13 Pacing for Sinus Node Disease
Sinus node disease (SND) is the most common indication for a cardiac pacing system.1 SND increases exponentially with age, affecting 1 of every 600 cardiac patients older than 65. SND is characterized by electrophysiologic abnormalities of both the sinus node and the atria. These electrophysiologic abnormalities include disturbances of impulse generation and exit from the sinus node to atrial tissue, impaired impulse transmission within the atria and specialized cardiac conduction system, failure of subsidiary pacemaker activity, and paroxysmal or chronic atrial tachycardias, including atrial fibrillation.2,3 The electrocardiographic manifestations of SND include (1) sinus bradycardia, (2) sinus pauses or sinus arrest, (3) sinoatrial exit block, (4) atrial tachycardia, (5) atrial fibrillation (initially paroxysmal), and (6) sinus node chronotropic incompetence.2,3 Bradyarrhythmias alternating with paroxysmal atrial flutter or fibrillation are common in SND. The natural history of SND includes recurrent syncope, heart failure, stroke, and atrial fibrillation.
Pathophysiology and Diagnosis
Cellular Electrophysiology
The sinoatrial node is a heterogeneous tissue with multiple cell types and a complex structure.3,4 The action potential governing pacemaking usually arises in the center of the sinoatrial node and propagates into the surrounding muscle of the right atrium. A variety of ionic currents contribute to normal sinus node automaticity (Fig. 13-1). These include If, a hyperpolarization-activated inward current, and the L– and T– type calcium currents (ICaL, ICaT). At present, debate remains over which of these currents is dominantly responsible for sinus node automaticity.5 There is limited or no measurable sodium current (INa) in the center of the node, but it is measurable in the periphery.3,4 INa may be responsible for activating peripheral cells. Outward currents that govern sinus node automaticity include the sodium-potassium (Na+,K+) pump, the delayed rectifier current (IKr), and the potassium channel activated by the muscarinic receptor, IKACh. The sodium-calcium exchanger and sarcoplasmic reticulum (SR)–mediated calcium ion (Ca2+) release also influence sinus node automaticity. Abnormalities of one or more of these ionic currents may contribute to sinus node dysfunction. During aging, connexin 43 (Cx43) disappears from the sinus node, which may contribute to abnormalities of conduction within the sinus node.3,6
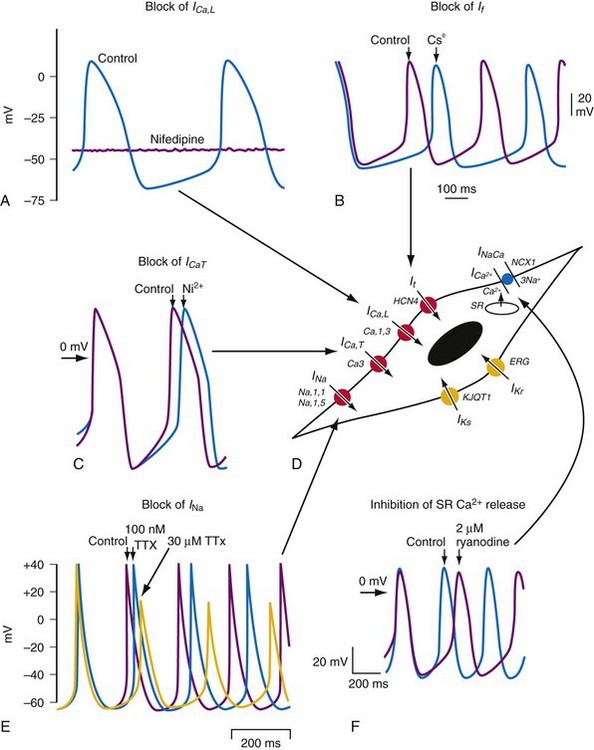
Figure 13-1 Pacemaking mechanisms.
(From Dobrzynski H, Boyett MR, Anderson RH: New insights into pacemaker activity: promoting understanding of sick sinus syndrome. Circulation 115:1921-1932, 2007.)
The molecular architecture of the human sinus node has recently been described.4 A complex and heterogeneous expression of ion channels is present within the sinus node compared to a paranodal region within the terminal crest and right atrial (RA) tissue. There is a lower expression of Nav1.5, Kv4.3, Kv1.5, HERG, Kir2.1, Kir6.2, the cardiac ryanodine receptor, the SR calcium pump, connexin 40 (Cx40), and Cx43 messenger ribonucleic acid (mRNA), but a higher expression of Cav1.3, Cav3.1, hyperpolarization-activated cyclic nucleotide–gated channel genes (HCN1, HCN4) mRNA in the sinus node compared to RA tissue (Figs. 13-2 and 13-3). Similar differences in expression of the corresponding ion channel proteins are observed in the sinus node compared to RA tissue. The expression pattern of many ion channels in the paranodal area is intermediate between that of the sinus node and right atrium, although greater expression of Kv4.2, Kir6.1, minK-related peptides, and other potassium channels (TASK1, SK2) is observed in the paranodal area than in the sinus node or atrial tissue. Estimating conductance of the key ionic currents based on a percentage of the mRNA levels expressed in the sinus node, then incorporating these in a mathematical model of human atrial action potential, successfully produced a model of pacemaking.4
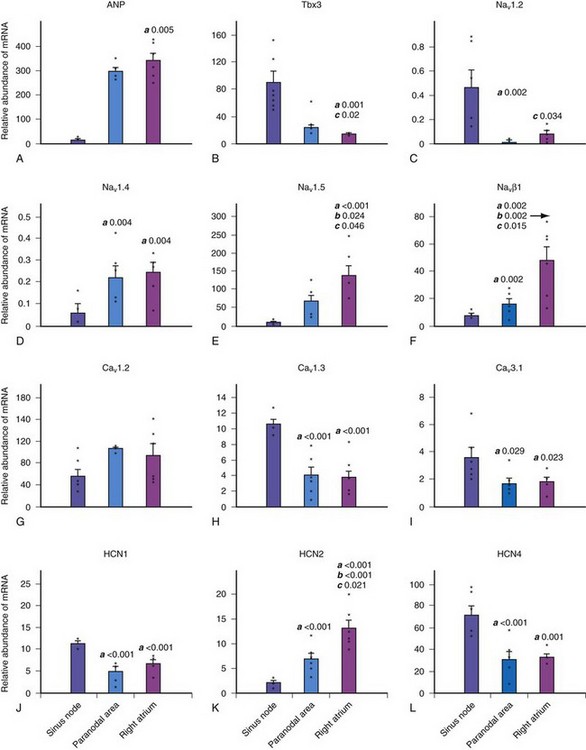
Figure 13-2 Relative abundance of mRNA in sinus node.
(From Chandler NJ, Greener ID, Tellez JO, et al: Molecular architecture of the human sinus node: insights into the function of the cardiac pacemaker. Circulation 119:1562-1575, 2009.)
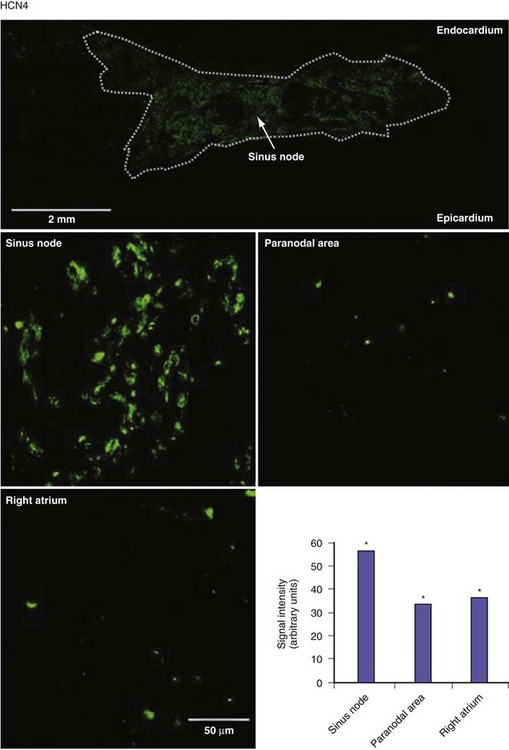
Figure 13-3 Expression of HCN4 protein in sinus node, paranodal area, and right atrium.
(From Chandler NJ, Greener ID, Tellez JO, et al: Molecular architecture of the human sinus node: insights into the function of the cardiac pacemaker. Circulation 119:1562-1575, 2009.)
Abnormalities of one or more ionic channels, transporters, or receptors that are inherited or that develop under pathophysiologic states, including aging or atrial fibrillation (AF), likely contribute to the substrate for SND.3 Knockout of various ion channels (Nav1.5, sodium channel β2-subunit, Cav1.3, Cav3.1, HCN2, HCN4, ankyrin-B) and gap-junction subunits (Cx40) in transgenic mice result in a SND phenotype characterized by bradycardia, sinus dysrhythmia and sinus node exit block.3–7 Mutations of the HCN4 gene have been associated with reduced membrane expression and decreased If currents in conjunction with abnormalities of sinus node dysfunction.8–10 In an experimental model of heart failure, decreases in the intrinsic sinus rate have been observed in association with significant decreases in If.11 Mutations of the alpha subunit of the sodium channel that causes one form of the long QT syndrome have also been associated with SND.12–14 Such mutations may lead to the presence of a persistent inward current and a negative shift in voltage-dependence of inactivation that either separately or in combination may cause a reduction in the sinus rate. Congenital SND that is characterized by bradycardia progressing to atrial inexcitability has also been associated with mutations of the α-subunit of the sodium channel that cause loss of function or significant impairments in channel gating (inactivation), resulting in reduced myocardial excitability.15 SND has also been linked to mutations in ankyrin-B, a protein important in the regulation of ion channel function.16,17
Knockout of HERG1 B, the gene that encodes IKr, in mice has also been associated with episodic sinus bradycardia.18 Mutations of the KCNQ1 gene that encodes the KvLQT1 potassium channel are also associated with SND.19 Thus, decrease or loss of IKr or IKs in the long QT syndrome may contribute to SND in that setting. Maternal antibodies may contribute to sinus node dysfunction in the setting of congenital complete heart block by inhibition of ICaL and ICaT.20 Knockout of ICaL in transgenic mice has been associated with SND.3,21,22
Some experimental models of ventricular hypertrophy and heart failure have been reported in association with sinus node dysfunction and AF.23–27 Because SND is age dependent, changes in sinus node function may be secondary to changes in ion channels or gap junctions. Loss of INa in the periphery of the sinus node and decreased expression of Cx43 have been reported in animal models of aging and may explain abnormalities of sinus node function.3 Thus, the causes of SND are likely multifactorial.
