51 Pediatric Equipment
Heating and Cooling Systems
The operating room (OR), which is comparable to a large infant incubator, provides the physical environment for the conduct of anesthesia. Readily controlled heating and cooling systems are crucial for thermal stability of the child, that is, control of the external environment. Neonates and small infants require a warmer room temperature than adults; they should be considered poikilothermic, particularly if they are preterm, critically ill, or stressed. With approximately 40% of heat loss by radiation and 35% by convection, heating the OR temperature warms the walls, which decreases radiation heat loss and warms the air, which decreases convection heat loss (see Chapters 35 and 36). Exposure to a cool room temperature during induction of anesthesia and surgical preparation may cause significant thermal stress, particularly in the neonate and young infant. Mild to moderate hypothermia may cause acidosis and apnea in infants, alter the pharmacokinetics of medications, cause difficulty in antagonizing the neuromuscular blockade, and increase oxygen (O2) consumption with shivering.1 Once the child is prepared and draped, the OR temperature may be reduced to a more comfortable level.
Warming Devices
Radiant Warmers
Overhead radiant heating units with servomechanism temperature control were useful to maintain the temperature of small neonates and infants in the past, but these have been replaced with other strategies. Given the risk of skin burn, the servomechanism control sensor must be applied to the warmed skin and should not measure body core temperature.1 A maximum skin temperature of 98.6° F (37° C) should preclude surface burns, although the risk persists in poorly perfused skin. The radiant warmer may be used during induction and surgical preparation; it may be used again as the drapes are removed. If infrared light bulbs are used, care must be taken to ensure that the heat lamp is an appropriate distance from the child to avoid thermal injury2; note that the red cast from the light may make evaluation of a child’s color difficult.
Warming Blankets
Circulating water mattresses help maintain normothermia in children with body surface areas of 0.5 m2 or less (approximately 10 kg).3 For most children, conductive heat loss accounts for less than 5% of total body heat loss; therefore these devices have limited usefulness in maintaining thermal homeostasis and virtually no benefit for children weighing more than 10 kg. To avoid surface burns, the fluid temperature should never exceed 102.2° F (39° C) and must be monitored4; several layers of material should be interposed between the child and the warming blanket to avoid direct contact. In many institutions these have been replaced by forced air warming mattresses.
Forced Warm Air Devices
The most useful device for maintaining thermal homeostasis is the warm air mattress that can be wrapped around the head and upper or lower torso.5–9 These devices rely on the combination of convection with warm air and plastic wrap that also serves to reduce evaporative heat losses. They are the single most effective means for warming children, even when only a portion of the body is covered.5,10 One report suggests that simple delivery of warm air beneath bed sheets without the use of a plastic blanket can be effective,10 but significant thermal injuries have been reported without the use of a licensed blanket or when used in children with poor skin perfusion.6 Therefore unlicensed use of these devices is not recommended. In extreme cases of hypothermia (cardiac surgery or liver transplant), a blanket can be placed both beneath and on top of the child to speed the warming process. Two concerns have been raised regarding these devices. First, concern has been expressed that these devices may incubate infections and distribute them into the OR. The standard design and design changes in the hardware may, unbeknownst to the operator, increase the infectious risk from some warmers.7–9 Concern also exists that under laminar airflow conditions for joint replacement, these devices increase the infectious risk.11 Also, the cost of these disposable air mattresses may be a considerable expense.
Passive Heat and Moisturizer Exchangers, “Artificial Nose”
Heat moisture exchangers are effective in preserving body heat, but ineffective to raise the child’s body temperature.12–14 In infants and children, these devices add humidity to the circuit15 and may also function as filters for infectious pathogens and reduce the transmission of infectious agents between children.16,17 These devices may increase the resistance to breathing during spontaneous ventilation and completely obstruct the circuit with prolonged use.18–20
Fluid and Blood Warmers
The effectiveness of standard fluid warmers depends on the time that the intravenous fluids or blood products are in contact with the warmer. Excessive heating may cause hemolysis of red blood cells.21 With slow intravenous fluid therapy, the fluid warmer does not contribute significant heat transfer because heat is lost along the intravenous tubing between the warmer and the child, before the fluid enters the child’s body. Warming intravenous fluid during maintenance therapy is virtually useless for temperature regulation.
