2.3 Paediatric advanced life support (PALS, APLS)
Introduction
Definition of ALS
The recommendations for advanced CPR given here are based on publications of the Australian Resuscitation Council,1 the European Resuscitation Council,2 the American Heart Association3 and the International Liaison Committee on Resuscitation (ILCOR).4 They are intended for use by medical and nursing personnel in hospital and by ambulance personnel in the field.
Diagnosing cardiac arrest
Healthcare personnel (doctors and nurses) have difficulty diagnosing cardiac arrest in infants and children if they rely on pulse palpation alone. Their accuracy is approximately 80% with a sensitivity of 0.85 and specificity of 0.65,5 which means that in 15% of circumstances they would not give CPR when needed and would give it in 35% when not needed. While application of CPR is not harmful when there is a circulation, the withholding of CPR when there is none dooms the patient to die. The time taken to diagnose cardiac arrest is longer than hitherto realised6 – as a group, healthcare personnel take an average of 15 seconds to exclude cardiac arrest by finding a pulse but 30 seconds to diagnose real cardiac arrest by the absence of a pulse. However, the accuracy and expediency of diagnosis are related to experience and training. Only experienced personnel who palpate pulses on a daily basis are able to detect a real pulse within 10 seconds but they, like inexperienced personnel, are unable to quickly diagnose cardiac arrest by the lack of a pulse and need on average about 25 seconds to confirm it. Clinical guidelines advise to spend no more than 10 seconds on pulse palpation and to combine whatever information is gained with observable signs of circulation such as responsiveness, movement and presence or absence of normal respiration. In short, if the patient is unresponsive and not breathing normally there is no point wasting time on pulse palpation (it is inaccurate and time consuming). Instead give CPR immediately.
Oxygen, ventilation and advanced airway support
Ventilation
Self-inflating bags
These bags should not be used to provide supplemental oxygen to a spontaneously breathing patient with a mask placed near or loosely over the face. With Laerdal and Partner bags, negligible amounts of oxygen (0.1–0.3 L min–1) issue from the patient valve when 5–15 L min–1 of oxygen is introduced into bags unconnected to patients.7 The patient valve is unlikely to open unless the mask is sealed well on the face. Although not recommended, if they are used in this way, it is vital to ensure that the patient valve opens or the reservoir bags deflates in unison with the chest movement.
Rates and ratios of external cardiac compression and ventilation in ALS
The techniques of external cardiac compression and expired air resuscitation (mouth-to-mouth) or rescue breathing are described in Section 2.2. The recommended ratio of external cardiac compression to ventilation in basic life support by a single rescuer is 30:21–4 which if able to be repeated 5 times in 2 minutes, with pauses for ventilations, would yield approximately 75 compressions and 5 breaths per minute.
Advanced airway support
Tracheal intubation
The trachea should be intubated as soon as practicable but it can be deferred if successful bag–mask ventilation can be given and should not be undertaken by inexperienced personnel out-of-hospital8 because of complications and poorer outcomes compared with use of bag–mask ventilation. Nonetheless, intubation has numerous advantages, which include establishment and maintenance of the airway, facilitation of mechanical ventilation, titration of oxygen therapy, minimisation of the risk of pulmonary aspiration, enablement of tracheal suction, provision of a route for the administration of selected drugs and preferred for transport and long-term ventilation. Regurgitation of gastric contents is common during cardiac arrest.
Endotracheal tube size (Table 2.3.1)
Uncuffed sizes are 2.5 mm for a premature newborn <1 kg, 3.0 mm for infants 1–3.5 kg, 3.5 mm for infants >3.5 kg and up to age of six months, size 4 mm for infants seven months to one year (Table 2.3.1). The approximate size may be chosen for children over one year by the formula: size (mm) = age (years)/4 + 4. Tubes one size larger and smaller should be readily available. The correct size should allow a small leak on application of moderate pressure but also enable adequate pulmonary inflation. If the lungs are non-compliant, however, it may be necessary to insert a tube without a leak or insert a cuffed tube. Appropriate- sized cuffed tubes may be estimated by the formula: size (mm) = age (years)/4 + 3.5.
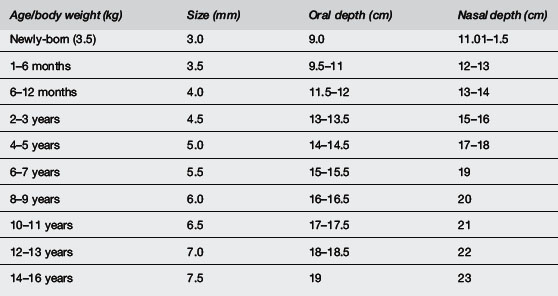
Laryngeal mask airway (LMA)
These have been used for resuscitation by medical, nursing and ambulance personnel trained in their selection and insertion. They may be used to maintain an airway and are a suitable alternative to the use of airway opening manoeuvres and use of oropharyngeal and nasopharyngeal airways. They are useful to establish an airway in the setting of airway obstruction or failed intubation.9 An intubating LMA serves as a conduit for intubation.
However, the role of LMA in provision of mechanical ventilation remains uncertain. Like bag–mask ventilation, they do not protect the airway from aspiration, which occurs commonly during cardiopulmonary resuscitation. They are a suitable alternative to a face mask as a means to give ventilation before endotracheal intubation and when intubation is difficult. This is a better technique when the operator is unskilled in the use of LMA and intubation. Although insertion of an LMA is easier to learn than endotracheal intubation, training should not replace mastery of bag–mask ventilation. They should not be used in semi-conscious patients or when the gag reflex is present and are not suitable for long-term use or use during transport when endotracheal intubation is far preferable. They are subject to dislodgment during movement and transport. Appropriate sizes according to body weight are given in Table 2.3.2.


Management of the difficult airway
Resuscitators must be well-skilled in the management of the airway and provision of bag–mask ventilation. It is vital to possess good basic resuscitation techniques (see Chapter 2.2