Clinical Electrophysiology
In normal hearts, the sinus node complex displays a dynamic range of activation sites along the posterolateral right atrium.28 There are multiple origins of sinus activation and exit sites to the atria, providing evidence of multicentricity of the sinus node complex. Electroanatomic mapping has demonstrated that preferential pathways of conduction exist between the sinus node and the exit of sinus activity to the atria. In SND, the sinus node complex more often is unicentric and localized to the low crista terminalis.29,30 Electroanatomic mapping in patients with SND has also demonstrated significant increases in atrial refractory periods at all RA sites, increased atrial conduction times along the lateral right atrium and coronary sinus, and greater number and duration of double potentials along the crista terminalis29 (Fig. 13-4).29
Significant regional conduction slowing with double potentials and fractionation associated with areas of low voltage and electrical silence has also been observed in the right atrium. The slow conduction may be caused by increased fibrosis in the atria, although other determinants of conduction velocity (e.g., INa or connexin expression) have not been studied in patients with SND. Areas of low voltage/scarring in the right atrium can be widespread, progressive, and severe. Similar changes are seen in conditions of chronic atrial stretch and increasing age.30 The mechanism of these changes in patients with SND is unclear. These results indicate not only that there is disease of the sinus node, but that the atrial electrophysiologic abnormalities also extend to the RA myocardium.
Patients with congestive heart failure (CHF) manifest significant sinus node remodeling, characterized by anatomic and structural changes along the crista terminalis, and a reduction in functional sinus node reserve.31,32 Compared to age-matched controls, patients with CHF have greater prolongation of the intrinsic sinus cycle length, more prolonged sinus node recovery time, caudal origin of sinus activity, prolongation of sinoatrial conduction time, greater number and duration of fractionated electrograms or double potentials along the crista terminalis, loss of voltage amplitude along the crista, and abnormal and circuitous propagation of the sinus impulse. In addition, atrial refractoriness and regional conduction times are prolonged, and these changes may contribute to the propensity to AF in this setting.32
Experimental and clinical data suggest that both AF and atrial flutter cause adverse remodeling of the sinus node.33–39 Sinus node activation in patients with persistent atrial flutter is characterized by more caudal activation, slower conduction time along preferential pathways, and modest shifts within the functional pacemaker complex. Prolonged sinus pauses after paroxysms of AF may result from depression of sinus node function that is eliminated by curative ablation of AF.38 Sinus node recovery times are prolonged immediately following successful cardioversion and shorten over time.39 In an experimental model of atrial tachyarrhythmias, downregulation of HCN2, HCN3, and minK subunit expression along with the corresponding currents If and IKs has been reported.40 These changes were associated with abnormalities of sinus node function, whereas the L– and T-type calcium units and Cx43 were unaffected. These data suggest that changes in If and IKs contribute to the abnormalities of sinus node function associated with AF.
The long-term loss of atrioventricular (AV) synchrony induced by VVI pacing is also associated with atrial electrical remodeling, characterized by nonuniform prolongation of atrial refractoriness and prolonged atrial conduction and sinus node recovery times.33 These electrophysiologic changes are reversible after restoration of AV synchrony with DDD pacing. Together, the changes in atrial and sinus node electrophysiology may contribute to the higher incidence of AF observed in patients treated with VVI pacing versus AV sequential pacing. The cellular mechanisms resulting in the association between sinus node dysfunction and atrial tachyarrhythmias (tachycardia) at present remain unknown.
Clinical Presentation
The most common symptoms for which patients with SND seek medical attention include presyncope, syncope, palpitations, decreased exercise tolerance, and fatigue2,3 (Table 13-1). Symptoms are usually intermittent and may be of variable duration. Many patients with electrocardiographic evidence of sinus node disease may be asymptomatic. Symptoms secondary to systemic thromboembolism may also be observed. Syncope may be secondary to profound sinus bradycardia, asystole, or atrial tachycardias. Syncope may occur without warning or may be heralded by dizziness or palpitations. The physical examination is frequently unremarkable, although sinus bradycardia or AF should raise suspicion of SND. However, sinus bradycardia is frequently observed in normal, healthy individuals of all age ranges. Clinical correlation with symptoms is important.
Type | Symptoms |
---|---|
Major |
Diagnosis
Table 13-2 summarizes the diagnostic tools presently available for SND. Because of the intermittent nature of this syndrome, the diagnosis is often time-consuming and frustrating. The sensitivity of both noninvasive tests and invasive electrophysiologic studies to identify SND as a potential cause of syncope are low (4%-16%).2 Implantable loop recorders have increased the likelihood of diagnosing bradycardia as the cause of syncope in patients with SND, and this approach in select patients is more cost-effective than a strategy of serial noninvasive studies followed by invasive studies.41–43
Diagnostic Mode | Device/Measurement |
---|---|
Electrocardiogram (ECG) |
Natural History
The course of SND is unpredictable; periods of symptomatic sinus node dysfunction may be separated by long periods of normal function.2 SND is believed to evolve over 10 to 15 years, commencing with an asymptomatic phase and ultimately progressing to complete failure of sinus node activity and the emergence of subsidiary pacemaker escape rhythms or the development of chronic AF. Menozzi et al.44 performed a prospective study in 35 untreated patients with SND.44 These subjects had a mean sinus rate at rest of 50 beats per minute or less (≤50 bpm) and/or intermittent sinoatrial block, as well as symptoms attributable to SND. The patients were followed for up to 4 years (mean, 17 ± 15 months). During follow-up, 57% of patients experienced at least one cardiovascular event that required treatment. Syncope occurred in 23%, symptomatic heart failure in 17%, permanent AF in 11%, and symptomatic paroxysmal atrial tachyarrhythmias in 6%. The rates of cardiovascular events were 35%, 49%, and 63% after 1, 2, and 4 years, respectively. Older age and associated left ventricular dysfunction were independent predictors of cardiovascular events. The rate of syncope was 16%, 31%, and 31% after 1, 2, and 4 years, respectively. Although a favorable outcome was observed in 43% of patients, the cohort studied was small and duration of follow-up relatively short.
In a cohort of 213 patients with symptomatic SND treated with atrial pacing, the incidence of permanent AF during follow-up was 1.4% per year.45 The risk of developing AF increased substantially in patients age 70 or older at pacemaker implantation. The risk of high-grade AV block was 1.8%/yr and was much greater in patients with complete bundle branch block or bifascicular block (35%) than in patients without such conduction disturbances (6%). Survival rates in this population were similar to those of a matched general population (97% at 1 year, 89% at 5 years, 72% at 10 years). Retrospective data also support the conclusion that survival in patients with SND treated with pacemaker therapy is similar to a general matched population.46
Clinical Outcomes in Sinus Node Disease
Pacing and Survival
A number of retrospective studies47 and one prospective study48 have reported that atrial-based pacing compared to ventricular pacing is associated with improved survival in patients with symptomatic bradycardia secondary to SND. Andersen et al.48 randomized 225 patients (mean age 76 years) with sinus node dysfunction, normal AV conduction, and a normal QRS complex to AAI or VVI pacing. Over a mean of 5.5 years of follow-up, fewer cardiac deaths occurred in the atrial pacing group (19%) compared to the ventricular pacing group (34%; P = .0065). The annual mortality rate was 3% in the atrial versus 6.4% in the ventricular pacing group.
However, these results have not been confirmed in three larger clinical trials. The Pacemaker Selection in the Elderly (PASE) trial randomized 407 patients age 65 and older to receive a dual-chamber pacemaker programmed to either the DDDR or the VVIR mode.49 Overall mortality was similar in the two pacing groups. Of the 175 patients with SND as the primary indication for pacing in this study, overall mortality was slightly higher in the VVIR group (8.8%/yr) than the DDDR group (5.2%/yr; P = .09).
The Canadian Trial of Physiologic Pacing (CTOPP) randomized 2568 patients from a general pacemaker population, 42% with SND as an indication for pacing but without permanent AF, to receive a ventricular (1474) or atrial-based (1094) pacemaker.50 Over a mean follow-up of 3.1 years, overall mortality was similar in both groups (6.3%/yr in physiologic vs. 6.6%/yr in ventricular pacing group; P = 0.92). Since the effects of atrial pacing for prevention of AF were delayed in the CTOPP population, and similar observations were reported by Danish investigators,48 the follow-up in CTOPP was extended. Over 6.4 years, the primary composite outcome of cardiovascular death or stroke occurred at 6.1%/year in patients assigned to ventricular pacing and 5.5%/year in those assigned to physiologic pacing.51 The relative risk reduction was 8.1% with physiologic pacing (95% CI = −6.5 to 20.7; P = 0.26).
The Mode Selection Trial (MOST) investigators randomized 2010 patients with SND to rate-modulated ventricular or dual-chamber pacing.52 Over a mean follow-up of 2.76 years, annual mortality was similar in both groups: 7.1% in the physiologic versus 7.4% in the ventricular pacing group (P = 0.65) (Fig. 13-5).
A pooled analysis of five randomized trials did not show a significant reduction in mortality (hazard ratio [HR] = 0.95; 95% CI = 0.87 to 1.03) associated with atrial or dual-chamber pacing versus ventricular pacing.53 In a population-based cohort of 8777 patients with SND treated with AAI or DDD pacemakers, a slight increase in all-cause mortality (HR = 1.12; CI = 1.00 to 1.25), was seen among DDD patients compared to AAI patients.54
Pacing and Atrial Fibrillation
Paroxysmal AF, atrial flutter, and atrial tachycardia (AT) occur frequently in SND.55 AT/AF detection and data storage features are now present in most dual-chamber pacemakers, facilitating the diagnosis and treatment of AF.55–61 Some devices have special algorithms developed specifically for the prevention and management of atrial tachyarrhythmias.58–61
Detection of Atrial Fibrillation
Accurate detection of atrial tachyarrhythmias, including atrial flutter and AF, by implantable devices with advanced AT management features is important for several reasons. Accurate detection ensures the appropriateness of automatic mode switching and of atrial antitachycardia pacing (ATP) for atrial flutter. Many implantable devices provide a wide range of diagnostic data, including the frequency of AT/AF, time of onset and duration of episodes, quantity of AT/AF (burden expressed as % of day or hours/day), ventricular rate control during AF, and symptomatic events56–58 (see Fig. 13-5). Such information may be valuable for managing antiarrhythmic drug therapy62–64 and when considering the need for antithrombotic therapy.65,66 Patients with AF associated with episodes of rapid ventricular rates are more likely to experience hospitalization for cardiovascular events63 (Fig. 13-6). Identification of such episodes by an implanted cardiac device can prompt changes in antiarrhythmic drug therapy.
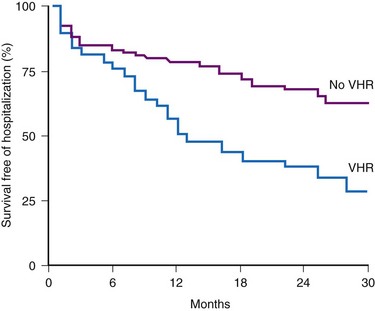
Figure 13-6 Survival free from hospitalization for cardiovascular symptoms.
(From Willems R, Morck ML, Exner DV, et al: Ventricular high-rate episodes in pacemaker diagnostics identify a high-risk subgroup of patients with tachy-brady syndrome. Heart Rhythm 1:414-421, 2004.)