Blood warmers vary from simple coiled tubing in a warming bath to the more sophisticated rapid transfusion devices. Rapid transfusion systems are vitally important in any institution that manages trauma patients or performs major surgical procedures. The new generation of warming devices is far superior to waterbath warmers. They use countercurrent heat exchange or microwave technology as a means of rapidly warming fluid along the length of the column of fluid. The choice of device depends on the size of the child, the anticipated rate of blood loss, and cost. The length of the intravenous tubing and the diameter of the intravenous catheter also limit the rate of rapid infusion. An example of these devices with the lowest capacity is the Hot Line (Smiths Medical ASD, Rockland, Mass.), which is extremely effective at rates up to 75 mL/min.22 The manufacturer’s data suggest that approximately 90 mL/min of refrigerated blood products can be warmed to 95° F (35° C) with this device. Another device that has a low volume (6 mL priming volume) and is inserted within the intravenous system just before the intravenous catheter is the Belmont Buddy fluid warmer (Belmont Instrument Corp., Billerica, Mass.). The manufacturer claims a warming capacity of cold fluids to 100.4° F (38° C) at flow rates as low as “keep vein open” (KVO) and as great as 100 mL/min.23 This device should be inserted close to the intravenous catheter because it may help maintain the child’s temperature by minimizing heat loss along the length of the intravenous tubing. A more complex and larger capacity system is the Level 1 System 1000 (Smiths Medical ASD, Rockland, Mass.), which uses countercurrent warming technology combined with the capacity to infuse fluid, blood, or plasma in bags under pressure. Because this device infuses bags of fluid under pressure, it is important to eliminate all air from the bags to avoid the possibility of air embolization. This system is capable of warming fluids at rates up to approximately 500 mL/min.24 The Level 1 device is capable of warming blood starting at 41° F (5° C) to 42.8° F (6° C) to at least 91.4° F (33° C) at rates as high as 250 mL/min.25 The manufacturer’s data suggest that up to 600 mL/min of red cells diluted to a hematocrit of 60% can be delivered through a 14-gauge intravenous catheter and 750 mL/min through an 8.5F introducer sheath. The addition of an air detection device with automatic flow interruption has improved safety.26 Another high-capacity system is the Belmont FMS warmer (Belmont Instrument Corp, Billerica, Mass.) uses microwave warming and is capable of fluid delivery at rates of 10 to 750 mL/min; this device has two air detectors and an in-line pressure detector. One study compared the flow rates and warming capabilities of the Level 1 system with the Rapid Infusion System (no longer manufactured but equivalent to two Belmont FMS systems); both devices were comparable when used with 18- or 20-gauge intravenous catheters in terms of warming and flow rates. However, for any catheter larger than 18 gauge, the Rapid Infusion System was superior for both flow and fluid warming, particularly for flows greater than 200 mL/min (Figs. 51-1 and 51-2).27,28 A similar comparison has been made with the Level 1 and the Belmont FMS systems, with a similar difference in terms of better warming capacity during high flows.29
In general, the Level 1 Hotline (Smiths Medical) or the Belmont Buddy appear most suited for low-volume, relatively slow blood loss. The Level 1 system seems most appropriate for children weighing 40 kg or less with expected rapid blood loss and the Belmont FMS for larger children with expected massive and rapid blood loss. The advantages and disadvantages of these warming devices are compared in Table 51-1.30
Heated Humidifiers
Airstream warming and humidification devices are useful for maintaining body temperature.31–33 If a humidifier is used, the anesthesiologist should be familiar with the potential hazards: overheating, leaks, “rain out,” changes in compression volume, and obstruction if connected in reverse. Humidifiers with servomechanism-controlled temperature regulation may prevent overheating and overhydration of infants. Anesthesia breathing circuits with internal heating elements may further reduce the rainout problem and minimize the risk of overheating; these advantages must be weighed against the additional expense. However, with the advent of warm air mattresses and more common use of circle systems in children of all sizes, there appears to be less of a need for in-line humidification as a means of warming children. The main indication for in-line humidification is to prevent secretions from drying when nonrebreathing circuits are used.
Wrapping
Any form of wrapping can reduce radiant and convective heat losses; plastic wrap used for food is particularly effective and inexpensive. Covering an infant’s head is of greatest value because the head represents such a large proportion of the body surface area. As with any warming device, there are hazards; the plastic drapes reduce conductive losses but also eliminate sweating as a mechanism of thermal regulation, so that hyperthermia may result.34 “Space” blankets with reflective aluminized Mylar layers have been very effective in preventing heat loss in the past.35 With effective room temperature control and forced air heating mattresses, the importance of wrapping the child has diminished.
Intravenous Therapy
The volume of the infusion fluid container should not exceed the child’s estimated fluid deficit unless a volume-limiting device is interposed between the intravenous container and the child. Generally, an intravenous set with a microdrop outlet (60 drops/mL) is most practical for children weighing less than 50 kg. Infusion sets that include a one-way valve to prevent retrograde flow in the intravenous administration set are most useful. The infusion setup should include easily accessible injection ports; intravenous extension tubing may be required if the access is in the lower extremities. A three-way stopcock within the system is also helpful should an “adult” intravenous line be needed to give a rapid bolus of intravenous fluid. Recent practice has shifted from needle injection ports to needleless. Both the needleless ports and stopcocks are reservoirs for small volumes of air that may be injected into the intravenous tubing if the air is not cleared during the initial setup or aspirated into the medication syringe before delivering the syringe contents. It is best to purge infusion sets of all air bubbles, especially at each injection port, before use with infants and children.36 In children with known cardiac defects with shunts or arteriovenous malformations, and in infants with patent foramen ovale, the potential for paradoxical air emboli is always present (up to 27% of adults).37 In these circumstances, intravenous air traps may reduce this risk, but caution is advised because blood cannot pass through such devices.38 In the smallest patients, a T-connector placed at the hub of the intravenous catheter allows direct injection of drugs with minimal fluid dead space. It should also be noted that stopcocks and injection ports may act as a reservoir for administered drugs; it is thus important to always flush through any medications administered with these systems. With standard injection ports, long needles will bypass the injection port and deliver drug into the main body of the intravenous tubing (see later).
