171 Typical temperatures of 7.2° C (45° F) and pressures up to 60 mm Hg are used.
Vapocoolant Spray
Vapocoolant spray and stretch methods are used by some practitioners to treat myofascial and musculoskeletal pain syndromes. The technique consists of a series of unidirectional applications of Fluori-Methane spray, which has replaced the highly flammable ethyl chloride spray in clinical usage.189 Treatment begins in the “trigger area” (area of deep myofascial hypersensitivity) and extends over the “reference zone” (area of referred pain) while passively stretching the muscle.189 The spray and stretch are performed parallel to the muscle fibers, at an approximate rate of 4 inches/s. Waiting briefly between applications helps prevent skin freezing. The therapeutic effect of spray and stretch is postulated to result from a counterirritant phenomenon.189 Precautions include general cold precautions and avoidance of cutaneous freezing.
Hydrotherapy
Hydrotherapy is defined as the external application of hot or cold water, in any form, for the treatment of disease.69 The main forms of hydrotherapy are whirlpool baths, the Hubbard tank, the shower cart, and contrast baths. Their primary uses are in arthritis and a variety of musculoskeletal conditions, and in the cleansing and debridement of burns and other dermal injuries.
Whirlpool Baths and Hubbard Tanks
Whirlpool baths and Hubbard tanks control water temperature and agitate it by aeration, dispersing thermal energy by convection (although as noted previously, the actual transfer of heat to or from the body is by conduction). Whirlpool baths come in a variety of sizes and are typically used for treatment of a limb or localized lesion. Because only a portion of the body is immersed, greater extremes of temperature can be tolerated without significant core body temperature change.69,158 As more body surface area is immersed and the extremes of temperature increase, there is increasing potential for alteration of core body temperature. Hubbard tanks are larger tanks generally used for whole-body immersion, so neutral temperatures (34° to 36° C, 93° to 97° F) should be used to prevent core temperature fluctuations.
An immersed body experiences a vertical antigravity force equal to that of the volume of the displaced water, decreasing stress on bones and joints.69 This property, along with the therapeutic effects of the water temperature, makes hydrotherapy appropriate for adjunctive treatment of degenerative arthritis, acute musculoskeletal injuries, burns, and skin ulceration23 and infection.69 Antiseptic conditions can be used for hydrotherapy of burns or infected areas, but truly sterile conditions are not easily achieved, necessitating provider vigilance in patient selection, given reports of Pseudomonas infections.190 Sodium hypochlorite is the most commonly used antibacterial solution used in burn programs.187 For Hubbard tank treatment of large wounds, salt can also be added to minimize fluid shifts. Isotonic saline is 0.9% sodium chloride (0.9 g of NaCl, 100 mL of H2O), so 900 g (approximately 2 lb) of salt should be added per 100 L (approximately 25 gallons) of water.
Shower Cart
Hydrotherapy has several features that are desirable for treatment of burns or other wounds. It loosens adherent dressings to facilitate removal, allows for removal of antimicrobial cream before reapplication, and softens eschar to facilitate debridement.194 There is a risk for autocontamination and cross-contamination, however, with conventional whirlpool baths or Hubbard tanks.194 The shower cart was developed in response to this risk.194 It allows for gentle spray or shower hydrotherapy during mechanical debridement of large surface area burns and other wounds under relatively sterile conditions.194 Typical units have overhead retractable shower heads (Figure 20-9), with independently adjustable water temperature and pressure. A shower cart also uses significantly less water and less space, and requires less maintenance than a Hubbard tank (see Chapter 58).
Contrast Baths
Contrast baths consist of alternating immersion of the distal limbs in hot (42° to 45° C, 108° to 113° F) then cold (8.5° to 12.5° C, 47° to 55° F) water.204 The effect is thought to be related to the cyclic vasoconstriction and vasodilatation produced by the temperature extremes. Thirty-minute treatment sessions are typical, beginning with a 10-minute immersion in hot water, followed by alternating immersions of 1 minute in cold and 4 minutes in hot water, and ending the session with a cold water immersion to theoretically limit swelling. The technique might be beneficial in the treatment of rheumatologic disease, neuropathic pain, or other chronic pain syndromes such as complex regional pain syndrome.
Other Modalities
Ultraviolet
Ultraviolet radiation is that part of the electromagnetic spectrum adjacent to the short-wavelength, high-frequency (violet) end of the visible light spectrum. Ultraviolet radiation has historically been used in physical medicine for the treatment of skin ulcers, but now its therapeutic use is almost exclusively dermatologic.143 Most current treatments with ultraviolet radiation use either ultraviolet A (wavelength 320 to 400 nm) or ultraviolet B (wavelength 290 to 320 nm) radiation.143 The minimal erythema dose can be used to titrate intensity, and is determined by exposing small areas of skin to different durations of ultraviolet radiation. The minimal erythema dose is that duration of exposure that produces erythema. Although originally used mainly in the treatment of psoriasis, there are now more than 30 dermatologic disorders for which ultraviolet radiation could be efficacious.143 Potential adverse effects include premature aging of the skin, nonmelanoma skin cancer, and cataracts.143
Iontophoresis
Iontophoresis is the migration of charged particles across biologic membranes under an imposed electrical field.123 Its primary use has been in transcutaneous systemic or local delivery of medicines. For systemic drug delivery, it avoids the problems associated with oral or intravenous routes such as gastric irritation, first-pass hepatic metabolism, and variable serum concentrations.32,33 Iontophoresis in physical medicine is used to deliver medicines directly to soft tissues, limiting systemic absorption.30,38,128 Ionic medications (local anesthetics, corticosteroids, analgesics, and antibiotics) or plain tap water has been used with this technique. The ionic solution to be iontophoresed is placed on the electrode of the same polarity, and then the negative, positive, and ground electrodes are applied to the skin. A direct current, typically between 10 and 30 mA, is applied to drive the solution away from the electrode and into the surrounding tissues.180 The quantity of solution transported appears to be dependent on the local current density, the duration of treatment, and the solution concentration.150 Although the mechanism of action and the clinical efficacy of iontophoresis continue to be debated, it is a modality commonly used by practitioners to treat a variety of conditions.∗ Iontophoresis is generally well tolerated, although miliariasis has been reported when treating hyperhydrosis.180
Phonophoresis
Phonophoresis involves the use of ultrasound to facilitate transdermal migration of topically administered medications. Corticosteroids are the most frequently used phonophoresis agents. The antiinflammatory effects of ultrasound and corticosteroids are thought to be synergistic.144 The actual mechanism of transdermal migration has not been well defined but could involve increased cell permeability from the thermal effects of ultrasound.144 Ultrasonic coupling gel is mixed with various chemical substances to produce the phonophoresis coupling agent.144 Studies have documented significant differences in acoustic transmission among different phonophoresis agents, and these differences should be considered in choosing an agent.27 Typical phonophoresis treatment parameters are similar to those of standard ultrasound: pulsed mode, 1-MHz transducer frequency, stroking technique, at 1.0 to 1.5 W/cm2, for approximately 5 minutes per site.144 There is also low-frequency phonophoresis that typically uses frequencies less than 100 kHz.140 Proposed indications for phonophoresis include osteoarthritis, bursitis, capsulitis, tendonitis, strains, fasciitis, epicondylitis, tenosynovitis, contracture, scar tissue, neuromas, and adhesions.77,144 Phonophoresis is a modality with some measure of clinical effectiveness, but there continue to be differences of opinion about its mechanism of action, clinical efficacy, and the extent of transdermal migration of agents.† The precautions for and contraindications to phonophoresis are listed in Box 20-6.