With advances in remote monitoring of pacemakers and implantable defibrillators, early detection of AT may facilitate earlier medical intervention.67 Many recent clinical device trials have used device-based indices of arrhythmia recurrence, frequency, and burden as surrogates for clinical endpoints.56 Although measures of AT/AF burden in devices is quite accurate, counts of AT/AF frequency are less accurate because of variations in device-based criteria for detection, termination, and redetection, as well as intermittent atrial undersensing during AT/AF episodes.68,69
Some investigators report comparatively low values for appropriate AT/AF episode detection using early-generation pacemaker mode-switching and detection algorithms.70 Table 13-3 summarizes factors influencing AT/AF underdetection or overdetection. It is important that pacemakers be programmed to optimize detection of AT/AF, with careful attention to the atrial sensitivity and post–ventriculoatrial blanking period. Atrial undersensing from inappropriate noise reversion has been described when high atrial sensing levels are programmed.71 Atrial lead position is an important factor for appropriate AT/AF detection because some sites (e.g., near coronary sinus os, within RA appendage) are associated with a high incidence of far-field R-wave (FFRW) oversensing that may be inappropriately classified as AF. Figure 13-7 shows an example of inappropriate classification caused by FFRW oversensing. Interelectrode lead spacing (e.g., 5 mm) may also minimize FFRW sensing.
Sensing | Factors |
---|---|
Undersensing |
AT/AF, Atrial tachycardia/fibrillation; EGM, electrogram.
Attention to these issues promotes high sensitivity and specificity of AT/AF detection.72 Applying these parameters, sensitivity of AT/AF detection was 97% for sustained episodes of 5 minutes or longer.73 Some devices have incorporated newer detection algorithms that include pattern recognition to facilitate detection of AT/AF and minimize inappropriate detection caused by FFRW oversensing.72,73 Such algorithms have high sensitivity (>95%) and specificity for AT/AF detection.72–75
Pacing Mode in Prevention of Atrial Fibrillation
Several prospective randomized clinical trials have reported that atrial or dual-chamber pacing prevents paroxysmal and permanent AF in patients with symptomatic bradycardia as the primary indication for cardiac pacing. Danish investigators reported a 46% relative risk reduction for the development of AF in the atrial pacing group (P = .012).48 The CTOPP study reported an 18% risk reduction in the development of AF (P = .05) in patients randomized to atrial-based versus ventricular pacing.50,76 This effect was sustained over longer-term follow-up (20% risk reduction at 6 years; P = .009).51 Patients with a structurally normal heart were most likely to derive benefit from atrial-based pacing.76 Although a retrospective subgroup analysis in CTOPP suggested that pacemaker-dependent patients were most likely to benefit from physiologic pacing for prevention of AF, this effect was not confirmed over long-term follow-up.51 The PASE investigators reported a 32% reduction in AF in patients with SND as the primary indication for pacing who were randomized to the dual-chamber pacing mode versus the ventricular pacing mode (P = .06).49 MOST reported a 21% relative risk reduction in the development of AF (P = .008) and a 56% relative risk reduction in the development of permanent AF (P < .001) in patients randomized to dual-chamber versus ventricular pacing52 (Fig. 13-8).
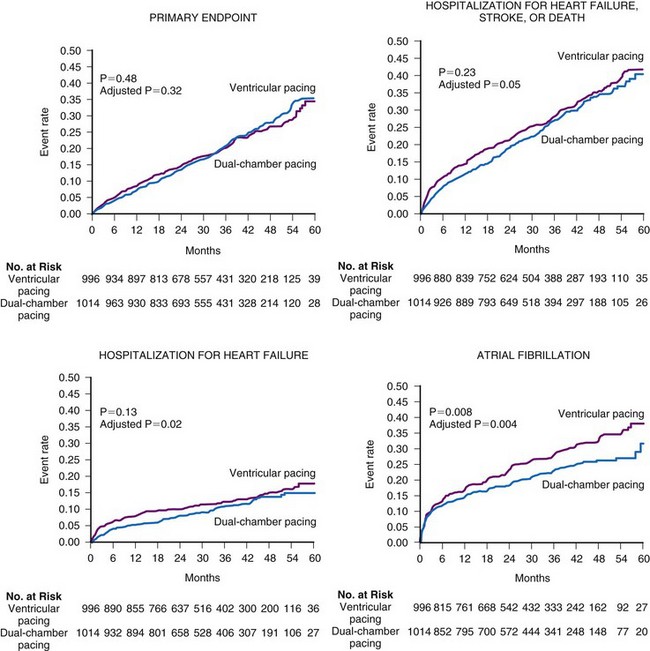
Figure 13-8 Rates of clinical events according to the mode of pacing in the MOST trial.
(From Lamas GA, Lee KL, Sweeney MO, et al: Mode Selection Trial in Sinus Node Dysfunction. Ventricular pacing or dual-chamber pacing for sinus node dysfunction. N Engl J Med 346:1854-1862, 2002.)
Table 13-4 summarizes the results of these clinical trials. In contrast, the United Kingdom Pacing and Cardiovascular Events (UKPACE) trial investigators, who randomized 2021 patients age 70 and older with high-grade AV block to dual-chamber or ventricular pacing, did not observe a benefit of dual-chamber pacing for AF.77 These data suggest that the primary benefit of dual-chamber pacing for prevention of AF occurs in patients with SND.
In the CTOPP population, the number needed to treat (NNT) to prevent any AF over 10 years (ideal longevity of dual-chamber pacemaker) was nine patients.58 In the MOST population, NNT to prevent permanent AF in patients with SND over 3 years was nine patients. The cost differential of dual-chamber pacing compared with ventricular pacing in CTOPP was $2500.78 The additional cost of dual-chamber pacing is approximately $1/day. AF is frequently unrecognized in the pacemaker population; anticoagulation is often underutilized; and class I-III antiarrhythmic drug therapy for prevention of AF may be proarrhythmic. Accordingly, atrial or dual-chamber pacing may be a cost-effective therapy to prevent a condition that causes substantial morbidity.78,79 However, to date, no study has shown that prevention of AF in this patient population translates into a substantial clinical benefit, whether improved quality of life or functional capacity or reduced hospitalization or health care utilization.
Pacing Algorithms in Prevention of Atrial Fibrillation
Over the past decade, a number of clinical studies have evaluated the role of atrial pacing algorithms for prevention of atrial fibrillation.58,59,80 Initial studies investigated the efficacy of atrial overdrive pacing achieved by programming a higher base pacing rate on suppression of AF.81,82 Subsequently, selective pacing algorithms were designed to target the triggers and substrate for AF, including continuous pacing rather than fixed-rate atrial overdrive, algorithms to prevent pauses after atrial premature beats or exercise, transient overdrive pacing after detection of premature atrial contractions (PACs) or after termination of an episode of AF, and atrial ATP for termination of atrial flutter that transitions into AF.58,60,61 Although small studies suggested promise of one or more of these algorithms for suppression of AF, larger randomized clinical trials have failed to demonstrate a major benefit of these therapies for prevention of AF compared to control groups with fixed-rate or rate-responsive pacing.
To test the hypothesis that atrial pacing might prevent paroxysmal AF in patients without symptomatic bradycardia as a primary indication for pacing, the Atrial Pacing Peri-Ablation for the Prevention of AF (PA3) study randomized 97 patients with frequent paroxysmal AF being considered for AV junction ablation to a trial of atrial pacing versus no pacing.81 The time to first recurrence of AF and total AF burden documented over 3-month follow-up were similar in the atrial pacing group (1.9 days; 95% CI = 0.8 to 4.6) compared to the no pacing group (4.2 days; 95% CI = 1.8 to 9.5; P = NS). In Phase II the PA3 trial tested the hypothesis that atrial pacing versus AV synchrony would prevent AF.82 After AV junction ablation, 76 patients were randomized to DDDR versus VDD pacing. The time to first recurrence of sustained AF and total AF burden over time were similar in the DDDR (0.37 day; 95% CI = 0.1 to 1.3) and the VDD (0.5 day; 95% CI, 0.2 to 1.7; P = NS) pacing groups. Furthermore, AF burden increased progressively over time, and 42% of the study population had developed permanent AF within 1 year after ablation. Patients maintained on constant antiarrhythmic drug therapy throughout the PA3 study developed significant increases in AF burden and were more likely to develop permanent AF very early after AV junction ablation than patients with deferred ablation.83
The dynamic atrial overdrive (DAO) pacing algorithm was evaluated in the Atrial Dynamic Overdrive Pacing Trial (ADOPT).84 Investigators randomized 399 patients with SND and paroxysmal AF to a trial of DDDR pacing versus DDDR pacing plus the overdrive atrial pacing algorithm. Patients were followed at 1, 3, and 6 months after pacemaker insertion. The primary study outcome was symptomatic AF burden, defined as percentage of days in symptomatic AF. Both groups experienced a substantial reduction in symptomatic AF over time. In addition, a modest but statistically significant reduction was found in symptomatic AF associated with the DAO algorithm during follow-up (Fig. 13-9, upper panel). However, the absolute risk reduction diminished over time: 1.25% at 1 month versus 0.36% at 6 months. Moreover, overall AF burden calculated from the mode-switch diagnostics was similar between the two groups and indeed, increased over time, suggesting that the overdrive pacing algorithm had no effect on total AF burden over time (Fig. 13-9, lower panel).
Other studies have not demonstrated a significant impact of DAO pacing for prevention of AF. The Atrial Septal Pacing Clinical Efficacy Trial (ASPECT) randomized 298 patients with paroxysmal AF and associated symptomatic bradycardia to conventional DDDR pacing or DDDR pacing plus three additional atrial pacing algorithms specifically designed for prevention of AF at atrial-septal or right atrial appendage pacing sites.85 After 3 months of treatment, patients were crossed-over to the alternate pacing strategy and followed for an additional 3 months. AF burden, determined from the diagnostic counters (in pacemaker measured as % time in AF) was the primary study outcome. The three AF prevention algorithms (atrial pacing preference, atrial rate stabilization, and “post mode switch overdrive” pacing) did not reduce AF burden or AF frequency despite suppression of atrial premature beat frequency (Fig. 13-10).
The AFTherapy Study randomized 372 patients with drug-refractory paroxysmal AF to dual-chamber pacing programmed to single-rate or rate-responsive pacing at lower rates of either 70 or 85 bpm or to a control group with single-rate pacing at 40 bpm.60 In the subsequent preventive pacing phase, patients were randomized to pacing at a lower rate of 70 bpm with or without programming of four preventive pacing algorithms. The primary endpoint was AF burden, with secondary endpoints of time to first AF episode and averaged sinus rhythm duration. Substantial data were excluded from the analysis because of atrial-sensing issues, predominantly oversensing, resulting in inappropriate classification of events as AF. In the conventional pacing phase, no significant differences in AF burden were found between the various lower rates and the control group or between single-rate and rate-responsive pacing. In the second phase, patients receiving preventive pacing therapies had a similar AF burden (median, 0 hr/day) compared with patients receiving conventional pacing (0.18 hr/day; P = 0.47).
In the Study of Atrial Fibrillation Reduction (SAFARI) trial, 240 patients with qualifying AF 4 months after pacemaker implantation were randomized to a suite of six pacing prevention therapies programmed on or off and followed for 6 months.61 A modest but statistically significant decrease in AF burden (median, 0.08 hr/day) was seen in the group randomized to AF prevention therapies on versus no change in AF burden in off group. Patients with a high baseline burden of AF (>1.44 hr/day) randomized to prevention therapies on experienced a significant reduction in AF burden (median, 1.38 hr/day), versus an increase in AF burden of 0.31 hr/day in the subgroup with high baseline AF burden randomized to therapies off.