The flow of crystalloid is not a simple linear function of perfusion pressure; the relationship deviates substantially from linearity. Additionally, elements of the intravenous system have isolated effects on one factor or another. For example, a 5-µm filter increases resistance and reduces flow at all pressures. A check valve significantly increases resistance but reduces flow primarily at high flow rates.39 A similar nonlinear function describes the pressure and flow relationship of intravenous cannulas.40 Studies of crystalloid solutions demonstrate steep increases in resistance with increasing pressure in a nonlinear manner with smaller-gauge cannulas (E-Fig. 51-1). The effects of the tubing set add to the effects of the cannula; connecting large-diameter tubing to a smaller-diameter catheter limits flow according to the diameter of the catheter. In situations in which massive and rapid blood loss is anticipated, insertion of extra-large peripheral intravenous catheters such as 5, 6, 7 or 8F pulmonary artery flow catheter introducers or specially designed rapid infusion catheters will provide the most efficient method for rapid volume administration. Special placement kits are available that allow the dilation of small veins by the placement of a guidewire through either a small needle (Seldinger technique) or a small-gauge intravenous line followed by dilation of the vein and placement of a larger-diameter cannula (see Chapter 48).
Accelerating flow through an intravenous line is a concern in emergency situations. Rapid transfusion devices are the best method for increasing flow rates (see earlier).22,24,25 For bags of crystalloid, colloid, or blood components a pressure infusion cuff is a relatively fast, readily available, and the least expensive method for rapidly administering large volumes.41 It is important to remove air from these bags so as to avoid air embolization because an air detector is not used with this technique. Next in order of efficacy are in-line blood pumps or a stopcock and syringe arrangement; gravity is the slowest method of infusion. The syringe technique is often used in infants and children (primarily for accurate measurement in infants); it is similar in efficiency to the in-line blood pump. Intravenous tubing designed for administering blood has better flow characteristics than standard intravenous tubing.42 Large-bore “resuscitation” tubing provides the best flow characteristics; however, the final common pathway, the lumen of the intravenous catheter itself, is the major limitation of flow capacity.25,39–44 Larger-diameter, short catheters allow greater flow than long narrow-diameter catheters.44 This factor is particularly important when rapid volume administration is required but the only intravenous access is through a central venous catheter. This problem is greatly magnified when multilumen catheters are used.44–45 If rapid volume resuscitation is a significant potential problem, then one single-lumen, large-bore peripheral intravenous line is of much greater value than a multilumen central venous catheter. Peripherally inserted central catheters (PICC) lines are a potential problem as well because of their very large resistance they cannot be relied on for rapid volume administration (see further discussion of laminar and turbulent flow).
The choice of fluid and its temperature also have effects on the rate of infusion, primarily because of differences in viscosity. Crystalloid passes most readily, followed by colloid, whole blood, and packed red blood cells. Dilution of packed red blood cells with normal saline markedly improves the flow rate and decreases hemolysis during rapid infusion.46 Hemolysis and associated hyperkalemia of packed red blood cells transfused through short or long catheters under high pressure appears to not be clinically important when the rate of transfusion is moderate. However, rapid transfusion may be associated with hyperkalemia, particularly in infants.47–51
One further concern that has very important clinical implications is the interaction among intravenous tubing design, infusion pumps, and carrier flow rates regarding timing of actual drug delivery to the child. This is an even greater issue in infants and neonates in whom the volumes of drugs and the carrier infusion rates are small. Several studies of adult equipment have examined the interactions of dead space volumes and carrier rates on the time lag to initial drug delivery, time to steady-state drug delivery, and then offset once the infusion is stopped. These interactions also vary whenever a change in carrier flow rate occurs (e.g., when a slow carrier infusion is suddenly increased, this will result in a bolus of drug). Conversely, when a rapid carrier rate is suddenly reduced, this will result in a marked reduction in drug delivery. The importance of these interactions increases the further upstream in the system that a drug is administered; for example, the administration of a drug directly into the T-connector will be the least susceptible to these variables than a drug administered into a side port of an intravenous line 10 to 30 cm away from the intravenous catheter. Thus, when life-support medications are being administered, it is vital to avoid fluctuations or interruptions in carrier rate and to reduce as much as possible the dead space in the system between the child’s bloodstream and the point of drug entering the intravenous system. This is true for the OR, during transit to the intensive care unit (ICU), and at the time of transfer of care. This is particularly an issue when OR pumps and intravenous equipment are switched over to new tubing and pumps in the ICU. Figure 51-3 illustrates the time delay in drug delivery comparing the dead space of an injection port with and without a needle that bypasses the dead space of the injection port and how this time delay can be minimized by “priming” the dead space of the injection port.52,53 Figure 51-4 illustrates several multidrug administration manifolds that allow administration of many medications simultaneously, each with differing dead space characteristics that could cause considerable delay in drug delivery if the dead space within each is inadequately primed. The best way to avoid this problem is to flush each drug through the system from entry to exit point, shut off the infusion, flush the carrier through the system to remove any medications from the carrier portion of the manifold, then attach the system to the patient, so that all infusions will immediately be discharged into the carrier intravenous fluid when turned on, rather than having a delay while the dead space at the connection is filled.