Low-Energy Laser
Laser is the acronym for light amplification by stimulated emission of radiation. It consists of a coherent (in phase), collimated (restricted in area) beam of photons of identical frequency. Low-energy lasers typically deliver less than 90 mW and should be distinguished from the higher power (10 to 100+ W) lasers used in surgery, dermatology, and ophthalmology. Low-energy lasers deliver minimal energies (between 1 and 4 J) and can be considered a form of intense, focal light therapy.13 They have been shown to affect many subcellular and cellular processes, although the mechanisms have not been well defined.13 However, it is important to note that the low-energy laser does not produce significant tissue temperature changes, so any potential physiologic effects appear to be nonthermal. Low-intensity laser therapy has been used experimentally to treat a wide variety of clinical conditions, but no consensus regarding indication or effectiveness has been established.∗ There is also wide variation in equipment, experimental design, and stimulation parameters reported in the low-energy laser therapy literature, and some think this may be responsible for the conflicting results.13,172 The low-energy laser was recently approved by the Food and Drug Administration for selected indications. Protective goggles should be worn by both the patient and the treating therapist to protect against potential retinal injury.
Interferential Current Therapy
Interferential current therapy (IFC) is a modality that uses two alternating current signals of slightly different frequency. To understand the mechanism of IFC, one must have an understanding of how waves interact. Figure 20-10 illustrates that interaction. At the intersection of two sinusoidal waveforms, the “interference” of the two waves produces a summated wave. When the two waves are in phase, there is constructive interference; the summated wave has an amplitude equal to the sum of the individual wave amplitudes.74 When the two waves are out of phase, there is destructive interference; the summated wave has a lower amplitude because the opposite polarities of the waves partially cancel each other.74 When two waves of equal amplitude and frequency are exactly in phase, the amplitude of the summated wave is exactly twice that of the individual waves. When two waves of equal amplitude and frequency are out of phase by exactly one-half wavelength (i.e., the peak of one wave intersects with the trough of the other wave), the amplitude of the summated wave is zero. As noted, IFC uses two alternating current signals of slightly different frequencies. As these two waveforms intersect, they are periodically in phase (constructive interference) and periodically out of phase (destructive interference). This periodic interference results in a new wave with cyclic modulation of amplitude, because of the cyclic constructive and destructive interference. The amplitude of the summated wave modulates at a beat frequency, equal to the difference in frequency between the two signals.74 For example, if one applicator channel frequency is 5100 Hz and the other is 5000 Hz, the beat frequency would be 100 Hz (5100 – 5000 = 100).
IFC machines typically use medium-frequency currents of approximately 4000 to 5000 Hz. Alternating currents of medium frequency (1000 to 10,000 Hz) have lower skin resistance than low-frequency currents (<1000 Hz); it is therefore postulated that they penetrate tissue more easily.74,176 However, many factors other than signal frequency also affect skin resistance and depth of penetration.4,107,108 Most IFC machines use two, four, or six applicators. These can be arranged in the same plane (planar), as in the lumbar area, or in different planes (coplanar), as in the shoulder. Figure 20-11 demonstrates a typical quadripolar IFC application. The classic “cloverleaf” pattern of interference produced between two circuits in a perfectly homogeneous medium is diagrammed. However, because of the marked heterogeneity of electrical conductance of human tissues, the actual summation is unlikely to occur as precisely as in this diagrammatic representation.4
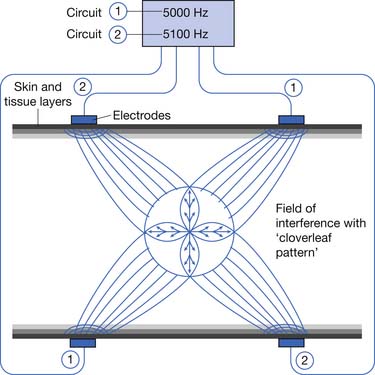
Figure 20-11 Quadripolar interferential current therapy application demonstrating classic “cloverleaf” pattern of interference.
(Redrawn from [Anonymous]: Nemectrodyn model 7 manual, Karlsruche, 1984, Nemectron, with permission of Nemectron.)
IFC parameters that can be manipulated include signal frequency, beat frequency, amplitude, and cycle time. IFC machines allow for either a fixed frequency difference (most machines are capable of producing beat frequencies from 1 to 120 Hz) or a modulated frequency difference, where the beat frequency varies over time.107 Frequency modulation is sometimes referred to as “sweep.”175 In theory, the use of frequency modulation limits neural adaptation.108 Amplitude can also be fixed or modulated. By manipulating the amplitude, the point of maximal amplitude interference changes. As Figure 20-12 shows, with equal amplitude in each circuit, the field of interference is at a 45-degree angle to the circuits. If the amplitude of circuit 1 is increased, the field will rotate toward circuit 1. Conversely, if the amplitude in circuit 1 is decreased, the field will rotate away from circuit 1. Amplitude modulation is sometimes referred to as “scan.”175 IFC scan mode might be preferable when the treatment area is large or poorly localized; theoretically, the area of tissue stimulation is larger with modulated amplitude than with fixed amplitude IFC.175 Some IFC machines also allow for on-off cycling. IFC treatment parameters can be manipulated to produce stimulation parameters similar to the different categories of transcutaneous electrical nerve stimulation (TENS) delivery: conventional, acupuncture-like, hyperstimulation, or burst modes.107,108 But Kloth107 notes that a major difference between IFC and TENS might be the ability of IFC to deliver higher currents than those of TENS.