A study based on characteristics preceding onset of AF derived from pacemaker diagnostic data analyzed whether subgroups of patients benefited from pace prevention therapies. During a 3-month diagnostic phase with conventional pacing, Lewalter et al.86 identified a substrate group (>70% of AF episodes preceded by <2 PACs before AF onset) and a trigger group (those with ≤70% of AF episodes with <2 PACs before AF onset). In the trigger group, pacing algorithms were programmed to avoid or prevent atrial premature beats, and in the substrate group, continuous atrial overdrive pacing was enabled. In the trigger group (n = 73), AF burden was reduced 28% (median AF burden: 2.06 hr/day during monitoring vs. 1.49 hr/day with pacing therapies “on” (P = .03) in association with reduced atrial premature beat frequency. AF burden was unchanged in the substrate group (median AF burden: 1.82 hr/day during monitoring vs. 2.38 hr/day with overdrive pacing “on” (P = 0.12). Thus, individualizing AF pace prevention algorithms based on individual AF onset characteristics may be beneficial in some patients.
Pacing Algorithms in Termination of Atrial Fibrillation
Episodes of atrial tachycardia and atrial flutter occur frequently in patients with AF, and AT or atrial flutter often transition between episodes of AF.73,74,87,88 Figure 13-11 illustrates an example of AF that organizes into atrial flutter and is then effectively terminated by atrial ATP therapy, which has been incorporated into some pacemakers and implantable defibrillators. Efficacy of atrial ATP for termination of AT and atrial flutter ranges from 30% to 54%.87,89–93 In select individuals, atrial ATP therapy reduces AT/AF burden over time.60,90,93
The hypothesis that successful pace termination of AT or atrial flutter would prevent AF over time was tested in the Atrial Therapy Efficacy and Safety Trial (ATTEST).89 In a parallel design, 370 patients received a Medtronic AT 500 and were randomized to DDDR pacing or DDDR pacing with atrial ATP therapies and atrial pace prevention therapies programmed on. Over 3-month follow-up, 15,000 episodes of AT were treated by atrial ATP therapies, with device-classified efficacy of 41%. However, AF frequency and AF burden were not reduced by the delivery of prevention therapies or atrial ATP therapies (Fig. 13-12). Friedman et al.94 randomized 405 patients with a history of AF and an implantable cardioverter-defibrillator (ICD, for standard indications) to atrial prevention and termination therapies on (n = 199) or off (n = 206). Patients were followed for 7 months. The mean AT/AF burden was 4.3 ± 20.0 hr/month in patients with AT/AF prevention and termination therapies on versus 9.0 ± 50.0 hr/month in the off group (P = 0.11).
Several factors may explain the discrepancy between the reported high atrial ATP efficacy for AT termination and the failure to demonstrate a significant reduction in AT/AF burden.57 Device-classified efficacy may be exaggerated because of spontaneous termination of many episodes of AT.74 Indeed, in ATTEST89 and GEM-III-AT clinical evaluation,74 approximately half of episodes classified as AT/AF lasted less than 10 minutes. By design, these devices define ATP “efficacy” if sinus or atrial paced rhythm occurs before redetection of AT/AF. Although the redetection time is usually less than 1 minute, these devices could allow up to 3 minutes from the last delivered ATP therapy for redetection to occur. Using a more conservative definition of efficacy—termination of AT or AF within 20 seconds of delivery of atrial ATP therapy—atrial ATP efficacy is lower than previously reported, terminating only 26% of all atrial tachyarrhythmias and 32% of AT episodes. These observations have led to changes in detection algorithms in newer devices. The incorporation of 50-Hz burst pacing algorithms into atrial defibrillators has not been shown to terminate AF.74
In the studies evaluating atrial ATP efficacy for AF prevention, not all patients were maintained on class I-III antiarrhythmic therapy, which may be important in facilitating ATP therapy and preventing early recurrence of AT/AF.95,96 Some episodes of AT are not reentrant, and some episodes classified as “AT” may have been AF that could not be pace-terminated.97 Certain patients do benefit from atrial ATP therapy for prevention of AF62,93 (see Fig. 13-11). Patients with high atrial ATP efficacy for termination of AT (>60% of all treated episodes effectively terminated) experience a significant reduction in AF burden98 (Fig. 13-13). As many as 30% of patients with SND and paroxysmal AF may benefit from atrial ATP therapy. Also, more aggressive programming of atrial ATP therapy than using the nominal values in these devices may increase therapy efficacy.99
Most studies comparing AF pacing algorithms in AF prevention have conducted relatively short-term follow-up. Comparing atrial ATP therapy, we combined atrial ATP and atrial pace prevention algorithms to conventional DDDR pacing for prevention of AF over 3 years of follow-up in 71 patients with AT/AF after pacemaker insertion.100 AT/AF burden remained stable over 3 years in the DDDR and ATP and prevention groups, but increased significantly over time in the ATP group (Fig. 13-14). Patients not receiving class I-III antiarrhythmic therapy were more likely to experience an increase in AT/AF burden over time. Furthermore, atrial ATP is not always effective and in some patients could be proarrhythmic. Therefore, again, if atrial ATP therapies are programmed on, their effectiveness needs to be reevaluated over time.
Site-Specific Atrial Pacing for Atrial Fibrillation Prevention
Clinical and experimental studies have demonstrated that septal pacing, dual-site RA pacing, and biatrial pacing reduce total atrial conduction times and dispersion of atrial refractoriness.101–103 In the cardiac surgery population, randomized trials report that RA, dual-site RA, and biatrial pacing prevent postoperative AF.104 In the pacemaker population, several clinical trials have evaluated the efficacy of selective atrial pacing sites for AF prevention. Patients randomized to pacing at the interatrial septum (Bachmann bundle) were less likely to develop permanent AF (47%) than those randomized to pacing in RA appendage (75%; P < .05).105 Figure 13-15 shows chest x-ray images of the atrial lead location in the septum at the Bachmann bundle.
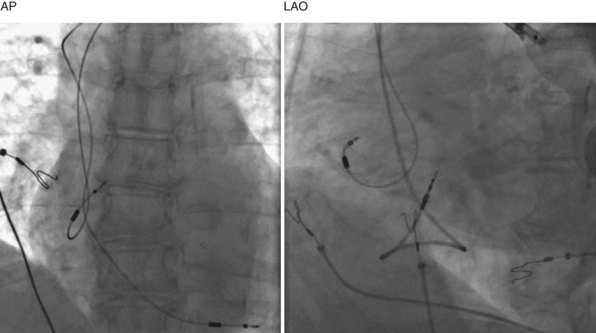
Figure 13-15 Chest radiographs showing atrial lead positioned on right atrial septum near Bachmann bundle.
(Courtesy Ken E. Ellenbogen.)
In a small series of patients, Padaletti et al.106 reported a significant reduction in AF frequency and AF burden in patients randomized to pacing near the triangle of Koch compared to those paced in the RA appendage. However, other studies have not confirmed these benefits.85,107 In the largest randomized trial conducted to date comparing RA appendage pacing to atrial-septal pacing, a significant reduction in AF frequency or AF burden was not observed over short-term follow-up in the two groups85 (see Fig. 13-10). Dual-site RA pacing, RA appendage and coronary sinus os, lead locations have been reported to confer a modest benefit for AF prevention compared to RA appendage pacing alone.108 The benefit of dual-site RA pacing was greatest in patients treated with antiarrhythmic drugs. Biatrial pacing has been reported to prevent paroxysmal AF and development of permanent AF in patients with marked intra-atrial conduction delays,109 perhaps secondary to atrial hemodynamic benefits associated with biatrial pacing.110
At present, the role of selective atrial lead site for prevention of AF in the pacemaker population remains uncertain.111 A hybrid approach, including aggressive antiarrhythmic therapy combined with dual-site RA pacing and RA linear ablation, has been reported to provide effective AF rhythm control in select patients.112 Nevertheless, a single-site atrial lead location would be preferable given the added expense and complexity of additional leads.
Small studies incorporating AF pace prevention algorithms with septal or dual-site pacing locations also have not been shown to prevent AF.113–115 The Septal Pacing for Atrial Fibrillation Suppression Evaluation (SAFE) study is currently evaluating whether low atrial-septal pacing compared to RA appendage pacing with or without atrial overdrive pacing will suppress AF116 (NCT 00419640). A total enrollment of 380 patients with a follow-up of at least 3 years is planned. The primary endpoint is the development of persistent AF. A similar trial in progress, RESPECT (CT 00289289), is also evaluating atrial pacing from the septum versus RA appendage (enrollment >400 patients), with the primary endpoint of symptom frequency and secondary endpoint of need for cardioversion.
Pacing and Stroke
Thromboembolism secondary to AF occurs in SND. In the Danish study, thromboembolic events were significantly less likely to occur in the atrial (12%; 2.1%/yr) than in the ventricular (23%; 4.3%/yr) pacing group (P = .023).48 In the Danish study, use of anticoagulation therapy was minimal. However, the larger clinical trials failed to show a benefit of atrial pacing for prevention of cerebrovascular accident (CVA, stoke). In CTOPP, annual incidence of stroke was similar in the atrial (1.0%) and the ventricular (1.1%) pacing groups.50,51 In MOST, annual CVA incidence was similar in the dual-chamber (1.5%) and the ventricular (1.8%) pacing groups.52,117
The failure to show a benefit of reducing stroke by preventing AF in these larger trials may be caused by other factors. The etiology of stroke in an elderly pacemaker population is multifactorial, not only secondary to emboli originating in the left atrium because of AF.118 Furthermore, the impact of randomized clinical trials of anticoagulation for prevention of stroke may have led to increased use of antithrombotic therapy in pacemaker patients with AF. In MOST, 72% of patients were receiving antiplatelet therapy or warfarin. A pooled analysis of the five largest randomized trials comparing atrial to ventricular pacing did demonstrate a significant reduction in stroke with atrial pacing (HR = 0.81; CI = 0.67 to 0.99; P = 0.038), which was similar among patients with SND and AV block.53
Relationship Between Stroke Risk and Atrial Fibrillation Burden/Duration
Analysis from clinical trials suggests that patients with intermittent AF are at similar risk for stroke as those with persistent or permanent AF. Analysis of AF data retrieved from device telemetry in several cohort studies suggested that longer-lasting AT/AF events were associated with an increased risk of arterial thromboembolism.65,66 Importantly, in a significant number of pacemaker patients, relapse of AF lasting more than 48 hours occurred in the absence of symptoms.119 Data from 725 patients with pacemakers capable of monitoring AT/AF burden showed that AT/AF lasting 24 hours or longer conferred a threefold increased risk of systemic thromboembolism compared with patients with no AT/AF or with AT/AF episodes lasting less than 24 hours.65
The TRENDS study designed a prospective observational cohort to assess the relationship between low levels of AF burden and the risk of stroke.66 Patients with one or more risk factors for stroke and a pacemaker or ICD that monitored AT/AF daily were followed for a mean of 1.4 years. AT/AF burden was defined as the longest total AT/AF duration on any given day during a 30-day window. The varying exposure was updated daily and related to the development of systemic thromboembolism during follow-up. The annualized thromboembolic risk during follow-up of 2486 patients was 1.1% for zero AT/AF burden and 1.1% for low and 2.4% for high burden subsets. These data suggest that systemic thromboembolism is a quantitative function of AT/AF burden, and that AT/AF burden longer than 5.5 hours on any day of the 30 days appeared to double the risk of systemic thromboembolism. The TRENDS data did not allow the investigators to define a safe AT/AF burden threshold that confers a risk no greater than that of patients with zero AF burden.
