CHAPTER 75 PATHOPHYSIOLOGY OF MULTIPLE SCLEROSIS: DEMYELINATION AND AXONAL INJURY
The inflammatory demyelinating lesions of multiple sclerosis cause a range of axonal conduction abnormalities that, in turn, produce the major clinical features of the illness. The clinical effects of these lesions were previously thought to arise directly from the consequences of the demyelination alone, but more recent findings have substantially broadened this understanding. It is now recognized that (1) conduction abnormalities reflect a reorganization of axonal membrane currents after demyelination, (2) inflammation may have direct effects on axonal function, and (3) neuroaxonal degeneration plays a significant role in disease progression.
DEMYELINATION
Action potentials generated by inward sodium currents at the nodes of Ranvier propagate successfully along normal myelinated axons because of the insulating layer of myelin covering the internode. The thickness of the myelin reduces the leakage of current between the nodes by increasing the resistance of the membrane and, of more importance, by reducing its capacitance. These properties have the effect of reducing the leakage of current between nodes and thus reducing the current that needs to be generated at the node for secure conduction to occur. In normal myelinated axons, the action potential at an individual node generates three to seven times more current than required to discharge the next node along the axon; this is referred to as a safety factor of three to seven.1
It is now clear that the membrane of the adult myelinated axon has a complicated molecular structure (Fig. 75-1).2,3 Apart from sodium channels, which are clustered at the nodes and expressed at a much lower density in the internodal membrane, there are also a number of potassium channels, including so-called slow channels, located mainly at the nodes, and faster channels, located in the juxtaparanodal region. These serve to limit the excitability of the fiber.4 There are a number of other potassium channels, of which some are inhibited by adenosine triphosphate (ATP) and others are activated by intracellular calcium ions. The axonal membrane is also known to contain calcium channels, but these do not appear to contribute significantly to action potential generation or propagation, at least under normal conditions. The ionic balance across the axonal membrane is maintained by a number of ATP-dependent pumps, including the sodium and calcium pumps, and calcium homeostasis also depends on the sodium/calcium exchanger.
Demyelination reduces the safety factor for conduction, and conduction fails, at least initially, if an internode or more of myelin is removed (Fig. 75-2). The failure occurs partly because the local excitatory sodium current generated by the last node of Ranvier before the demyelinated segment is now dispersed across a much wider area of naked axonal membrane than just the nodal membrane. Even partial-thickness demyelination increases the effective capacitance of the axolemma as the thickness of the myelin is reduced, increasing the amount of local current necessary to depolarize the nodal membrane to its firing threshold and perhaps resulting in conduction block. Conduction is also likely to be blocked, or severely impaired, if the myelin loss occurs preferentially at the paranodes, which causes nodal widening and increases the capacitance of the “nodal” axolemma. Although conduction may later be restored (see later discussion), the density of sodium channels in the newly exposed axolemma is initially too low to support conduction along the demyelinated axolemma.5,6
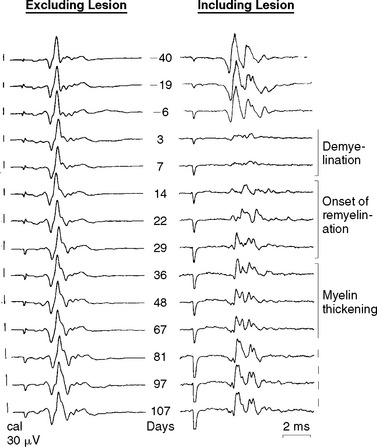
(Reproduced from Smith KJ, Blakemore WF, McDonald WI: Central remyelination restores secure conduction. Nature 1979; 280:395-396. Reprinted by permission from Macmillan Publishers Ltd. Copyright 1979. http://www.nature.com/index.html.)
Effects of Temperature
Small changes in temperature can have dramatic effects on conduction in demyelinated axons7–10 (Fig. 75-3), because the safety factor in demyelinated axons is typically reduced near to unity. The success of conduction in many demyelinated axons is therefore finely balanced, inasmuch as small changes can tip the safety factor either to just below unity, in which case conduction is blocked, or to just above unity, in which case conduction will succeed. Temperature changes affect the safety factor by altering the kinetics of the sodium channels.11 Warming shortens the action potential,12 thereby reducing the time that current is able to flow to depolarize the demyelinated region to its firing threshold, and cooling has the opposite effect.