Some have reported that IFC is useful in a variety of musculoskeletal conditions, in neurologic conditions, and in the management of urinary incontinence,∗ although other literature fails to demonstrate its superiority over other interventions or placebo.149,185,191 As with many areas of physical agent modalities, further research is needed to define the role of IFC.163
Precautions and contraindications for IFC are the same as those for other forms of transcutaneous electrical stimulation.74,107 IFC should not be used near implanted stimulators (pacemakers, intrathecal pumps, spinal cord stimulators, etc.) because of the potential for interference with the function of these devices.74,107 One should be aware of the potential for vascular responses when IFC is used near sympathetic ganglia or the carotid sinus.107 IFC should not be used near open incisions or abrasions because of the potential for concentration of electrical current.107 It should not be used near the gravid uterus because of concern about potential adverse effects on fetal development or the potential for stimulating uterine contractions.74,107 In the presence of venous thrombosis, IFC could result in mechanical stimulation of vascular smooth muscle, with the theoretic potential of precipitating emboli.74,107 It should not be used in an insensate area or in a patient cognitively incapable of accurately reporting pain.107 Ford68 reported on a case of full-thickness burn with IFC use near knee arthroplasty. Additionally, IFC should not be used near SWD because of the potential for interference with resultant excessive electrical stimulation.107
1. Abramson D.I., Chu L.S.W., Tuck S., et al. Effect of tissue temperatures and blood flow on motor nerve conduction velocity. JAMA. 1966;198:1082-1088.
2. Abramson D.I., Tuck S., Chu S.W., et al. Effect of paraffin bath and hot fomentations on local tissue temperatures. Arch Phys Med Rehabil. 1964;45:87-94.
3. Al-Kurdi D., Bell-Syer S.E.M., Flemming K. Therapeutic ultrasound for venous leg ulcers. Cochrane Database Syst Rev. (1):2008.
4. Alon G. Interferential current news [letter]. Phys Ther. 1986;66:280-281.
5. [Anonymous]: Nemectrodyn model 7 manual, Karlsruche 1984, Nemectron.
6. Askew L.J., Beckett V.L., An K., et al. Objective evaluation of hand function in scleroderma patients to assess effectiveness of physical therapy. Br J Rheumatol. 1983;22:224-232.
7. Baba-Akbari S.A., Flemming K., Cullum N.A., et al. Therapeutic ultrasound for pressure sores. Cochrane Database Syst Rev. (3):2006.
8. Balmaseda M.T., Fatehi M.T., Koozekanani S.H., et al. Ultrasound therapy: a comparative study of different coupling media. Arch Phys Med Rehabil. 1986;67:147-150.
9. Banga A., Panus P. Clinical applications of ionophoretic devices in rehabilitation medicine. Crit Rev Phys Med Rehabil. 1998;10:147-179.
10. Barber F.A., McGuire D.A., Click S. Continuous-flow cold therapy for outpatient anterior cruciate ligament reconstruction. Arthroscopy. 1998;14(2):130-135.
11. Bare A.C., McAnaw M.B., Pritchard A.E., et al. Phonophoretic delivery of 10% hydrocortisone through the epidermis of humans as determined by serum cortisol concentrations. Phys Ther. 1996;76:738-745.
12. Barlas D., Homan C.S., Thode H.C. In vivo tissue temperature comparison of cryotherapy with and without external compression. Ann Emerg Med. 1996;28:436-439.
13. Basford J.R. The clinical and experimental status of low energy laser therapy. Crit Rev Phys Rehabil Med. 1989;1:1-9.
14. Basford J.R. Low intensity laser therapy: still not an established clinical tool. Lasers Surg Med. 1995;16:331-342.
15. Basford J.R., Malanga G.A., Krause D.A., et al. A randomized controlled evaluation of low-intensity laser therapy: plantar fasciitis. Arch Phys Med Rehabil. 1998;79:249-254.
16. Belanger A. Evidence-based guide to therapeutic physical agents. Philadelphia: Lippincott Williams & Wilkins; 2002.
17. Bell K.R., Lehmann J.F. Effect of cooling on H- and T-reflexes in normal subjects. Arch Phys Med Rehabil. 1987;68:490-493.
18. Bocobo C., Fast A., Kingery W., et al. The effect of ice on intra-articular temperature in the knee of the dog. Am J Phys Med. 1991;70:181-185.
19. Borell R.M., Henley E.J., Ho P., et al. Fluidotherapy: evaluation of a new heat modality. Arch Phys Med Rehabil. 1977;58:69-71.
20. Borell R.M., Parker R., Henley E.J., et al. Comparison of in vivo temperatures produced by hydrotherapy, paraffin wax treatment, and fluidotherapy. Phys Ther. 1980;60:1273-1276.
21. Brosseau L., Casimiro L., Judd M.G., et al. Therapeutic ultrasound for treating patellofemoral pain syndrome. Cochrane Database Syst Rev. (4):2001.
22. Brosseau L., Welch V., Wells G., et al. Low level laser therapy for osteoarthritis and rheumatoid arthritis: a metaanalysis. J Rheumatol. 2000;27:1961-1969.
23. Burke D., Ho C., Saucier M., et al. Effects of hydrotherapy on pressure ulcer healing. Am J Phys Med Rehabil. 1998;77:394-398.
24. Busse J.W., Bhandari M., Kulkarni A.V., et al. The effect of low-intensity pulsed ultrasound therapy on time to fracture healing: a meta-analysis. CMAJ. 2002;166:437-441.
25. Byl N.N. The use of ultrasound as an enhancer for transcutaneous drug delivery: phonophoresis. Phys Ther. 1995;75(6):539-553.
26. Cacchio A., De Blasis E., Desiati P. Effectiveness of treatment of calcific tendinitis of the shoulder by disodium EDTA. Arthritis Rheum. 2009;61:84-91.
27. Cameron M., Monroe L. Relative transmission of ultrasound by media customarily used for phonophoresis. Phys Ther. 1992;72:142-148.
28. Casimiro L., Brosseau L., Welch V., et al. Therapeutic ultrasound for the treatment of rheumatoid arthritis. Cochrane Database Syst Rev. (3):2002.