The Asymptomatic Atrial Fibrillation and Stroke Evaluation in Pacemaker Patients and the Atrial Fibrillation Reduction Atrial Pacing Trial (ASSERT) is a multicenter cohort follow-up study in elderly hypertensive patients with a recently implanted pacemaker and no prior history of AF.120 The study tests the hypothesis that AT/AF detected by pacemaker diagnostics during follow-up predicts an increased risk of CVA and other vascular events, with results expected soon. Preliminary results were presented in November 2010 at the American Heart Association Meeting. ASSERT showed that device-detected atrial tachyarrhythmias were associated with an increased risk of stroke and systemic embolism (2.5x).
Pacing and Pacemaker Syndrome
The pacemaker syndrome consists of a constellation of signs and symptoms that result from the loss of AV synchrony during ventricular pacing2,121 (Table 13-5). The definition and diagnostic criteria of pacemaker syndrome vary, but symptoms include fatigue, dyspnea on exertion, paroxysmal nocturnal dyspnea, orthopnea, orthostatic hypotension, and syncope. In the MOST study, pacemaker syndrome was prospectively defined as (1) new or worsened dyspnea, orthopnea, elevated jugular venous pressure, rales, and edema, with ventriculoatrial conduction during ventricular pacing, or (2) symptoms of dizziness, weakness, presyncope or syncope, and reduced systolic blood pressure (>20 mm Hg) during VVIR pacing compared with atrial pacing or sinus rhythm.52,121 The incidence of pacemaker syndrome was 13.8% at 6 months, 16.0% at 1 year, 17.7% at 2 years, 19.0% at 3 years, and 19.7% at 4 years. Univariate predictors of pacemaker syndrome were a higher percentage of ventricular paced beats, a higher programmed lower pacemaker rate, and a slower underlying sinus heart rate. However, only a higher percentage of paced beats was an independent predictor of developing pacemaker syndrome. Quality of life (using various metrics) decreased with the diagnosis of pacemaker syndrome and improved after the pacemaker was reprogrammed to a physiologic mode.121 In the PASE study, of the 204 patients randomized to VVIR, 26% crossed over to DDDR for intolerance to ventricular pacing.49 The Danish study reported a 2% incidence of pacemaker syndrome;48 in CTOPP it was 2.7% at 3 years.50 The incidence of pacemaker syndrome was likely underestimated in these latter two trials, because treatment would have required a surgical intervention. Pacemaker syndrome possibly was overestimated in the MOST and PASE studies, because it was simple to cross patients over to the DDDR pacing mode.121
Degree | Symptoms |
---|---|
Mild |
Pacing and Congestive Heart Failure
In the Danish study, New York Heart Association (NYHA) functional class and diuretic use were significantly higher during follow-up in the ventricular than in the atrial pacing group 48. In CTOPP, the annual incidence of hospitalization for CHF was similar in the ventricular (3.5%) and atrial (3.1%) pacing groups (relative risk reduction, 7.9%; 95% CI = −18.5 to 28.3%; P = 0.52).50 In MOST the annual incidence of hospitalization for CHF was similar in patients receiving dual-chamber (3.7%) or ventricular (4.4%) pacing (HR = 0.82; 95% CI = 0.63 to 1.06; P = 0.13).52 During follow-up, patients receiving dual-chamber pacing had a lower heart failure score than patients receiving ventricular pacing (average points/ follow-up visit: ventricular, 1.75; dual chamber, 1.49; P < .001).
Pacing and Quality of Life
Dual-chamber pacing is associated with improved physiologic parameters, including cardiac output, exercise capacity, and exercise oxygen consumption (Vo2), compared with ventricular pacing.118 However, none of the large clinical trials conducted to date has demonstrated substantial improvements in quality of life (QOL) measures associated with atrial-based pacing compared to ventricular pacing.122 The PASE study did not demonstrate an improvement in QOL measures in patients treated with dual-chamber pacing compared to ventricular pacing, although there was a modest improvement in some SF-36 scales at 3 months of follow-up in the subgroup with SND.49 In MOST, dual-chamber pacing resulted in a small but measurable increase in QOL compared with ventricular pacing.52 In MOST, AF development after pacemaker insertion did not appear to be a major QOL determinant. In CTOPP, QOL improved after pacemaker implantation in both atrial pacing and ventricular pacing groups, but no significant health-related QOL difference was observed.122 ADEPT did not observe an improvement in QOL measures associated with rate-adaptive pacing in patients with chronotropic incompetence.123 Thus, atrial-based pacing may confer some modest improvements in quality of life in patients with SND, particularly the group at risk for pacemaker syndrome.49
Potential Detrimental Effects of Ventricular Pacing
Although some randomized trials studying pacing mode selection for patients with sinus node disease have reported no clinical benefit of DDDR pacing over VVIR pacing, post hoc analyses from MOST have reported an association between a high percentage of right ventricular (RV) apical pacing and worse clinical outcomes, including an increased risk for AF and heart failure hospitalization.124 This raises concern that the beneficial effects of maintaining AV synchrony may have been mitigated by the deleterious effects of RV apical pacing.
Risk of Atrial Fibrillation
The MOST investigators reported that ventricular pacing adversely influenced the development of AF, independent of AV synchrony.124 Patients programmed to DDDR were more likely to be paced in the ventricle (90%) than patients programmed to VVIR (58%; P = .001). Patients more frequently paced in the ventricle were more likely to develop AF. The risk of developing AF increased approximately 1% for each 1% increase in ventricular pacing (Fig. 13-16). Nielsen et al.125 also reported adverse effects of AV sequential pacing compared to atrial pacing on the development of AF. They randomized 177 patients with sick sinus syndrome to AAIR pacing, DDDR pacing with a short (≤150 msec) or a long (300 msec) AV interval. AF during follow-up was significantly less common in the AAIR group (7.4%) than either the DDDR-short (23.3%) or the DDDR-long (17.5%) AV delay group (P = 0.03).126 The impact of ventricular pacing after AV junction ablation on AF burden was evaluated in patients participating in the PA3 study who remained on stable antiarrhythmic drug therapy throughout follow-up.82 Before ablation, AF burden was 3.0 ± 1.2 hr/day and increased to 10.4 ± 2.2 and 11.8 ± 2.3 hr/day in the two follow-up periods (P < .05). These data suggest that the proarrhythmic effect of ventricular pacing occurs rapidly in patients with AF.
Risk of Heart Failure
In MOST, ventricular pacing more than 40% of the time in the DDDR group was associated with a 2.6-fold risk of hospitalization for CHF, whereas pacing more than 80% in VVIR was associated with a 2.5-fold risk of hospitalization124 (see Fig. 13-16). The DAVID trial randomized 506 patients who had received a dual-chamber ICD to dual-chamber rate-responsive pacing at 70 bpm or ventricular backup pacing at 40 bpm.127 Only 12% of this study population had severely symptomatic heart failure. The primary study outcome measure, death or hospitalization for new or worsened heart failure, was lower in the ventricular paced group compared to the dual-chamber paced group (relative hazard 1.61, range 1.06-2.44; P ≤ .03). The rate of hospitalization at 1 year for CHF was 13.3% in the ventricular group versus 22.6% in the dual-chamber group. Patients with left ventricular (LV) dysfunction who were more frequently paced in the ventricle were more likely to experience an adverse outcome.128 Patients with DDDR RV pacing greater than 40% had worse outcomes than the VVI backup group.
The Pacing to Avoid Cardiac Enlargement (PACE) study was designed to test the hypothesis that atrial-synchronized biventricular pacing (BiVP) is superior to RV apical pacing in preserving LV systolic function and avoiding adverse LV remodeling in patients with a normal left ventricular ejection fraction (LVEF) and standard indications for pacing.129 Patients (177) in whom a biventricular pacemaker had been successfully implanted were randomly assigned to receive BiVP (89) or RV apical pacing (88). More than 40% of the study population had SND as the primary indication for pacing. The primary endpoints were LVEF and left ventricular end-systolic volume (LFESV). At 12 months, mean LVEF was significantly lower in the RV pacing group than the BiVP group (54.8 ± 9.1% vs. 62.2 ± 7.0%; P < .001) and LVESV was significantly higher in the RV group than the BiVP group (35.7 ± 16.3 mL vs. 27.6 ± 10.4 mL; P < .001). There were no differences in hospital admissions for heart failure during follow-up between the two groups. Although interesting, the data do not generally apply to the population with SND. By study design, short AV intervals were programmed, which forced a high proportion of RV apical pacing.
The abnormal effects of RV apical pacing have been attributed to the abnormal electrical and mechanical activation pattern of the ventricles.130 Table 13-6 summarizes adverse effects of RV apical pacing on electrical and mechanical function, including changes in cardiac metabolism and perfusion, remodeling, and hemodynamics. Myocardial perfusion defects may be present in up to 65% of patients after long-term RV apical pacing and are mainly located near the pacing site. RV pacing may also result in structural changes and LV remodeling, including mitochondrial variations and degenerative fibrosis. Changes in LV wall thickness, LV remodeling, functional mitral regurgitation, and left atrial remodeling may occur during RV apical pacing. Hemodynamic properties and global mechanical function may be affected by the abnormal electrical and mechanical activation of the left ventricle. Pacing at the RV apex may result in a decrease in cardiac output and may alter LV filling properties. Changes in myocardial strain and timing of regional strain have been reported in an animal model of cardiac pacing. Prinzen et al.131 reported a significant decrease in strain in the regions close to the pacing site and an increase in myocardial strain in remote regions. The timing of peak regional strain considered to be “mechanical dyssynchrony” is also altered during pacing. Together, these changes may contribute to decreased LV systolic function, decreased functional capacity, and ultimately progression to symptomatic heart failure. Functional mitral regurgitation may lead to adverse atrial electrical remodeling, creating a substrate for AF. Figure 13-17 illustrates a conceptual model of the potential detrimental effects of RV apical pacing.
Functional Change | Effects |
---|---|
Ventricular electrical activation delays |
Algorithms to Minimize Ventricular Pacing
Based on the concern about the potential adverse consequences of frequent RV apical pacing, algorithms have been introduced to minimize ventricular pacing, including search AV hysteresis, managed ventricular pacing (MVP), and AAIsafeR mode.132–139 These algorithms substantially reduced the amount of ventricular pacing, from 90% to 1% in select patient populations, including those with SND.132–136
Search AV Hysteresis
Search AV hysteresis operates in the DDDR, DDIR, DVIR, or VDD modes.134,135 A programmable maximum AV offset or extension is applied to the programmed sensed and paced AV intervals. The maximum operational AV intervals can range from 40 to 600 msec based on the programmed AV intervals and the maximum increase in AV interval programmed. Search AV mode continuously adapts the operating AV interval to a value slightly longer than the AV conduction time (≈30 msec). If 8 of 16 ventricular paced events occur at maximum sensed or paced AV intervals, these intervals will return to the programmed values. A conduction check is then automatically performed 30 minutes later; it will progressively double, up to 16 hours, if the subsequent AV conduction check does not uncover intrinsic AV conduction. If 10 consecutive AV conduction failures occur at 16 hours, the algorithm will disable, and the device will revert to the DDD mode. In search AV hysteresis, the maximum sensed AV interval shortens when the atrial rate exceeds 80 bpm and is 150 msec at 120 bpm or higher.