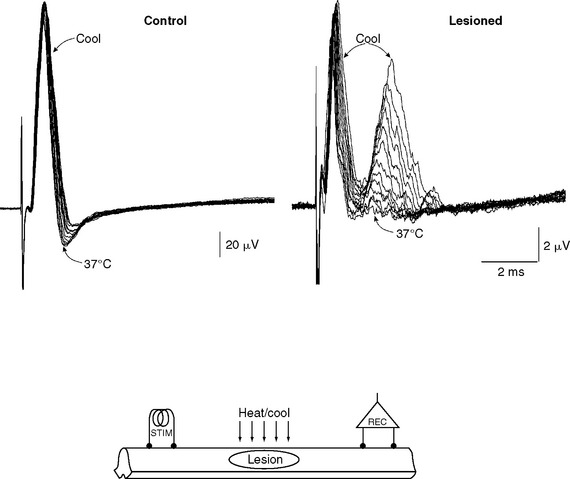
(Reproduced from Smith KJ: Conduction properties of central demyelinated and remyelinated axons, and their relation to symptom production in demyelinating disorders. Eye 1994; 8:224-237. Reprinted by permission from Macmillan Publishers Ltd. Copyright 1979. http://www.nature.com/eye/index.html.)
Even subtle changes in body temperature can profoundly alter the expression of symptoms, such as in the visual system (Uhthoff’s phenomenon).13 Thus, vision can improve simply by drinking a glass of cold water,14 whereas a hot shower or bath, or sunbathing, can aggravate disability significantly.15 Indeed, in earlier years, the deleterious effects of body warming underpinned the “hot bath test” for multiple sclerosis. The therapeutic benefits both of body cooling11,16 and of prolonging the action potential17,18 have been considered. Thus, the potassium channel-blocking drugs 4-aminopyridine and 3,4-diaminopyridine can prolong the action potential, favoring successful conduction in demyelinated axons.19 These drugs have been found to improve disability in clinical trials in multiple sclerosis,20 although it has also been suggested that this effect could arise from the potentiation of synaptic transmission.21 The widespread use of aminopyridines has been inhibited by the fact that they are markedly proconvulsant at doses that are not much higher than the therapeutic dose.22
Activity-Dependent Conduction Block
Apart from fixed conduction block, demyelinated axons with a safety factor just above unity have a prolonged refractory period for conduction through the lesion23 (Fig. 75-4) and can also exhibit intermittent conduction failure as a result of periods of sustained electrical activity. The rise in the intra-axonal sodium concentration with repetitive firing stimulates the activity of the electrogenic Na+/K+-ATPase (sodium pump) and leads to axonal hyperpolarization.24 Even a small hyperpolarization can effectively, and abruptly, block conduction in such demyelinated axons for periods of approximately 0.2 to 2 seconds.25 Conduction resumes as the intra-axonal sodium concentration recovers and pump activity falls, and this cycle may then be repeated, causing a continuous train of impulses to be divided into short bursts separated by silent periods. The intermittent failure of impulse transmission on sustained activation may contribute significantly to the fatigability experienced by many patients with multiple sclerosis: for example, those with spinal cord lesions whose gait becomes impaired on walking short distances, or those whose vision “fades” or becomes blurred on fixated gaze, particularly in bright surroundings.26,27 There is therefore a potential therapeutic role for partial blockade of Na+/K+-ATPase, and indeed some benefit with such a therapy has been demonstrated in a small number of patients.28
REMYELINATION
There is ample evidence that demyelinated axons can undergo repair by remyelination in multiple sclerosis29,30 and that remyelination is likely to play a major role in restoring conduction and in the remission of neurological deficits after relapses.31 New internodes are thinner and shorter than normal, but remyelinated axons can nonetheless conduct, even when invested with only thin new myelin sheaths composed of as few as five myelin lamellae.32 New nodes formed by remyelination show aggregations of sodium channel immunoreactivity,33 and, at least in the peripheral nervous system, the new nodes are excitable.34 Not only do axons repaired by remyelination conduct (see Fig. 75-2) but also remyelination restores the security5,35 and velocity of conduction to near-normal values regardless of whether the repair is effected primarily by oligodendrocytes,35 Schwann cells (even within the central nervous system),36,37 or transplanted olfactory ensheathing cells.38 Although the role of axonal impulse activity in promoting myelination and remyelination remains controversial, it is possible that because demyelinated axons often exhibit conduction block, the absence of electrical activity could contribute to the failure of remyelination in some multiple sclerosis lesions.39,40 Remyelination is likely to confer not only secure conduction to repaired axons but also protection from degeneration, and developing strategies to promote remyelination is an active area of research.