29. Cetin N., Aytar A., Atalay A., et al. Comparing hot pack, short-wave diathermy, ultrasound, and TENS on isokinetic strength, pain, and functional status of women with osteoarthritic knees: a single-blind, randomized, controlled trial. Am J Phys Med Rehabil. 2008;87:443-451.
30. Chantraine A., Ludy J.P., Berger D. Is cortisone iontophoresis possible? Arch Phys Med Rehabil. 1986;67:38-40.
31. Chiara T., Carlos J., Martin D., et al. Cold effect on oxygen uptake, perceived exertion, and spasticity in patients with multiple sclerosis. Arch Phys Med Rehabil. 1998;79:523-528.
32. Chien Y.W., Banga A.K. Iontophoretic (transdermal) delivery of drugs: overview of historical development. J Pharm Sci. 1989;78:353-354.
33. Chien Y.W., Siddiqui O., Shi W.M., et al. Direct current iontophoretic transdermal delivery of peptide and protein drugs. J Pharm Sci. 1989;78:376-383.
34. Clarke D.H., Stelmach G.E. Muscular fatigue and recovery curve parameters at various temperatures. Res Q. 1966;37:468-479.
35. Clarke R.S., Hellon R.F., Lind A.R. Vascular reactions of the human forearm to cold. Clin Sci. 1958;17:165-179.
36. Coakley W.T. Biophysical effects of ultrasound at therapeutic intensities. Physiotherapy. 1978;64:166-169.
37. Cohn B.T., Draeger R.I., Jackson D.W. The effects of cold therapy in the postoperative management of pain in patients undergoing anterior cruciate ligament reconstruction. Am J Sports Med. 1989;17:344-349.
38. Costello C.T., Jeske A.H. Iontophoresis: application in transdermal medication delivery. Phys Ther. 1995;75(6):554-563.
39. Craig J.A., Barlas P., Baxter G.D., et al. Delayed-onset muscle soreness: lack of effect of combined phototherapy/low-intensity laser therapy at low pulse repetition rates. J Clin Laser Med Surg. 1996;14(6):375-380.
40. Crawford F., Thomson C. Interventions for treating plantar heel pain. Cochrane Database Syst Rev. (1):2003.
41. Dahlstedt L., Samuelson P., Dalen N. Cryotherapy after cruciate knee surgery. Acta Orthop Scand. 1996;67(3):255-257.
42. Daniel D.M., Stone M.L., Arendt D.L. The effect of cold therapy on pain, swelling, and range of motion after anterior cruciate ligament reconstructive surgery. Arthroscopy. 1994;10(5):530-533.
43. Daniels S., Blondel D., Crum L.A., et al. Ultrasonically induced gas bubble production in agar based gels. Part I: experimental investigation. Ultrasound Med Biol. 1987;13:527-539.
44. Darrow H., Schulthies S., Draper D., et al. Serum dexamethasone levels after Decadron phonophoresis. J Athl Train. 1999;34:338-341.
45. DeBie R., deVet H., Lenssen T., et al. Low-level laser therapy in ankle sprains: a randomized clinical trial. Arch Phys Med Rehabil. 1998;79:1415-1420.
46. DeLateur B.J., Lehmann J.F., Stonebridge J.B., et al. Muscle heating in human subjects with 915 MHz microwave contact applicator. Arch Phys Med Rehabil. 1970;51:147-151.
47. Dellhag B., Wollersjo I., Bjelle A. Effect of active hand exercise and wax bath treatments in rheumatoid arthritis patients. Arthritis Care Res. 1992;5:87-92.
48. Demirtas R., Oner C. The treatment of lateral epicondylitis by iontophoresis of sodium salicylate and sodium diclofenac. Clin Rehabil. 1998;12:23-29.
49. Denys E.H. AAEM minimonograph no. 14: the influence of temperature in clinical neurophysiology. Muscle Nerve. 1991;14:795-811.
50. Diller K.R. Analysis of burns caused by long-term exposure to a heating pad. J Burn Care Rehabil. 1991;12:214-217.
51. Docker M., Bazin S., Dyson M., et al. Guidelines for the safe use of continuous shortwave therapy equipment. Physiotherapy. 1992;78:755-757.
52. Dover J.S., Phillips T.J., Arndt K.A. Cutaneous effects and therapeutic uses of heat with emphasis on infrared radiation. J Am Acad Dermatol. 1989;20:278-286.
53. Draper D., Castro J., Feland B., et al. Shortwave diathermy and prolonged stretching increase hamstring flexibility more than prolonged stretching alone. J Orthop Sports Phys Ther. 2004;34:13-20.
54. Draper D., Knight K., Fujiwara T., et al. Temperature change in human muscle during and after pulsed short-wave diathermy. J Orthop Sports Phys Ther. 1999;29:13-18.
55. Drez D., Faust D.C., Evans J.P. Cryotherapy and nerve palsy. Am J Sports Med. 1981;9:256-257.
56. Dumoulin C., Seaborne D.E., Quirion-DeGirardi C., et al. Pelvic-floor rehabilitation, part 1: comparison of two surface electrode placements during stimulation of the pelvic-floor musculature in women who are continent using bipolar interferential currents. Phys Ther. 1995;75:1067-1074.
57. Dyson M. Non-thermal cellular effects of ultrasound. Br J Cancer. 1982;45(suppl):165-171.
58. Dyson M., Woodward B., Pond J.B. Flow of red blood cells stopped by ultrasound. Nature. 1971;232:572-573.
59. Dziedzic K., Hill J., Lewis M., et al. Effectiveness of manual therapy or pulsed shortwave diathermy in addition to advice and exercise for neck disorders: a pragmatic randomized controlled trial in physical therapy clinics. Arthritis Rheum. 2005;53:214-222.
60. Edwards D.J., Rimmer M., Keene G.C.R. The use of cold therapy in the postoperative management of patients undergoing arthroscopic anterior cruciate ligament reconstruction. Am J Sports Med. 1996;24(2):193-195.
61. Einhorn T.A. Current concepts review: enhancement of fracture-healing. J Bone Joint Surg. 1995;77:940-956.
62. Eldred E., Lindsley D.F., Buchwald J.S. The effect of cooling on mammalian muscle spindles. Exp Neurol. 1960;2:144-157.
63. Ennis W.J., Foremann P., Mozen N., et al. Ultrasound therapy for recalcitrant diabetic foot ulcers: results of a randomized, double-blind, controlled, multicenter study. Ostomy Wound Manage. 2005;51:24-39.