Airway Apparatus
Masks
The importance of elimination of mechanical dead space increases with decreasing size of the child. Rendell-Baker/Soucek masks, developed from molds of the facial contours of Caucasian children, were designed to minimize mechanical dead space without the inflatable cuff or high dome of adult masks. Transparent disposable plastic models are preferable to the classic black conductive rubber because they allow observation of a child’s color and condensate from exhaled humidity with respirations. Plastic disposable masks with soft inflatable cuffs around the periphery of the mask are particularly well suited for children with anatomic or mechanical problems that interfere with normal mask application. An especially useful mask has a built-in port, which allows passage of an endotracheal tube over a fiberoptic scope, thus providing excellent fiberoptic intubation conditions while maintaining spontaneous respiration, oxygenation, and depth of anesthesia (see E-Fig. 12-13).54
Oral Airways
A complete selection of oral airways must be readily available. Infants have a relatively large tongue, which easily obstructs the airway once they lose consciousness. If too large an airway is inserted, damage to laryngeal structures (traumatic epiglottitis, uvular swelling) may result in postoperative airway obstruction (see Fig. 12-13).55 Improperly inserted airways, by obstructing venous and lymphatic drainage, also may result in airway obstruction secondary to swelling of the tongue.56,57 A tongue blade is useful to help insert the airway without kinking the tongue or catching the tongue or lips between the airway and the teeth.
Laryngeal Mask Airway
The laryngeal mask airway (LMA) has proved to be a great addition to the airway management of children. Minimal practice is required to develop the skills for insertion. In some circumstances the LMA can be lifesaving (see Chapter 12).58–64 This device generally provides a clear airway for maintaining spontaneous respirations; however, it cannot be relied on if controlled ventilation is required. Controlled ventilation has been described but holds the risk of gastric dilation.65 We think that this device should be used primarily for spontaneous ventilation and with great caution with controlled ventilation in infants and children. Modern anesthesia ventilators provide the alternative and likely safer pressure-assisted mode of ventilation.66 The LMA is particularly useful in children with anatomic airway abnormalities, because it provides a clear airway while other measures are planned for successful tracheal intubation or a tracheotomy.64,67 Fiberoptic intubation through the LMA is possible in children with midfacial hypoplasia syndromes and in those with redundant airway tissue such as mucopolysaccharidoses (see also E-Fig. 12-22, A through C). Fiberoptic intubation is easier through the LMA because the child can be well oxygenated while using the fiberoptic scope to find the laryngeal inlet. Emergency placement of this device can also be lifesaving in children who are difficult to ventilate with bag and mask with or without a nasal or oral airway.68
Variations to the classic LMA include disposable LMAs of all sizes, intubating LMAs in larger sizes, LMAs with reinforced tubing, and the ProSeal LMA (LMA, La Jolla, Calif.) with a distal opening that provides the ability to pass an orogastric tube (see Fig. 12-18, A and B). This variation to the classic LMA produces a better laryngeal seal and provides the ability to ventilate the lungs without a significant leak at greater peak inflation pressures.69 This variation would seem to offer the greatest advantage in the child with a known difficult airway or in the emergency management of the child with a difficult airway who also has a full stomach. Many other LMA variants are available, each with claimed advantages. Each practitioner should assess which device is most effective in the practice environment70 (see also Chapter 12 for a more in-depth discussion).