Managed Ventricular Pacing
The MVP algorithm operates in the AAIR mode, with backup DDDR pacing if AV conduction fails.132–134 In the AAIR mode, if AV conduction fails for one beat, a ventricular backup pacing stimulus is delivered 80 msec after the next atrial beat to avoid long pauses (Fig. 13-18). If AV conduction fails for two of four successive atrial sensed or paced events, the device switches to the DDDR mode. Automatic conduction checks are then performed by the inhibition of one ventricular paced event. The first conduction check occurs 1 minute after switching to the DDDR mode. If intrinsic AV conduction occurs, the algorithm reverts to the AAIR mode. The interval for conduction checks progressively doubles (2, 4, 8 minutes) up to 16 hours; checks then occur every 16 hours. The MVP mode does not automatically disable.
AAIsafeR Mode
The AAIsafeR mode operates in the AAIR mode and switches to the DDDR mode when certain criteria are met.136–138 Mode switching from AAIR to DDDR occurs if at least seven intervals between atrial paced or sensed events and ventricular sensed events exceed a predefined limit (programmable between 350 and 450 msec; AV block I criterion). If 3 of 12 sensed or paced atrial events are not followed by a ventricular sensed event, mode switching from AAIR to DDDR occurs (AV block II criterion). Two consecutive atrial events without consecutive ventricular sensed events results in mode switching (AV block III criterion). To avoid prolonged pauses, DDDR pacing occurs if a programmable duration of ventricular asystole is detected (programmable between 2 and 4 seconds) independent of the relationship between atrial and ventricular events.
These pacing algorithms have substantially reduced the percent ventricular pacing in both patients with SND and those with intermittent AV block, although the magnitude of benefit is less than in patients with SND133–136 (Fig. 13-19). Importantly, many patients with AV block as the primary indication for pacing experience intermittent AV block, and intrinsic AV conduction is preserved much of the time.133–135 Thus, these algorithms should be considered for patients with intermittent AV block.
Clinical Outcomes
The Search AV Extension and Managed Ventricular Pacing for Promoting Atrioventricular Conduction (SAVE PACe) trial tested the hypothesis that a strategy of minimizing ventricular pacing would reduce the risk of persistent AF better than conventional dual-chamber pacing.139 Patients with SND (n = 1065), intact AV conduction, and a normal QRS interval were randomized to conventional DDDR pacing or DDDR pacing with algorithms to minimize ventricular pacing (search AV or MVP) programmed “on.” The AV interval was programmed between 120 and 180 msec for patients randomized to conventional DDDR pacing. Patients were followed for 1.7 ± 1.0 years, when the study was stopped because it had met the primary outcome. The median percent ventricular pacing was lower in the group assigned to minimal ventricular pacing (9.1%) compared to the conventional DDDR group (99%). The percent atrial pacing was similar in both groups. During follow-up, persistent AF developed in 68 of 535 patients assigned to conventional DDDR pacing (12.7%) versus 40 of 530 in the group assigned to minimal ventricular pacing (7.9%; P = .004 by log rank test). A 40% reduction in the relative risk of developing persistent AF was seen with the strategy of minimizing ventricular pacing. No differences in mortality, hospitalization for heart failure, or cardioversion for AF were observed between the two groups. Although this study supports a strategy of minimizing ventricular pacing for prevention of AF, the magnitude of benefit has been overestimated by the study design. Most centers programming “conventional” DDDR pacing would select longer AV delays (usually >200 msec). The study design actually imposed a higher degree of ventricular pacing in the DDDR mode than would be traditionally expected with individualized programming of AV delays in pacemaker follow-up programs.
Is One Mode for Minimizing Ventricular Pacing Superior?
Clinical studies report that MVP mode offers greater reduction in the percent ventricular pacing than search AV mode, except for patients with permanent AV block.134,135 Figure 13-20 shows ventricular pacing in MVP versus search AV mode in 322 patients followed for 1 month. MVP was associated with a significant reduction in percent ventricular pacing for all categories of AV conduction except permanent AV block. Whether such differences translate into improved clinical outcomes remains unknown.
Complications and Clinical Nuances
Overall, these algorithms have been reported to be safe and well tolerated.132–139 However, depending on device programming, prolonged AV delays of up to 600 msec may occur. These long AV delays cannot be considered physiologic and may result in pacemaker syndrome, with atrial contraction occurring early in diastole.140 This may be particularly detrimental for patients with heart failure or mitral regurgitation. Examples of varying AV delays and pauses followed by backup ventricular pacing associated with MVP mode are shown in Figure 13-18. Although the MVP mode relies primarily on atrial pacing, timing cycles are based on ventricular intervals. In the case of a ventricular premature beat, noncompetitive atrial pacing will extend the ventriculoatrial (VA) interval, resulting in an extension of the next atrial pacing interval.141 This results in atrial pacing rates below the programmed lower rate limit. Abrupt changes in ventricular cycle length (short-long-short) sequences have been reported to facilitate ventricular tachyarrhythmia onset in patients with implantable defibrillators. Such short-long-short sequences may be observed with the MVP mode and may constitute a form of ventricular proarrhythmia142 (Fig. 13-21). Short-long-short sequences may also be observed with traditional DDDR programming preceding VT. Cases of torsade de pointes VT have been reported in pacemaker patients attributed to prolonged pauses secondary to programming in the MVP mode.143,144 Based on these observations, the MVP mode should not be considered in patients with long QT syndrome or those with permanent complete heart block.
Treatment of Sinus Node Disease
Pacing Modalities
Table 13-7 shows the indications for pacing in the patient with SND.145 Although none of the large, randomized clinical trials have shown a survival benefit of atrial-based pacing, dual-chamber pacemakers are often implanted in North America.1 Atrial or dual-chamber pacing may be considered in patients with SND for prevention of AF and pacemaker syndrome.48,145 However, prevention of AF in this population has not been demonstrated to reduce stroke or mortality.
Class | Indications |
---|---|
I | Sinus node dysfunction with documented symptomatic bradycardia, including sinus pauses |
Symptomatic chronotropic incompetence | |
Symptomatic sinus bradycardia secondary to required drug therapy | |
IIa | Sinus node dysfunction with heart rate <40 bpm without a clear association between symptoms and documented bradycardia |
Syncope of unknown origin when evidence of sinus node dysfunction discovered or provoked during electrophysiologic study | |
IIb | Minimally symptomatic, with heart rate <40 bpm while awake |
III | Sinus node dysfunction in asymptomatic patients |
Symptoms suggestive of bradycardia clearly documented in absence of bradycardia | |
Symptomatic bradycardia secondary to nonessential drug therapy |
Class I: General consensus that pacing is indicated; Class II: divergence of opinion on need for pacing; Class III: general consensus that pacing is not indicated.
Figure 13-22 shows a decision tree on pacing mode selection for patients with SND.146 It is important to remember that many patients with SND have intrinsic AV conduction and do not require ventricular pacing most of the time. Consequently, considering strategies that minimize ventricular pacing, such as AAIR pacing when clinically indicated, programming long AV delays to minimize ventricular pacing, using algorithms to minimize RV apical pacing, or even backup ventricular pacing at a low rate, may be sufficient for most patients with SND. Backup ventricular pacing may be effective for the patient with infrequent pauses and normal chronotropic response. In patients with an implantable defibrillator, AAI pacing showed no advantage over backup VVI pacing.147
AAIR Pacing
As many as 20% of patients with symptomatic SND are potential candidates for AAIR pacing systems.1,48 A rate-adaptive pulse generator should be considered because of the high incidence of chronotropic incompetence in patients with SND.146 Although this is the most economical approach to providing atrial pacing in this population, AAIR mode is used for less than 1% of implants in North America.1 Concerns about progression of AV block and the development of chronic AF likely explain the low utilization of AAIR in SND patients. However, these concerns appear to be unfounded if patients are carefully selected.148,149 The risk of progression to AV block is less than 1% per year if patients have normal AV conduction and no intraventricular conduction delays on the surface electrocardiogram (ECG) at implant. The likelihood of developing permanent AF is small (<1.5 %/yr) if patients are under age 70 at implant, have no history of paroxysmal AF,148,149 and show no evidence of marked intra-atrial conduction delays.150
Table 13-8 lists the contraindications to AAIR pacing. Given the concerns that dual-chamber pacing may result in unnecessary ventricular pacing that promotes the development of AF and CHF, higher utilization of AAIR pacing should be considered in patients with SND. A cost-effectiveness analysis performed in the MOST population showed that during the first 4 years of the trial, dual-chamber pacemakers increased quality-adjusted life expectancy by 0.013 year per subject, at an incremental cost-effectiveness ratio of $53,000 per quality-adjusted year of life gained. This cost could be further reduced by increasing the use of AAIR over DDDR pacing in select patients.79
Type | Contraindications |
---|---|
Absolute |
AV, Atrioventricular; IVCD, intraventricular conduction delay.
Rate-Adaptive Pacing
Chronotropic incompetence is common in SND, and rate-adaptive pacing is beneficial in select patients. ADEPT evaluated whether dual-chamber rate-modulated pacing, compared with dual-chamber pacing alone, improved QOL in 872 patients with documented chronotropic incompetence.123 At 6 months, patients randomized to DDDR had a higher peak exercise heart rate (113.3 ± 19.6 bpm) than those randomized to DDD (101.1 ± 21.1 bpm; P < .0001). However, at 1 year, there were no significant differences between groups randomized to DDD versus DDDR with respect to specific activity scale or the secondary QOL endpoints.
Alternate Ventricular Pacing Sites
There has been some debate about the optimal ventricular pacing site for minimizing the risk of heart failure or AF in patients receiving dual-chamber pacemakers.151 However, it is important to remember that the vast majority of patients with SND have intrinsic AV conduction the vast majority of the time. Accordingly, strategies to minimize ventricular pacing whenever possible should be pursued, including the judicious use of AAI pacing, optimal use of AV search hysteresis, and use of algorithms that switch from AAI to DDD pacing when atrial conduction fails.146 Clinical studies have demonstrated that the amount of ventricular pacing can be substantially reduced with these newer pacing algorithms, even in patients with AV block as the primary indication for pacing.132–138 No data to date suggest that biventricular pacing prevents heart failure in patients without significant systolic dysfunction.152 Right ventricular outflow tract and high RV septal pacing sites may be associated with shorter ventricular activation times and less ventricular dyssynchrony than RV apical pacing.151,153,154 However, no large clinical trials have shown that any of these pacing sites are superior to the traditional RV apical pacing site. Clinical trials evaluating alternate RV pacing sites are in progress.