64. Ennis W.J., Valdes W., Gainer M., et al. Evaluation of clinical effectiveness of MIST ultrasound therapy for the healing of chronic wounds. Adv Skin Wound Care. 2006;19:437-446.
65. Falconer J., Hayes K.W., Chang R.W. Therapeutic ultrasound in the treatment of musculoskeletal conditions. Arthritis Care Res. 1990;3:85-91.
66. Foley-Nolan D., Barry C., Coughlan R., et al. Pulsed high frequency (27MHz) electromagnetic therapy for persistent neck pain: a double blind, placebo-controlled study of 20 patients. Orthopedics. 1990;13:445-451.
67. Folkow B., Fox R.H., Krog J., et al. Studies on the reactions of the cutaneous vessels to cold exposure. Acta Physiol Scand. 1963;58:342-354.
68. Ford K., Shrader M., Smith J., et al. Full-thickness burn formation after the use of electrical stimulation for rehabilitation of unicompartmental knee arthroplasty. J Arthroplasty. 2005;20:950-953.
69. Franchimont P., Juchmes J., Lecomte J. Hydrotherapy: mechanisms and indications. Pharmacol Ther. 1983;20:79-93.
70. Gam A., Thorsen H., Lonnberg F. The effect of low-level laser therapy on musculoskeletal pain: a meta-analysis. Pain. 1993;52:63-66.
71. Gersten J.W. Effect of metallic objects on temperature rises produced in tissue by ultrasound. Am J Phys Med. 1958;37:75-82.
72. Gnatz S.M. Increased radicular pain due to therapeutic ultrasound applied to the back. Arch Phys Med Rehabil. 1989;70:493-494.
73. Goats G.C. Continuous shortwave (radiofrequency) diathermy. Br J Sports Med. 1989;23:123-127.
74. Goats G.C. Interferential current therapy. Br J Sports Med. 1990;24:87-91.
75. Grant A.E. Massage with ice (cryokinetics) in the treatment of painful conditions of the musculoskeletal system. Arch Phys Med Rehabil. 1964;45:233-238.
76. Green R.J., Laycock J. Objective methods for evaluation of interferential therapy in the treatment of incontinence. IEEE Trans Biomed Eng. 1990;37:615-623.
77. Griffin J.E., Echternach J.L., Price R.E., et al. Patients treated with ultrasonic driven hydrocortisone and with ultrasound alone. Phys Ther. 1967;47:594-601.
78. Griffin X.L., Costello I., Costa M.L. The role of low intensity pulsed ultrasound therapy in the management of acute fractures: a systematic review. J Trauma. 2008;65:1446-1452.
79. Gudeman S., Eisele S., Heidt R., et al. Treatment of plantar fasciitis by iontophoresis of 0.4% dexamethasone: a randomized, double-blind, placebo-controlled study. Am J Sports Med. 1997;25:312-316.
80. Guy A.W., Lehmann J.F., Stonebridge J.B. Therapeutic applications of electromagnetic power. Proc IEEE. 1974;62:55-75.
81. Guyton A.C. Body temperature, temperature regulation, and fever. In Guyton A.C., editor: Textbook of medical physiology, ed 8, Philadelphia: Saunders, 1991.
82. Harris E.D., McCroskery P.A. The influence of temperature and fibril stability on degradation of cartilage collagen by rheumatoid synovial collagenase. N Engl J Med. 1974;290:1-6.
83. Hayes K.W. Heat and cold in the management of rheumatoid arthritis. Arthritis Care Res. 1993;6(3):156-166.
84. Hay-Smith E.J.C. Therapeutic ultrasound for postpartum perineal pain and dyspareunia. Cochrane Database Syst Rev. (3):1998.
85. Hecht P.J., Bachmann S., Booth R.E., et al. Effects of thermal therapy on rehabilitation after total knee arthroplasty. Clin Orthop. 1983;178:198-201.
86. Heckman J.D., Ryaby J.P., McCabe J., et al. Acceleration of tibial fracture-healing by non-invasive, low-intensity pulsed ultrasound. J Bone Joint Surg. 1994;76:26-34.
87. Hedenberg L. Functional improvement of the spastic hemiplegic arm after cooling. Scand J Rehabil Med. 1970;2:154-158.
88. Hekkenberg R.T., Oosterbaan W.A., vanBeekum W.T. Evaluation of ultrasound therapy devices. Physiotherapy. 1986;72:390-394.
89. Henley E. Fluidotherapy. Crit Rev Phys Med Rehabil. 1991;3:173-195.
90. Henley E. Transcutaneous drug delivery: iontophoresis, phonophoresis. Crit Rev Phys Med Rehabil. 1991;2:139-151.
91. Heussler H., Hinchey G., Margiotta E., et al. A double-blind, randomized trial of low-power laser treatment in rheumatoid arthritis. Ann Rheum Dis. 1993;52:703-706.
92. Hocutt J.E., Jaffe R., Rylander C.R., et al. Cryotherapy in ankle sprains. Am J Sports Med. 1982;10:316-319.
93. Holdsworth L., Anderson D. Effectiveness of ultrasound used with a hydrocortisone coupling medium or epicondylitis clasp to treat lateral epicondylitis: pilot study. Physiotherapy. 1993;79:19-25.
94. Hong C- Z. Reversible conduction block in patients with polyneuropathy after ultrasound thermotherapy at therapeutic dosage. Arch Phys Med Rehabil. 1991;72:132-137.
95. Hurley D., Minder P., McDonough S., et al. Interferential therapy electrode placement technique in acute low back pain: a preliminary investigation. Arch Phys Med Rehabil. 2001;82:485-493.
96. Ivey M., Johnston R.V., Uchida T. Cryotherapy for postoperative pain relief following knee arthroplasty. J Arthroplasty. 1994;9(3):285-290.
97. Jan M., Chai H., Wang C., et al. Effects of repetitive shortwave diathermy for reducing synovitis in patients with knee osteoarthritis: an ultrasonographic study. Phys Ther. 2006;86:236-244.
98. Jarit G.J., Mohr K.J., Waller R., et al. The effects of home interferential therapy on post-operative pain, edema, and range of motion of the knee. Clin J Sport Med. 2003;13:16-20.
99. Johnson C.C., Guy A.W. Nonionizing electromagnetic wave effects in biological materials and systems. Proc IEEE. 1972;60:692-718.