Tracheal Tubes
A variety of tracheal tubes must be available with appropriate sizes of stylets. There may be considerable variations from one manufacturer to another in wall thickness, external diameter, kink resistance, and direction and angle of bevel, as well as differences in tracheal tube cuff length and thickness of cuffed tracheal tubes.71 All tracheal tubes should be implantation tested and manufactured in accordance with the Z79 international agreement for safe products. Tracheal tubes are sized by the internal diameter (ID) in millimeters. Despite differences in endotracheal tube wall thickness among manufacturers, a first approximation for the correct tracheal tube size for an average child older than 2 years of age is72:
Preterm infants weighing less than 1500 g generally require a 2.5-mm ID tracheal tube, whereas preterm infants of more than 1500 g require a 3.0-mm ID tube, full-term neonates require a 3.0- to 3.5-mm ID tube, a 1-year-old requires a 4.0-mm ID, and a 2-year-old requires a 4.5- to 5.0-mm ID. Although it has been suggested that the correct diameter of the tracheal tube may be determined by examining the diameter of the distal phalanx of the child’s first or fifth digit, evidence suggests that this is unreliable.69 Tracheal tubes at least one half-size larger and smaller than estimated should be immediately available to accommodate airway size variability. The only true test for appropriate size selection is that the tube passes through the subglottis easily and has an appropriate leak (e.g., between 10 and 40 cm H2O peak inflation pressure). The leak may be easily assessed by closing the circuit pop-off valve and slowly increasing the pressure by gently squeezing the anesthesia bag while listening over the larynx with a stethoscope. This technique has been demonstrated to be a sensitive and accurate measure of fit between the tracheal lumen and the endotracheal tube.73 It should be borne in mind that a leak is dependent in part, upon the child’s head position and degree of paralysis.74 For example, if the neck is flexed or the head is turned from side to side, a leak may become worse or decrease. If the trachea was intubated without the use of muscle relaxants, the child must be well anesthetized to assess the leak properly. If a child is coughing, it is difficult to make this assessment. Conversely, if the child has laryngospasm around the tube, then it will appear to be too large because a leak may not be present. On the other hand, too large a leak may prevent adequate ventilation should positive-pressure ventilation be required, pollute the OR excessively, and dilute the inspired gases during spontaneous respirations. In this circumstance, the tracheal tube should be replaced with the next larger size and the leak reassessed. The relationship of age, tracheal tube sizes and inhalaton pressure leak is commonly used to estimate laryngeal size (see Fig. 31-13).
The past decade has seen a transition from the routine use of uncuffed tubes in children during general anesthesia to the routine use of cuffed tubes. Uncuffed tubes are available for use from 2.0-mm ID to 6-mm ID and have been used as a standard in children up to 8 years of age. Indeed, traditional teaching suggests that the use of uncuffed tubes is the most appropriate practice75; however, this is not evidence-based. The use of cuffed tracheal tubes has been demonstrated to reduce costs of inhalation agents use because of the ability to use lower fresh gas flows.76 With the introduction of the MICROCUFF (Kimberly-Clark Health Care, Roswell, Ga.) tube and publication of several clinical studies, practice has shifted from the routine use of uncuffed to cuffed tracheal tubes. The external diameter of cuffed tracheal tubes is approximately 0.5 mm larger than uncuffed because of the cuff, so one must use a smaller-ID tube for a given tracheal size. Cuffed tracheal tubes as small as 3.0 mm ID are available for use in elective and emergency surgery in infants and children. Some have proposed that the advantages of a cuffed tracheal tube for children include reduced number of reintubations, decreased leak around the tracheal tube leading to less contamination of the operating room, greater ease and consistency of ventilation, reduced cost if low-flow anesthesia is used, and a theoretical attenuated incidence of aspiration.76–82 If a cuffed endotracheal tube is used, the cuff is generally inflated just enough to provide a leak between 20 and 30 cm H2O peak inflation pressure or cuff pressure can be monitored using a device that continually monitors cuff pressure.83 It must recognized that the endotracheal tube cuff pressure will increase during a nitrous oxide (N2O)-supplemented anesthetic because of diffusion of N2O into the cuff,84 unless saline is used to fill the cuff.85 However, because the MICROCUFF tube seals the airway at a reduced pressure compared with polyurethane cuffed tubes, the time until the pressure within the cuff becomes excessive with the MICROCUFF tube is greater than with traditional tracheal tubes.86 Excessive cuff pressures may present a special additional hazard to children, in whom swelling of the pseudostratified columnar epithelium that lines the inside of the cricoid ring may critically reduce the diameter of the upper airway. To date, studies have not investigated long-term complications such as subglottic stenosis that might occur in the intensive care unit. Tracheal tubes used in the OR remain in the trachea for only a brief time; thus it is unlikely that such use could result in long-term complications. An important advantage of the MICROCUFF tube is that the cuff is located closer to the tip of the tube (thereby reducing the potential for cuff pressure on the vocal cords or the cricoid cartilage, but eliminating the Murphy eye), lower cuff sealing pressure (again potentially reducing injury to the mucosa of the trachea), and less leakage around the cuff (less microaspiration) (see Fig. 12-15).87–92 However, the marked nearly fivefold difference in cost will likely prohibit the routine use of this tracheal tube for short term intubation. Additionally, reports of easy kinking in the smaller size tubes resulted in a product recall.93 The authors’ practice has increased the use of the Mallinckrodt Lo-Pro Endotracheal Tubes (Nellcor, Pleasanton, Calif.), which is not a new product but anecdotally provides a low-pressure cuff that has a lower contour, aiding the view during tracheal intubation, and has a smaller effect on the size of the outer diameter of the tracheal tube. No specific studies have demonstrated any differences in complications when comparing different low-pressure endotracheal tubes. The original Khine formula (4 + age [years]/4)79 has been critically evaluated, and a new formula with greater tracheal tube size accuracy has been proposed (3.5 + age [years]/4).79,94 However, these studies did not adequately examine the infants in the lowest age range, nor did they examine these specific brands of tracheal tubes. The argument could be made that long-term follow-up data for complications has not been adequately investigated.95 Currently, we do not recommend the routine use of 3.0-mm ID cuffed tracheal tubes in neonates because the potential for laryngeal/tracheal damage far outweighs the advantage of avoiding an extra laryngoscopy and intubation.