Biologic Pacemakers
Advances in the development of biologic pacemakers are underway, and progress in this field has recently been reviewed.155 Suppression of Kir2.1 channels has unmasked latent pacemaker activity in ventricular cells. Overexpression of HCN2 channels has elicited ectopic pacemaker activity in a canine model. Subepicardial injection of HCN2-overexpressing human mesenchymal stem cells into canine left ventricle induced spontaneous rhythms in animals with chronic AV block. In this model, the pacemaking activity required 10 to 12 days to become stable, then persisted for at least 6 weeks. In contrast to the direct cell injection approaches, fusion technology allows implantation of biologic pacemakers at a specific site. Cardiac stem cells may be viable candidates for biologic pacemakers, and work in this field is underway. Although this field is evolving rapidly, much works needs to be done before biologic pacemakers become reality (see Chapter 7).
1 Mond HG, Irwin M, Morillo C, Ector H. The world survey of cardiac pacing and cardioverter-defibrillators: calendar year 2001. Pacing Clin Electrophysiol. 2004;27:955-964.
2 Gillis AM. Pacing for sinus node disease: indications, techniques and clinical trials. In: Ellenbogen KA, Kay GW, Wilkoff BL, editors. Clinical cardiac pacing and defibrillation. ed 3. Philadelphia: Saunders; 2007:407-427.
3 Dobrzynski H, Boyett MR, Anderson RH. New insights into pacemaker activity: promoting understanding of sick sinus syndrome. Circulation. 2007;115:1921-1932.
4 Chandler NJ, Greener ID, Tellez JO, et al. Molecular architecture of the human sinus node: insights into the function of the cardiac pacemaker. Circulation. 2009;119:1562-1575.
5 Verkerk AO, van Ginneken AC, Wilders R. Pacemaker activity of the human sinoatrial node: role of the hyperpolarization-activated current, If. Int J Cardiol. 2009;132:318-336.
6 Jones SA, Lancaster MK, Boyett MR. Aging-related changes of connexins and conduction within the sinoatrial node. J Physiol. 2004;560(Pt 2):429-437.
7 Ludwig A, Herrmann S, Hoesl E, Stieber J. Mouse models for studying pacemaker channel function and sinus node arrhythmia. Prog Biophys Mol Biol. 2008;98:179-185.
8 Schulze-Bahr E, Neu A, Friederich P, et al. Pacemaker channel dysfunction in a patient with sinus node disease. J Clin Invest. 2003;111:1537-1545.
9 Stieber J, Hofmann F, Ludwig A. Pacemaker channels and sinus node arrhythmia. Trends Cardiovasc Med. 2004;14:23-28.
10 Ueda K, Nakamura K, Hayashi T, et al. Functional characterization of a trafficking-defective HCN4 mutation, D553N, associated with cardiac arrhythmia. J Biol Chem. 2004;279:27194-27198.
11 Verkerk AO, Wilders R, Coronel R, et al. Ionic remodeling of sinoatrial node cells by heart failure. Circulation. 2003;108:760-766.
12 Veldkamp MW, Wilders R, Baartscheer A, et al. Contribution of sodium channel mutations to bradycardia and sinus node dysfunction in LQT3 families. Circ Res. 2003;92:976-983.
13 Ruan Y, Liu N, Priori SG. Sodium channel mutations and arrhythmias. Nat Rev Cardiol. 2009;6:337-348.
14 Lei M, Huang CL, Zhang Y. Genetic Na+ channelopathies and sinus node dysfunction. Prog Biophys Mol Biol. 2008;98:171-178.
15 Benson DW, Wang DW, Dyment M, et al. Congenital sick sinus syndrome caused by recessive mutations in the cardiac sodium channel gene (SCN5A). J Clin Invest. 2003;112:1019-1028.
16 Hund TJ, Mohler PJ. Ankyrin-based targeting pathway regulates human sinoatrial node automaticity. Channels (Austin). 2008;2:404-406.
17 Le Scouarnec S, Bhasin N, Vieyres C, et al. Dysfunction in ankyrin-B-dependent ion channel and transporter targeting causes human sinus node disease. Proc Natl Acad Sci USA. 2008;105:15617-15622.
18 Lees-Miller JP, Guo J, Somers JR, et al. Selective knockout of mouse ERG1 B potassium channel eliminates IKr in adult ventricular myocytes and elicits episodes of abrupt sinus bradycardia. Mol Cell Biol. 2003;23:1856-1862.
19 Demolombe S, Lande G, Charpentier F, et al. Transgenic mice overexpressing human KvLQT1 dominant-negative isoform. Part I. Phenotypic characterisation. Cardiovasc Res. 2001;50:314-327.
20 Hu K, Qu Y, Yue Y, Boutjdir M. Functional basis of sinus bradycardia in congenital heart block. Circ Res. 2004;94:e32-e38.
21 Platzer J, Engel J, Schrott-Fischer A, et al. Congenital deafness and sinoatrial node dysfunction in mice lacking class D L-type Ca2+ channels. Cell. 2000;102:89-97.
22 Zhang Z, Xu Y, Song H, et al. Functional Roles of Ca(v)1, 3 (α1D) calcium channel in sinoatrial nodes: insight gained using gene-targeted null mutant mice. Circ Res. 2002;90:981-987.
23 Gillis AM, Kavanagh KM, Mathison HJ, et al. Heart block in mice overexpressing calcineurin but not NF-AT3. Cardiovasc Res. 2004;64:488-495.
24 Opthof T, Coronel R, Rademaker HM, et al. Changes in sinus node function in a rabbit model of heart failure with ventricular arrhythmias and sudden death. Circulation. 2000;101:2975-2980.
25 Hong CS, Cho MC, Kwak YG, et al. Cardiac remodeling and atrial fibrillation in transgenic mice overexpressing junctin. FASEB J. 2002;16:1310-1312.
26 Du Y, Huang X, Wang T, et al. Downregulation of neuronal sodium channel subunits Nav1, 1 and Nav1, 6 in the sinoatrial node from volume-overloaded heart failure rat. Pflugers Arch. 2007;454:451-459.
27 Zicha S, Fernández-Velasco M, Lonardo G, et al. Sinus node dysfunction and hyperpolarization-activated (HCN) channel subunit remodeling in a canine heart failure model. Cardiovasc Res. 2005;66:472-481.
28 Stiles MK, Brooks AG, Roberts-Thomson KC, et al. High-density mapping of the sinus node in humans: role of preferential pathways and the effect of remodeling. J Cardiovasc Electrophysiol. 2010;21:532-539.
29 Sanders P, Morton JB, Kistler PM, et al. Electrophysiological and electroanatomic characterization of the atria in sinus node disease: evidence of diffuse atrial remodeling. Circulation. 2004;109:1514-1522.
30 Kistler PM, Sanders P, Fynn SP, et al. Electrophysiologic and electroanatomic changes in the human atrium associated with age. J Am Coll Cardiol. 2004;44:109-116.
31 Sanders P, Kistler PM, Morton JB, et al. Remodeling of sinus node function in patients with congestive heart failure: reduction in sinus node reserve. Circulation. 2004;110:897-903.
32 Sanders P, Morton JB, Davidson NC, et al. Electrical remodeling of the atria in congestive heart failure: electrophysiological and electroanatomic mapping in humans. Circulation. 2003;108:1461-1468.
33 Sparks PB, Mond HG, Vohra JK, et al. Electrical remodeling of the atria following loss of atrioventricular synchrony: a long-term study in humans. Circulation. 1999;100:1894-1900.
34 Sparks PB, Jayaprakash S, Vohra JK, Kalman JM. Electrical remodeling of the atria associated with paroxysmal and chronic atrial flutter. Circulation. 2000;102:1807-1813.
35 Kalman JM, Sparks PB. Electrical remodeling of the atria as a consequence of atrial stretch. J Cardiovasc Electrophysiol. 2001;12:51-55.
36 Manios EG, Kanoupakis EM, Mavrakis HE, et al. Sinus pacemaker function after cardioversion of chronic atrial fibrillation: is sinus node remodeling related with recurrence? J Cardiovasc Electrophysiol. 2001;12:800-806.
37 Hadian D, Zipes DP, Olgin JE, Miller JM. Short-term rapid atrial pacing produces electrical remodeling of sinus node function in humans. J Cardiovasc Electrophysiol. 2002;13:584-586.
38 Hocini M, Sanders P, Deisenhofer I, et al. Reverse remodeling of sinus node function after catheter ablation of atrial fibrillation in patients with prolonged sinus pauses. Circulation. 2003;108:1172-1175.
39 Raitt MH, Kusumoto W, Giraud G, McAnulty JH. Reversal of electrical remodeling after cardioversion of persistent atrial fibrillation. J Cardiovasc Electrophysiol. 2004;15:507-512.
40 Yeh YH, Burstein B, Qi XY, et al. Funny current downregulation and sinus node dysfunction associated with atrial tachyarrhythmia: a molecular basis for tachycardia-bradycardia syndrome. Circulation. 2009;119:1576-1585.
41 Krahn AD, Klein GJ, Yee R, Skanes AC. Randomized Assessment of Syncope Trial: conventional diagnostic testing versus a prolonged monitoring strategy. Circulation. 2001;104:46-51.
42 Krahn AD, Klein GJ, Yee R, et al. Cost implications of testing strategy in patients with syncope: Randomized Assessment of Syncope Trial. J Am Coll Cardiol. 2003;42:495-501.
43 Solano A, Menozzi C, Maggi R, et al. Incidence, diagnostic yield and safety of the implantable loop-recorder to detect the mechanism of syncope in patients with and without structural heart disease. Eur Heart J. 2004;25:1116-1119.
44 Menozzi C, Brignole M, Alboni P, et al. The natural course of untreated sick sinus syndrome and identification of the variables predictive of unfavorable outcome. Am J Cardiol. 1998;82:1205-1209.
45 Brandt J, Anderson H, Fahraeus T, Schuller H. Natural history of sinus node disease treated with atrial pacing in 213 patients: implications for selection of stimulation mode. J Am Coll Cardiol. 1992;20:633-639.
46 Jahangir A, Shen WK, Neubauer SA, et al. Relation between mode of pacing and long-term survival in the very elderly. J Am Coll Cardiol. 1999;33:1208-1216.
47 Connolly SJ, Kerr C, Gent M, Yusuf S. Dual-chamber versus ventricular pacing: critical appraisal of current data. Circulation. 1996;94:578-583.
48 Andersen HR, Nielsen JC, Thomsen PEB, et al. Long-term follow-up of patients from a randomized trial of atrial versus ventricular pacing for sick-sinus syndrome. Lancet. 1997;350:1210-1216.
49 Lamas GA, Orav EJ, Stambler BS, et al. Quality of life and clinical outcomes in elderly patients treated with ventricular pacing as compared with dual-chamber pacing. Pacemaker Selection in the Elderly Investigators. N Engl J Med. 1998;338:1097-1104.
50 Connolly SJ, Kerr CR, Gent M, et al. CTOPP Investigators. Effects of physiologic pacing versus ventricular pacing on the risk of stroke and death due to cardiovascular causes. Canadian Trial of Physiological Pacing. N Engl J Med. 2000;342:1385-1391.
51 Kerr CR, Connolly SJ, Abdollah H, et al. Canadian Trial of Physiological Pacing: effects of physiological pacing during long-term follow-up. Circulation. 2004;109:357-362.
52 Lamas GA, Lee KL, Sweeney MO, et al. Mode Selection Trial in Sinus Node Dysfunction. Ventricular pacing or dual-chamber pacing for sinus node dysfunction. N Engl J Med. 2002;346:1854-1862.