100. Jones S.L. Electromagnetic field interference and cardiac pacemakers. Phys Ther. 1976;56:1013-1018.
101. Kantor G. Evaluation and survey of microwave and radiofrequency applicators. J Microw Power. 1981;16:135-150.
102. Kavros S.J., Liedl D.A., Boon A.J. expedited wound healing with noncontact, low-frequency ultrasound therapy in chronic wounds: a retrospective analysis. Adv Skin Wound Care. 2008;21:416-423.
103. Kavros S.J., Miller J.L., Hanna S.W. Treatment of ischemic wounds with noncontact, low-frequency ultrasound: the Mayo Clinic experience, 2004-2006. Adv Skin Wound Care. 2007;20:221-226.
104. Kavros S.J., Schenck E.C. Use of noncontact low-frequency ultrasound in the treatment of chronic foot and leg ulcerations: a 51-patient analysis. J Am Podiatr Med Assoc. 2007;97:95-101.
105. Khan Y., Laurencin C.T. Fracture repair with ultrasound: clinical and cell-based evaluation. J Bone Joint Surg Am. 2008;90(suppl 1):138-144.
106. Klaiman M.D., Shrader J.A., Danoff J.V., et al. Phonophoresis versus ultrasound in the treatment of common musculoskeletal conditions. Med Sci Sports Exerc. 1998;30:1349-1355.
107. Kloth L.C. Interference current. In Nelson R.M., Currier D.P., editors: Clinical electrotherapy, ed 2, Norwalk, CT: Appleton & Lange, 1991.
108. Kloth L.C. Electrotherapeutic alternatives for the treatment of pain. In: Gersh M.R., editor. Electrotherapy in rehabilitation. Philadelphia: FA Davis, 1992.
109. Knutsson E., Mattsson E. Effects of local cooling on monosynaptic reflexes in man. Scand J Rehabil Med. 1969;1:126-132.
110. Kotke F.J., Lehmann J.F., editors. Krusen’s handbook of physical medicine and rehabilitation, ed 4. Philadelphia: Saunder, 1990:293.
111. Kristiansen T.K., Ryaby J.P., McCabe J., et al. Accelerated healing of distal radial fractures with the use of specific, low-intensity ultrasound. J Bone Joint Surg. 1997;79:961-973.
112. Lehmann J.F. Therapeutic heat and cold, ed 4. Baltimore: Williams & Wilkins; 1990.
113. Lehmann J.F., Brunner G.D., Stow R.W. Pain threshold measurements after therapeutic application of ultrasound, microwaves and infrared. Arch Phys Med Rehabil. 1958;39:560-565.
114. Lehmann J.F., DeLateur B.J., Stonebridge J.B. Selective muscle heating by shortwave diathermy with a helical coil. Arch Phys Med Rehabil. 1969;50:117-123.
115. Lehmann J.F., DeLateur B.J., Warren C.G., et al. Heating produced by ultrasound in bone and soft tissue. Arch Phys Med Rehabil. 1967;48:397-401.
116. Lehmann J.F., DeLateur B.J., Warren C.G., et al. Therapeutic temperature distribution produced by ultrasound as modified by dosage and volume of tissue exposed. Arch Phys Med Rehabil. 1967;48:662-666.
117. Lehmann J.F., DeLateur B.J., Warren C.G., et al. Heating of joint structures by ultrasound. Arch Phys Med Rehabil. 1968;49:28-30.
118. Lehmann J.F., Johnston V.C., McMillan J.A., et al. Comparison of deep heating by microwaves at frequencies 2456 and 900 megacycles. Arch Phys Med Rehabil. 1965;46:307-314.
119. Lehmann J.F., Masock A.J., Warren C.G., et al. Effect of therapeutic temperatures on tendon extensibility. Arch Phys Med Rehabil. 1970;51:481-487.
120. Lehmann J.F., McDougall J.A., Guy A.W., et al. Heating patterns produced by shortwave diathermy applicators in tissue substitute models. Arch Phys Med Rehabil. 1983;64:575-577.
121. Lehmann J.F., McMillan J.A., Brunner G.D., et al. Comparative study of the efficiency of short-wave, microwave and ultrasonic diathermy in heating the hip joint. Arch Phys Med Rehabil. 1959;40:510-512.
122. Lehmann J.F., Silverman D.R., Baum B.A., et al. Temperature distributions in the human thigh, produced by infrared, hot pack and microwave applications. Arch Phys Med Rehabil. 1966;47:291-299.
123. Lekas M.D. Iontophoresis treatment. Otolaryngol Head Neck Surg. 1979;87:292-298.
124. Leutz D.W., Harris H. Continuous cold therapy in total knee arthroplasty. Am J Knee Surg. 1995;8:121-123.
125. Levenson J.L., Weissberg M.P. Ultrasound abuse: case report. Arch Phys Med Rehabil. 1983;64:90-91.
126. Levy A.S., Marmar E. The role of cold compressive dressings in the postoperative treatment of total knee arthroplasty. Clin Orthop Relat Res. 1993;297:174-178.
127. Lewis T. Observations upon the reactions of the vessels of the human skin to cold. Heart. 1930;15:177-208.
128. Li L.C., Scudds R.A. Iontophoresis: an overview of the mechanisms and clinical application. Arthritis Care Res. 1995;8(1):51-61.
129. Lowdon B.J., Moore R.J. Determinants and nature of intramuscular temperature changes during cold therapy. Am J Phys Med. 1975;54:223-233.
130. Marks R., Ghanagaraja S., Ghassemi M. Ultrasound of osteo-arthritis of the knee: a systematic review. Physiotherapy. 2000;86:452-463.
131. Marks R., Ghassemi M., Duarte R., et al. A review of the literature on shortwave diathermy as applied to osteo-arthritis of the knee. Physiotherapy. 1999;85:304-316.
132. McGown H.L. Effects of cold application on maximal isometric contraction. Phys Ther. 1967;47:185-192.
133. McLeod D.R., Fowlow S.B. Multiple malformations and exposure to therapeutic ultrasound during organogenesis. Am J Med Genet. 1989;34:317-319.
134. Meeusen R., Lievens P. The use of cryotherapy in sports injuries. Sports Med. 1986;3:398-414.
135. Melzack R., Jeans M.E., Stratford J.G., et al. Ice massage and transcutaneous electrical stimulation: comparison of treatment for low-back pain. Pain. 1980;9:209-217.
136. Mense S. Effects of temperature on the discharge of muscle spindles and tendon organs. Pflugers Arch. 1978;374:159-166.