ADAPTIVE CHANGES IN THE EXPRESSION OF ION CHANNELS IN DEMYELINATION
The clustering of voltage-sensitive ion channels and other axolemmal proteins, such as the L1 cell adhesion molecule family members neurofascin and NrCAM, and 480/270kD ankyrin at nodes of Ranvier,41 requires contact with, or at least close proximity to, myelinating Schwann cells in the peripheral nervous system and with oligodendrocytes in the central nervous system.42–46 Because maintenance of ion channel distribution also seems to depend, at least in part, on the presence of compact myelin and the formation of normal, tight paranodal axoglial junctions,47–50 demyelination can produce changes in the patterns of expression of these ion channels. In fact, the restoration of conduction in demyelinated axons involves substantial remodeling of the axonal membrane at a molecular level. Immunocytochemical studies have revealed an increased expression of two different isoforms of voltagegated sodium channels, Nav 1.6 and Nav 1.2, along demyelinated axons.47,48,51 Both of these sodium channels, particularly Nav 1.6, can generate persistent sodium currents, which may be important in axonal degeneration (see later discussion). The Nav 1.8 channel, which is normally expressed only in adult spinal sensory and trigeminal neurons, has also been shown to occur aberrantly in Purkinje cells in multiple sclerosis and in experimental autoimmune encephalomyelitis and potentially to contribute to cerebellar dysfunction.52–55
On physiological examination, both a seemingly continuous distribution of sodium channels56 and, in contrast, an aggregation of sodium channels into “φ-nodes,”34 have been described. φ-Nodes appear to be the precursors of the new nodes of Ranvier formed during remyelination, and it may be significant that the initial observation of a continuous distribution of sodium channels was made in a lesion (diphtheria toxin) in which repair by remyelination is only slowly achieved. It is still not clear how sodium channels aggregate during remyelination to form the new nodes of Ranvier. The formation of the channel aggregations may be driven by the axon and may also depend on the adjacent myelinating cells.33,44,46,50,57,58
These adaptive changes can, in some axons, lead to the restoration of conduction, even in the absence of repair by remyelination. Factors favoring the restoration of conduction in a particular axon include a small diameter59,60 and either a short internode59,61,62 or a widened node before the demyelinated region. The first two of these factors characterize axons in the optic nerve, and they may contribute to the excellent recovery of vision that can follow optic neuritis. However, axons as large as 5.5μm in diameter have been shown to conduct in the absence of remyelination, which raises the possibility that most central axons might be able to conduct under ideal conditions. This possibility may help explain the presence in some patients of clinically silent demyelinating lesions in pathways that usually manifest in symptom production.63–67
The restoration of conduction in segmentally demyelinated axons was first demonstrated by a sophisticated examination of conduction in spinal root axons demyelinated by the intrathecal injection of diphtheria toxin56; nearly 20 years were to pass before there was proof that conduction could also occur in demyelinated central axons,32 although circumstantial evidence had accumulated.68–71 In the peripheral nervous system, conduction can resume as soon as 6 days after the induction of a demyelinating lesion, but in the central nervous system, longer intervals (e.g., 2 to 3 weeks) appear to be required. Once restored, conduction is continuous, or microsaltatory, rather than saltatory, and it is therefore much slower than normal and also less secure. The velocity of conduction along a demyelinated segment of rat ventral root axons is reduced from normal, to the range of 0.7 to 2.3m/second34,56 and similar values are likely to apply in the central nervous system. Even though this degree of slowing occurs across only the demyelinated portion of an axon, it is still sufficient to disperse and delay the compound action potential and, perhaps coupled with a reduction in the total number of axons conducting, to result in diagnostically valuable delays in the visual,72 somatosensory, and brainstem auditory evoked potentials. Slowing of conduction (as opposed to conduction block) generally causes few symptoms, although sensations dependent on the precise timing of impulses in different axons may be affected. For example, patients with unilateral optic neuritis may perceive the Pulfrich phenomenon (the tendency of a pendulum to appear to trace a circle when a neutral density filter is placed over one eye, because of delayed transmission of information in the filtered pathway) without the normal need of the filter,73 and auditory functions dependent on precisely coordinated information may be distorted by a unilateral lesion in the auditory pathway.