Molded preformed tracheal tubes are especially useful for head and neck surgery because they remove the anesthesia circuit connections from the surgical field.96 When excessive external pressure may be applied to the tracheal tube or when extreme flexion of the neck is likely to kink a standard endotracheal tube, a tracheal tube with Tovell spiral wire reinforcement may be used. Its kink resistance is well-known, but the additional wall thickness limits its applicability in younger children. The spiral springlike construction has caused the tube to pop out of the airway; in addition, repeated improper sterilization of nondisposable models may lead to bubble formation within the rubber, leading to airway obstruction when used with N2O.97
The carbon dioxide (CO2) laser for treatment of laryngeal polyposis and other airway lesions has introduced the problem of ignition of the tracheal tube or other material in the airway. Avoiding high levels of O2 and N2O, which both support combustion, diminishes the risk of fire. Special stainless steel and metal implanted tracheal tubes are available but are quite expensive. Protecting the tube surface with aluminum or copper foil or wet sponges and red rubber tracheal tubes, which have a much reduced ignition potential, may offer the greatest protection (see Chapter 31).98–102 Electrocautery can ignite standard tracheal tubes, esophageal stethoscopes, oral airways, feeding tubes, tissue, and packs if the correct circumstances combine.103–105 Rubber tracheal tubes may soon no longer be manufactured because they are made of latex rubber but are still being advocated by some practitioners for laser surgery.
where NRe is the Reynolds number, D is the diameter of the airway or blood vessel, and V is the velocity, ρ is the density, and µ is the viscosity of the gas or fluid. An NRe less than 2100 denotes flow that is laminar, whereas an NRE of 3000 or greater denotes turbulent flow. Flows that have NRe between 2100 and 3000 may be either laminar or turbulent. Conceptually, laminar flow is organized flow in which each plate of fluid, air, or gas moves in parallel at the same speed, with zero velocity at the interface between the wall of the airway or blood vessel and the gas or fluid. Laminar flow occurs in blood beyond the aortic arch and in airways beyond the fifth bronchial division. In contrast, turbulent flow is chaotic, unpredictable flow in which the fluid, air, or gas does not flow in parallel plates but rather develop eddies and lateral as well as forward flow, thus dissipating large amounts of energy. Turbulent flow occurs in the aortic arch and in the large airways of the tracheobronchial tree down to the fifth bronchial division.
Laminar flow is characterized by the Hagen-Poiseuille equation:
Where ΔP is the pressure drop, Q is the flow rate, η is the viscosity of the fluid (air or gas), l is the length of the airway or blood vessel, and r is the radius of the airway or blood vessel. Thus pressure drop is proportional to the flow rate and viscosity of the fluid and inversely proportional to the radius to the fourth power.
Where ΔP is the pressure drop, Q is the flow rate, ρ is the density and r is the radius of the airway or blood vessel. Note that in contrast to laminar flow, pressure drop is proportional to the square of the flow rate and inversely related to the fifth power of the radius.
Therefore the resistance to gas or fluid flow is greater in relation to the longer and narrower tracheal tube (or intravenous catheter) used; anything that increases turbulence may proportionally increase the resistance to gas or fluid flow. In practical terms, this means that with a tracheal tube, when the respiratory rate is increased, turbulent flow results (meaning that resistance changes with radius to the fifth power, not the fourth power), decreasing the size of the delivered tidal volume.106 In addition, flow may vary from laminar to turbulent at different portions of the respiratory cycle. Studies with in vivo models have shown that this effect is less pronounced with progressively larger tracheal tubes; however, larger tubes may result in reductions in delivered tidal volume when the respiratory rate is increased in children who require a smaller than normal endotracheal tube; that is, an increase in respiratory rate from 10 to 20/min does not double the delivered minute ventilation.106–108 Data suggest that in children with poorly compliant lungs, lung compliance seems to be the most important factor, even with very small tracheal tubes.106 The implications of these observations are that accurate measurement of end-expired CO2 or arterial blood gases are the only way to be certain of the effectiveness of ventilation. In patients with severe pulmonary disease with ventilation-perfusion mismatch, only arterial blood gases or transcutaneous CO2 monitoring will provide the needed information.