137. Miglietta O. Action of cold on spasticity. Am J Phys Med. 1973;52:198-205.
138. Miller D.L. A review of the ultrasonic bioeffects of microsonation, gas-body activation, and related cavitation-like phenomena. Ultrasound Med Biol. 1987;13:443-470.
139. Mitchell S., Trowbridge C., Fincher A., et al. Effect of diathermy on muscle temperature, electromyography, and mechanomyography. Muscle Nerve. 2008;38:992-1004.
140. Mitrogotri S., Kost J. Low-frequency sonophoresis: a review. Adv Drug Deliv Rev. 2004;56:589-601.
141. Moffett J., Richardson P., Frost H., et al. A placebo controlled double blind trial to evaluate the effectiveness of pulsed short wave therapy for osteoarthritic hip and knee pain. Pain. 1996;67:121-127.
142. Montorsi F., Salonia A., Guazzoni G., et al. Transdermal electromotive multi-drug administration for Peyronie’s disease: preliminary results. J Androl. 2000;21:85-90.
143. Morison W.L. Phototherapy and photochemotherapy. Adv Dermatol. 1992;7:255-271.
144. Newman J.T., Nellermoe M.D., Carnett J.L. Hydrocortisone phonophoresis. J Am Podiatr Med Assoc. 1992;82:432-435.
145. Nolte P.A., Van Der Krans A., Patka P., et al. Low-intensity pulsed ultrasound in the treatment of nonunions. J Trauma. 2001;51:693-703.
146. Nukada H., Pollock M., Allpress S. Experimental cold injury to peripheral nerve. Brain. 1981;104:779-811.
147. Nutt J., Anderson V., Peacock J., et al. DBS and diathermy interaction induces severe CNS damage. Neurology. 2001;56:1384-1386.
148. Oakley E.M. Dangers and contraindications of therapeutic ultrasound. Physiotherapy. 1978;64:173-174.
149. Olah K.S., Bridges N., Denning J., et al. The conservative management of patients with symptoms of stress incontinence: a randomized, prospective study comparing weighted vaginal cones and interferential therapy. Am J Obstet Gynecol. 1990;162:87-92.
150. O’Malley E.P., Oester Y.T. Influence of some physical chemical factors on iontophoresis using radio-isotopes. Arch Phys Med Rehabil. 1955;36:310-316.
151. Oosterveld F.G.J., Rasker J.J. Treating arthritis with locally applied heat or cold. Semin Arthritis Rheum. 1994;24(2):82-90.
152. Ozawa A., Haruki Y., Iwashita K., et al. Follow-up of clinical efficacy of iontophoresis therapy for postherpetic neuralgia (PHN). J Dermatol. 1999;26:1-10.
153. Paliwal B.R., Shrivastava P.N. Microwave hyperthermia: principles and quality assurance. Radiol Clin North Am. 1989;27:489-497.
154. Penderghest C., Kimura I., Gulick D. Double-blind clinical efficacy study of pulsed phonophoresis on perceived pain associated with symptomatic tendinitis. J Sport Rehabil. 1998;7:9-19.
155. Perez C.A., Emami B. Clinical trials with local (external and interstitial) irradiation and hyperthermia: current and future perspectives. Radiol Clin North Am. 1989;27:525-542.
156. Perkins J.F., Li M., Nicholas C.H., et al. Cooling as a stimulus to smooth muscles. Am J Physiol. 1950;163:14-26.
157. Perron M., Malouin F. Acetic acid iontophoresis and ultrasound for the treatment of calcifying tendinitis of the shoulder: a randomized control trial. Arch Phys Med Rehabil. 1997;78:379-384.
158. Petajan J.H., Watts N. Effects of cooling on the triceps surae reflex. Am J Phys Med. 1962;41:240-251.
159. Philadelphia Panel. Philadelphia Panel evidence-based clinical practice guidelines on selected rehabilitation interventions for low back pain. Phys Ther. 2001;81:1641-1674.
160. Philadelphia Panel. Philadelphia Panel evidence-based clinical practice guidelines on selected rehabilitation interventions for knee pain. Phys Ther. 2001;81:1675-1700.
161. Philadelphia Panel. Philadelphia Panel evidence-based clinical practice guidelines on selected rehabilitation interventions for neck pain. Phys Ther. 2001;81:1701-1717.
162. Philadelphia Panel. Philadelphia Panel evidence-based clinical practice guidelines on selected rehabilitation interventions for shoulder pain. Phys Ther. 2001;81:1719-1730.
163. Poitras S., Brosseau L. Evidence-informed management of chronic low back pain with transcutaneous electrical nerve stimulation, interferential current, electrical muscle stimulation, ultrasound, and thermotherapy. Spine J. 2008;8:226-233.
164. Price R., Lehmann J.F., Boswell-Bessette S., et al. Influence of cryotherapy on spasticity at the human ankle. Arch Phys Med Rehabil. 1993;74:300-304.
165. Rattanachaiyanont M., Kuptniratsaikul V. No additional benefit of shortwave diathermy over exercise program for knee osteoarthritis in peri-/post-menopausal women: an equivalence trial. Osteoarthritis Cartilage. 2008;16:823-828.
166. Riedl C., Plas E., Engelhardt P., et al. Iontophoresis for treatment of Peyronie’s disease. J Urol. 2000;163:95-99.
167. Ritzmann S.E., Levin W.C. Cryopathies: a review. Arch Intern Med. 1961;107:186-204.
168. Robertson V., Ward A., Jung P. The effect of heat on tissue extensibility: a comparison of deep and superficial heating. Arch Phys Med Rehabil. 2005;86:819-825.
169. Rosim G., Barieri C., Lancas F., et al. Diclofenac phonophoresis in human volunteers. Ultrasound Med Biol. 2005;31:337-343.
170. Scarcella J.B., Cohn B.T. The effect of cold therapy on the postoperative course of total hip and knee arthroplasty patients. Am J Orthop. 1995;24(11):847-852.
171. Scheffler N., Sheitel P., Lipton M. Use of Cryo/Cuff for the control of postoperative pain and edema. J Foot Surg. 1992;31:141-146.
172. Schindl A., Schindl M., Pernerstofer-Schon H., et al. Low-intensity laser therapy: a review. J Invest Med. 2000;48:312-326.