PLASTIC CHANGES
Recovery from a relapse of multiple sclerosis is also likely to involve “plastic” cortical changes; functional magnetic resonance imaging and other evidence support this view.74–81 Thus, to accomplish even minor tasks, patients with multiple sclerosis activate a larger part of the cortex than normal, including cortical areas not normally associated with the particular activity. Whether this increased cortical activation contributes to the sense of fatigue experienced by many patients remains unresolved.
POSITIVE SYMPTOMS: AXONAL HYPEREXCITABILITY
The recovery processes in demyelinated axons can lead not only to the restoration of conduction but also to axonal hyperexcitability.82,83 Ectopic impulses can be generated in regular or bursting discharges, and they conduct away from their site of initiation in both directions along the axon.84,85 Discharges in sensory pathways may give rise to tingling sensations referred to the body parts normally innervated by those axons. Similar discharges are likely to underlie other paroxysmal phenomena, including trigeminal neuralgia, tonic spasms, hemifacial spasm, and possibly episodic dysarthria and limb ataxia. Positive phenomena can be enhanced in patients by measures that increase axonal excitability, such as hyperventilation, and treatment with bicarbonate or calcium chelators.83,86–88
It appears that spontaneous discharges can arise from the excitatory effects of inward sodium currents that can develop in the demyelinated axolemma,83,89 and sodium channel blockers such as carbamazepine and phenytoin are effective treatments for positive symptoms. Spontaneous discharges could also arise from inward potassium currents that can result from the accumulation of potassium ions in limited extracellular compartments around axons.90 Finally, it has been proposed that impulses can sometimes be “reflected” from sites of demyelination91–93; that is, an impulse propagating through a demyelinated site can induce the formation of a second impulse that travels back along the same axon in the opposite direction. A pair of reflecting sites could generate a train of apparently spontaneous impulses. Single, normally evoked impulses propagating through generator sites where any of these mechanisms of hyperexcitability have arisen could also trigger episodes of ectopic activity.25,91,94,95
Sensory axons are more prone to develop ectopic discharges than are motor axons, and, accordingly, positive sensory phenomena are more common in demyelinating disease than are their motor counterparts.96,97 These differences may occur partly because of the greater expression of persistent sodium currents along sensory axons.98 The application of tumor necrosis factor directly to the sciatic nerve has been found to evoke ectopic discharges in nociceptive axons, including unmyelinated axons.99 It is therefore possible that in inflammatory demyelinating diseases such as multiple sclerosis, tumor necrosis factor is involved in promoting the generation of ectopic discharges along demyelinated axons within inflammatory foci. Other inflammatory mediators may also favor ectopic activity. For example, exposure to nitric oxide is known to be conducive to persistent opening of sodium channels.100,101
Ephaptic Transmission between Axons
Ephaptic interactions involving electrical crosstalk between demyelinated axons can explain a number of clinical observations in which paroxysmal, positive phenomena affect anatomically adjacent axonal pathways.102–104 For example, tonic spasms may involve sensory symptoms along with contralateral limb contraction, as expected from a lateral spread of excitation from the spinothalamic to the corticospinal tract within the spinal cord. Hartmann102 described a patient in whom trigeminal neuralgia could be triggered by auditory stimuli and who had a pontine lesion involving the adjacent lateral lemniscus and trigeminal sensory pathway on magnetic resonance imaging. That said, ephaptic transmission has rarely been demonstrated electrophysiologically. Indeed, the best documented interaction occurs between normal and amyelinated (i.e., never myelinated, rather than demyelinated) axons in the spinal roots of the dystrophic mouse.105,106