Intubation Equipment
Routine Equipment
Intubation equipment must suit pediatric children of all sizes. Laryngoscopes are available with lightweight small handles and a full range of blades (see Chapter 12). An Oxyscope (Foregger, Inc. Langhorne, Pa.), which incorporates a small-bore O2 delivery tube, reduces the incidence of cyanosis and bradycardia in spontaneously breathing neonates should laryngoscopy be prolonged (see E-Fig. 12-12, A and B).109 Magill forceps are available in several sizes for children of different ages.
Special Airway Equipment
Difficult airways require special intubation equipment; flexible and rigid fiberoptic laryngoscopes and bronchoscopes are available for infants and children. Flexible fiberoptic laryngoscopes are available as small as 1.8 mm external diameter; these are suitable for passage of 2.5-mm ID endotracheal tube.110 The small scope is limited because it does not provide suction. Fiberoptic endoscopic skills should be developed during routine elective cases and in training exercises using airway or full manikin simulators to acquire these skills before encountering emergency cases or children with abnormal airway anatomy (see Chapter 12).110 Fiberoptic intubation through the LMA in infants and neonates with midfacial hypoplasia allows the maintenance of a clear airway, delivery of O2 with or without inhalation anesthetic and provides optimal conditions for successful placement of the tracheal tube.61,62,111–114
Lighted stylets provide an alternative technique for management of a difficult airway.115–118 Other airway devices such as the Bonfils fiberscope (Karl Storz, Tuttlingen, Germany), the Airtraq disposable optical laryngoscope (Prodol Meditec, Vizcaya, Spain), the GlideScope video laryngoscope (Verathon, Bothell Wash.), the Storz DCI video laryngoscope (Karl Storz, Tuttlingen, Germany), and the Trueview PCD Infant (Truphatek, Netanya, Israel) can optimize the management of difficult pediatric airways119–125 (see Chapter 12 for a more in-depth discussion).
Suction Apparatus
A functioning suction apparatus must be available before beginning any anesthetic administration. The device should be capable of regulated pressures; suctioning the oropharynx requires a greater vacuum level than suctioning the tracheal tube. Special circumstances (e.g., a full stomach and arterial bleeding in the oropharynx) may require a second suction device. A separate vacuum system is needed to scavenge waste anesthetic gases. Several sizes of suction catheters are helpful, depending on the indication—tracheal, gastric, or oropharyngeal suction. A 6 or 8F suction catheter with a thumb-controlled side port is useful for suctioning through small tracheal tubes, whereas 10, 12, and 14F catheters are useful for larger tracheal tubes. Routine suction of stomach contents after induction of anesthesia may reduce the potential for gastric fluid regurgitation and serious pulmonary aspiration of gastric contents. In larger patients, vented catheters (e.g., Salem Sump; Sherwood Medical, St. Louis) may be more efficacious than unvented catheters. Suctioning the stomach with the child in the supine position and in left and right lateral decubitus positions has been shown to be most efficient in evacuation of stomach contents.126
The Anesthesia Machine and Its Appendages
Anesthesia Machine
All children can be anesthetized with standard adult machinery as long as the anesthesiologist is aware of the pitfalls and limitations. No anesthesia machines are currently marketed solely for pediatric use; however, anesthesia machines that have the ability to be customized for pediatric use may be of value, but not necessary. If one wishes to use special circuits, a Mapleson D system may be permanently mounted, allowing the choice of either a circle or open system. Modifying the anesthesia machine has inherent danger, especially for inexperienced users; the circuit or other components could be assembled incorrectly and potentially be unsafe. In addition, air should be available from a central pipeline or a cylinder with an appropriate yoke and flow meter. This is particularly advantageous in circumstances in which N2O or a high inspired O2 concentration is contraindicated (avoiding hyperoxia in a premature infant, N2O in a patient with bowel distention, or laser airway surgery).127–129 Even though machines are designed not to allow the delivery of a hypoxic gas mixture, an in-line O2 analyzer and pulse oximetry are indicated to use an air–oxygen blend safely. The anesthesia machine should also be equipped with an alternative means of providing O2 and positive-pressure ventilation (self-inflating bag) in case of a machine failure or malfunction.