173. Schmidt K.L., Ott V.R., Rocher G., et al. Heat, cold and inflammation [review]. Z Rheumatol. 1979;38:391-404.
174. Scott B.O. Effects of contact lenses on short-wave field distribution. Br J Ophthalmol. 1956;40:696-697.
175. Selkowitz D.M. Electrical currents. In: Cameron M.H., editor. Physical agents in rehabilitation: from research to practice. Philadelphia: Saunders, 1999.
176. Shafshak T.S., El-Sheshai A.M., Soltan H.E. Personality traits in the mechanisms of interferential therapy for osteoarthritic knee pain. Arch Phys Med Rehabil. 1991;72:579-581.
177. Shah S., Farrow A. Investigation of practices and procedures in the use of therapeutic diathermy: a study from the physiotherapists’ health and safety perspective. Physiother Res Int. 2007;12:228-241.
178. Shepherd J.T., Rusch N.J., Vanhoutte P.M. Effect of cold on the blood vessel wall. Gen Pharmacol. 1983;14:61-64.
179. Shields N., Gormley J., O’Hare N. Short-wave diathermy: current clinical and safety practices. Physiother Res Int. 2002;7:191-202.
180. Shrivastava S.N., Singh G. Tap water iontophoresis in palmo-plantar hyperhidrosis. Br J Dermatol. 1977;96:189-195.
181. Shulman A.G. Ice water as primary treatment of burns. JAMA. 1960;173:96-99.
182. Stewart H.F., Harris G.R., Herman B.A., et al. Survey of use and performance of ultrasonic therapy equipment in Pinellas County, Florida. Phys Ther. 1974;54:707-714.
183. Stolman L. Treatment of excess sweating of the palms by iontophoresis. Arch Dermatol. 1987;123:893-896.
184. Stolman L. Treatment of hyperhydrosis. Dermatol Clin. 1998;16:863-869.
185. Taylor K., Newton R.A., Personius W.J., et al. Effects of interferential current stimulation for treatment of subjects with recurrent jaw pain. Phys Ther. 1987;67:346-350.
186. Ter Haar G., Dyson M., Oakley E.M. The use of ultrasound by physiotherapists in Britain, 1985. Ultrasound Med Biol. 1987;13:659-663.
187. Thomson P.D., Bowden M.L., McDonald K., et al. A survey of burn hydrotherapy in the United States. J Burn Care Rehabil. 1990;11:151-155.
188. Thornton K.L. Principles of ultrasound. J Reprod Med. 1992;37:27-32.
189. Travell J. Ethyl chloride spray for painful muscle spasm. Arch Phys Med. 1952;33:291-298.
190. Tredget E.E., Shankowsky H.A., Joffe A.M., et al. Epidemiology of infections with Pseudomonas aeruginosa in burn patients: the role of hydrotherapy. Clin Infect Dis. 1992;15:941-949.
191. Van der Heijden G., Leffers P., Wolters P., et al. No effect of bipolar interferential electrotherapy and pulsed ultrasound for soft tissue disorders: a randomised controlled trial. Ann Rheum Dis. 1999;58:530-540.
192. Van der Windt D.A.W.M., van der Heijden G.J.M.G., van den Berg S.G.M., et al. Ultrasound therapy for musculoskeletal disorders: a systematic review. Pain. 1999;81:257-271.
193. Van der Windt D.A.W.M., van der Heijden G.J.M.G., van den Berg S.G.M., et al. Therapeutic ultrasound for acute ankle sprains. Cochrane Database Syst Rev. 2002;(1):CD001250.
194. Walk E.E., Himel H.N., Batra E.K., et al. Aquatic access for the disabled. J Burn Care Rehabil. 1992;13:356-363.
195. Warren C.G., Koblanski J.N., Sigelmann R.A. Ultrasound coupling media: their relative transmissivity. Arch Phys Med Rehabil. 1976;57:218-222.
196. Watson C.W. Effect of lowering of body temperature on the symptoms and signs of multiple sclerosis. N Engl J Med. 1959;261:1253-1259.
197. Waylonis G.W. The physiologic effects of ice massage. Arch Phys Med Rehabil. 1967;48:37-42.
198. Webb J.M., Williams D., Ivory J.P., et al. The use of cold compression dressing after total knee replacement: a randomized controlled trial. Orthopedics. 1998;21(1):59-61.
199. Weinberger A., Fadilah R., Lev A., et al. Intra-articular temperature measurements after superficial heating. Scand J Rehabil Med. 1989;21:55-57.
200. Welch V., Brosseau L., Casimiro L., et al. Thermotherapy for treating rheumatoid arthritis. Cochrane Database Syst Rev. (2):2002.
201. Welch V., Brosseau L., Peterson J., et al. Therapeutic ultrasound for osteoarthritis of the knee. Cochrane Database Syst Rev. (3):2001.
202. Werners R., Pynsent P.B., Bulstrode C.J. Randomized trial comparing interferential therapy with motorized lumbar traction and massage in the management of low back pain in a primary care setting. Spine. 1999;24:1579-1584.
203. Whitelaw G.P., DeMuth K.A., Demos H.A., et al. The use of the Cryo/Cuff versus ice and elastic wrap in the postoperative care of knee arthroscopy patients. Am J Knee Surg. 1995;8(1):28-31.
204. Woodmansey A., Collins D.H., Ernst M.M. Vascular reactions to the contrast bath in health and in rheumatoid arthritis. Lancet. 1938;2:1350-1353.
205. Wright V., Johns R.J. Quantitative and qualitative analysis of joint stiffness in normal subjects and in patients with connective tissue diseases. Ann Rheum Dis. 1961;20:36-45.
206. Yarrobino T., Kalbfleisch J., Ferslew K., et al. Lidocaine iontophoresis mediates analgesia in lateral epicondylalgia treatment. Phyisother Res Int. 2006;11:152-160.
207. Yousefi-Nooraie R., Schonstein E., Heidari K., et al. Low level laser therapy for nonspecific low-back pain. Cochrane Database Syst Rev. (2):2007.
208. Ziskin M.C. Fundamental physics of ultrasound and its propagation in tissue. Radiographics. 1993;13:705-709.
∗ References 21, 28, 84, 130, 192, 193.
† References 24, 61, 78, 86, 105, 111, 120, 145.
∗ References 29, 53, 59, 66, 73, 97, 131, 141, 165.
∗ References 10, 37, 42, 60, 85, 96, 124, 126, 170, 198, 203.
∗ References 9, 30, 38, 40, 48, 79, 90, 128, 142, 152, 157, 166, 180, 183, 184, 206.
† References 11, 25, 26, 44, 90, 93, 106, 154, 169.