Scavenger
The scavenging of anesthetic waste gases to limit exposure of anesthetic gases to health care providers is important. However, during pediatric anesthesia, exposure to anesthetic gases occurs during inhalational inductions by mask where high fresh gas flows are used and a poor mask seal exists because of patient anxiety or movement. The use of uncuffed tracheal tubes with large leaks also increases exposure. Modern scavenging systems are designed to avoid applying either negative or positive pressure to a patient circuit by using an open system, in which a reservoir is used to collect the waste gases and active (suction) or passive pressures are used to remove waste gases from the OR.130 In a closed scavenging system, positive or negative relief values are used in the reservoir or waste gas exhaust hose to ensure that positive pressure does not build up in the system or if the system is blocked so negative pressure is not applied to the patient circuit.131,132 In addition to scavenging systems, exposure to anesthetic gases is also related to adequate ventilation of the OR.133
Circuits
A full spectrum of anesthetic circuits have been used in infants and children.134 Adolescents and older children may be anesthetized with a standard adult semiclosed circle absorber system, perhaps with the substitution of a smaller rebreathing bag. Younger children may be anesthetized with the circuit modified by replacing the hoses with small-diameter pediatric tubing and by substituting a smaller reservoir bag. In the past, most infants and neonates (10 kg or less) were anesthetized with nonrebreathing open systems, such as the Mapleson D or F variety. These circuits are used infrequently today and may soon be of historical interest only because the increasing pressure for cost containment and the expense of the newer anesthetic agents has forced most children’s hospitals to convert to circle systems. In our institutions, we use only circle systems; this has simplified teaching and markedly reduced anesthetic agent costs. However, because Mapleson D systems are still being used, the advantages and disadvantages of open and closed systems for infants and neonates are reviewed in the following discussion.
Mapleson D systems do not have directional valves or a CO2 absorber, thus eliminating the resistance intrinsic to the opening pressure of circuit valves and to turbulent flow through soda lime.135 This may be an advantage in spontaneously breathing neonates during induction before intubation. The main disadvantage of open systems is the need for relatively high fresh gas flows and waste of expensive anesthetic agents, as well as unnecessary pollution of the environment. The work of breathing may be important for spontaneously breathing infants, so most pediatric circuits and masks are designed to eliminate both dead space and resistance. The classic example of this type of system is the Ayre T-piece; this circuit includes no valves or reservoir bags (Fig. 51-5).136 Later modifications included changes to the T-piece and expiratory limb.137 The Jackson-Rees modification involves the addition of a reservoir bag to the expiratory limb (Mapleson F).138
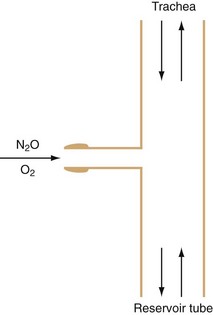
FIGURE 51-5 The T piece as described by Ayre.
(From Ayre P. The T-piece technique. Br J Anaesth 1956;28:520-3.)
The Magill circuit and its various modifications, Mapleson A to E (Fig. 51-6) remain popular.139 These systems consist of a source of fresh gas flow into the circuit, a reservoir bag, a pressure-relief (pop-off ) valve, tubing of various lengths connecting all of these parts, and an adapter for the mask or tracheal tube. Each of these has advantages and disadvantages, which are well reviewed elsewhere.130,134,140,141 The Mapleson D variety is the most commonly used because of its safety and versatility with both controlled and spontaneous ventilation.141 The pop-off valve is at the end of the expiratory limb, just before the reservoir bag; thus, fresh gas washes alveolar gas out of the expiratory limb during expiration. The efficiency of this washout (to prevent rebreathing) depends on the volume of the expiratory limb, the fresh gas flow, and the size of the tidal volume.130 Larger children (heavier than 15 kg) require greater fresh gas flow rates to prevent rebreathing during spontaneous ventilation.141,142 Fresh gas flow rates as low as 1 times minute ventilation may be used with controlled ventilation without an increase in expired CO2 values.143 Rebreathing (an increase in inspired CO2) can occur in children with controlled ventilation when the respiratory rate increases above 20 breaths per minute; this apparent rebreathing can be diminished by using greater fresh gas flows (E-Fig. 51-2). The reason for this phenomenon may relate to inadequate washout of expired alveolar gas before the onset of the next breath. The advantages of minimal dead space and low resistance to flow with nonrebreathing circuits are counterbalanced by the disadvantages of heat and humidity lost to the anesthetic gases and significant waste of anesthetic agents because of the high flows required.134,141 A clinically important factor is that whenever a change in anesthetic gas concentration is made at the vaporizer, this change is immediately reflected at the airway. This allows a more rapid induction of anesthesia, which may be an advantage but also means a greater risk of producing an anesthetic overdose when compared with a circle system. The most common and efficacious use of the Mapleson D circuit is for the safe transport of a child to and from the OR and for intrahospital transport. In this situation the light weight of the system, the ability to easily adjust peak inflation pressure and provide positive end expiratory pressure, and the ability to use relatively low O2 flows make this system far superior to self-inflating (Ambu type) devices.