53 Healey JS, Toff WD, Lamas GA, et al. Cardiovascular outcomes with atrial-based pacing compared with ventricular pacing: meta-analysis of randomized trials, using individual patient data. Circulation. 2006;114:11-17.
54 Fored CM, Granath F, Gadler F, et al. Atrial vs. dual-chamber cardiac pacing in sinus node disease: a register-based cohort study. Europace. 2008;10:825-831.
55 Gillis AM, Morck M. Atrial fibrillation after DDDR pacemaker implantation. J Cardiovasc Electrophysiol. 2002;13:542-547.
56 Gillis AM. Rhythm control in atrial fibrillation: endpoints for device-based trials. Heart Rhythm. 2004;1:B52-B57.
57 Gillis AM. Pacing to prevent atrial fibrillation. Cardiol Clin. 2000;18:25-36.
58 Gillis AM. Clinical trials of pacing for maintenance of sinus rhythm. J Interv Card Electrophysiol. 2004;10(Suppl 1):55-62.
59 Ellenbogen KA. Pacing therapy for prevention of atrial fibrillation. Heart Rhythm. 2007;4(Suppl 3):84-87.
60 Camm AJ, Sulke N, Edvardsson N, et al. AFTherapy Investigators. Conventional and dedicated atrial overdrive pacing for the prevention of paroxysmal atrial fibrillation: the AFTherapy study. Europace. 2007;9:1110-1118.
61 Gold MR, Adler S, Fauchier L, et al. SAFARI Investigators. Impact of atrial prevention pacing on atrial fibrillation burden: primary results of the Study of Atrial Fibrillation Reduction (SAFARI) trial. Heart Rhythm. 2009;6:295-301.
62 Gillis AM, Morck M, Fitts S. Antitachycardia pacing therapies and arrhythmia monitoring diagnostics for the treatment of atrial fibrillation. Can J Cardiol. 2002;18:992-995.
63 Willems R, Morck ML, Exner DV, et al. Ventricular high-rate episodes in pacemaker diagnostics identify a high-risk subgroup of patients with tachy-brady syndrome. Heart Rhythm. 2004;1:414-421.
64 Boriani G, Padeletti L, Santini M, et al. Rate control in patients with pacemaker affected by brady-tachy form of sick sinus syndrome. Heart J. 2007;154:193-200.
65 Capucci A, Santini M, Padeletti L, et al. Italian AT500 Registry Investigators. Monitored atrial fibrillation duration predicts arterial embolic events in patients suffering from bradycardia and atrial fibrillation implanted with antitachycardia pacemakers. J Am Coll Cardiol. 2005;46:1913-1920.
66 Glotzer TV, Daoud EG, Wyse DG, et al. The relationship between daily atrial tachyarrhythmia burden from implantable device diagnostics and stroke risk: the TRENDS study. Circ Arrhythm Electrophysiol. 2009;2:474-480.
67 Ricci RP, Morichelli L, Santini M. Remote control of implanted devices through home monitoring technology improves detection and clinical management of atrial fibrillation. Europace. 2009;11:54-61.
68 De Simone A, Senatore G, Turco P, et al. Specificity of atrial mode switching in detecting atrial fibrillation episodes: roles of length and contiguity. Pacing Clin Electrophysiol.. 2005;28(Suppl 1):47-49.
69 Van Hemel NM, van de Bos AA, Koïstinen J, Fast JH. Verification of pacemaker automatic mode switching for the detection of atrial fibrillation and atrial tachycardia with Holter recording. Europace. 2006;8:950-961.
70 Passman RS, Weinberg KM, Freher M, et al. Accuracy of mode switch algorithms for detection of atrial tachyarrhythmias. J Cardiovasc Electrophysiol. 2004;15:773-777.
71 Kolb C, Halbfass P, Zrenner B, Schmitt C. Paradoxical atrial undersensing due to inappropriate atrial noise reversion of atrial fibrillation in dual-chamber pacemakers. J Cardiovasc Electrophysiol. 2005;16:696-700.
72 Purerfellner H, Gillis AM, Holbrook R, Hettrick DA. Accuracy of atrial tachyarrhythmia detection in implantable devices with arrhythmia therapies. Pacing Clin Electrophysiol. 2004;27:983-992.
73 Fitts SM, Hill MR, Mehra R, Gillis AM. High rate atrial tachyarrhythmia detections in implantable pulse generators: low incidence of false-positive detections. The PA3 Clinical Trial Investigators. Pacing Clin Electrophysiol. 2000;23:1080-1086.
74 Gillis AM, Unterberg-Buchwald C, Schmidinger H, et al. GEM III AT Worldwide Investigators. Safety and efficacy of advanced atrial pacing therapies for atrial tachyarrhythmias in patients with a new implantable dual-chamber cardioverter-defibrillator. J Am Coll Cardiol. 2002;40:1653-1659.
75 Kouakam C, Kacet S, Hazard JR, et al. Ventak AV Investigators. Performance of a dual-chamber implantable defibrillator algorithm for discrimination of ventricular from supraventricular tachycardia. Europace. 2004;6:32-42.
76 Skanes AC, Krahn AD, Yee R, et al. Canadian Trial of Physiologic Pacing. Progression to chronic atrial fibrillation after pacing: the Canadian Trial of Physiologic Pacing. CTOPP Investigators. J Am Coll Cardiol. 2001;38:167-172.
77 Toff WD, Camm AJ, Skehan JD. United Kingdom Pacing and Cardiovascular Events Trial Investigators. Single-chamber versus dual-chamber pacing for high-grade atrioventricular block. N Engl J Med. 2005;353:145-155.
78 Gillis AM, Kerr CR. Whither physiologic pacing? Implications of CTOPP. PACE. 2000;23(8):1193-1196.
79 Rinfret S, Cohen DJ, Lamas GA, et al. Cost-effectiveness of dual-chamber pacing compared with ventricular pacing for sinus node dysfunction. Circulation. 2005;111:165-172.
80 Gillis AM. Selective pacing algorithms for prevention of atrial fibrillation: the final chapter? Heart Rhythm. 2009;6:295-301.
81 Gillis AM, Wyse DG, Connolly SJ, et al. Atrial pacing peri-ablation for prevention of paroxysmal atrial fibrillation. Circulation. 1999;99:2553-2558.
82 Gillis AM, Connolly SJ, Lacombe P, et al. PA3 Investigators. A randomized crossover comparison of DDDR versus VDD pacing post-AV node ablation for prevention of atrial fibrillation. Circulation. 2000;102:736-741.
83 Willems R, Wyse DG, Gillis AM. Atrial Pacing Periablation for Paroxysmal Atrial Fibrillation (PA3) Study Investigators. Total AV nodal ablation increases atrial fibrillation burden in patients with paroxysmal AF despite continuation of antiarrhythmic drug therapy. J Cardiovasc Electrophysiol. 2003;14:1296-1301.
84 Carlson MD, Ip J, Messenger J, et al. ADOPT Investigators. A new pacemaker algorithm for the treatment of atrial fibrillation: results of the Atrial Dynamic Overdrive Pacing Trial (ADOPT). J Am Coll Cardiol. 2003;42:627-633.
85 Padeletti L, Purerfellner H, Adler SW, et al. Worldwide ASPECT Investigators. Combined efficacy of atrial septal lead placement and atrial pacing algorithms for prevention of paroxysmal atrial tachyarrhythmia. J Cardiovasc Electrophysiol. 2003;14:1189-1195.
86 Lewalter T, Yang A, Pfeiffer D, et al. Individualized selection of pacing algorithms for the prevention of recurrent atrial fibrillation: Results from the VIP registry. Pacing Clin Electrophysiol. 2006;29:124-134.
87 Israel CW, Hugl B, Unterberg C, et al. Pace-termination and pacing for prevention of atrial tachyarrhythmias: results from a multicenter study with an implantable device for atrial therapy. J Cardiovasc Electrophysiol. 2001;12:1121-1128.
88 Israel CW, Ehrlich JR, Gronefeld G, et al. Prevalence, characteristics and clinical implications of regular atrial tachyarrhythmias in patients with atrial fibrillation: insights from a study using a new implantable device. J Am Coll Cardiol. 2001;38:355-363.
89 Lee MA, Weachter R, Pollak S, et al. ATTEST Investigators. The effect of atrial pacing therapies on atrial tachyarrhythmia burden and frequency: results of a randomized trial in patients with bradycardia and atrial tachyarrhythmias. J Am Coll Cardiol. 2003;41:1926-1932.
90 Friedman PA, Dijkman B, Warman EN, et al. Atrial therapies reduce atrial arrhythmia burden in defibrillator patients. Circulation. 2001;104:1023-1028.
91 Adler SW2nd, Wolpert C, Warman EN, et al. Efficacy of pacing therapies for treating atrial tachyarrhythmias in patients with ventricular arrhythmias receiving a dual-chamber implantable cardioverter defibrillator. Circulation. 2001;104:887-892.
92 Stephenson EA, Casavant D, Tuzi J, et al. ATTEST Investigators. Efficacy of atrial antitachycardia pacing using the Medtronic AT500 pacemaker in patients with congenital heart disease. Am J Cardiol. 2003;92:871-876.
93 Israel CW, Gronefeld G, Li YG, Hohnloser SH. Usefulness of atrial pacing for prevention and termination of atrial tachyarrhythmias in a patient with persistent atrial fibrillation. Pacing Clin Electrophysiol. 2002;25:1527-1529.
94 Friedman PA, Ip JH, Jazayeri M, et al. RID-AF Investigators. The impact of atrial prevention and termination therapies on atrial tachyarrhythmia burden in patients receiving a dual-chamber defibrillator for ventricular arrhythmias. J Interv Card Electrophysiol. 2004;10:103-110.
95 Camm AJ, Savelieva I. Rationale and patient selection for “hybrid” drug and device therapy in atrial and ventricular arrhythmias. J Interv Card Electrophysiol. 2003;9:207-214.
96 Murgatroyd FD. “Pills and pulses”: hybrid therapy for atrial fibrillation. J Cardiovasc Electrophysiol. 2002;13:S40-S46.
97 Konings KT, Kirchhof CJ, Smeets JR, et al. High-density mapping of electrically induced atrial fibrillation in humans. Circulation. 1994;89:1665-1680.
98 Gillis AM, Koehler J, Morck M, et al. High atrial antitachycardia pacing therapy efficacy is associated with a reduction in atrial tachyarrhythmia burden in a subset of patients with sinus node dysfunction and paroxysmal atrial fibrillation. Heart Rhythm. 2005;2:791-796.
99 Hugl B, Israel CW, Unterberg C, et al. AT500 Verification Study Investigators. Incremental programming of atrial anti-tachycardia pacing therapies in bradycardia-indicated patients: effects on therapy efficacy and atrial tachyarrhythmia burden. Europace. 2003;5:403-409.
100 Gillis AM, Morck M, Exner DV, et al. Impact of atrial antitachycardia pacing and atrial pace prevention therapies on atrial fibrillation burden over long-term follow-up. Europace. 2009;11:1041-1047.
101 Yu WC, Chen SA, Tai CT, et al. Effects of different atrial pacing modes on atrial electrophysiology: implicating the mechanism of biatrial pacing in prevention of atrial fibrillation. Circulation. 1997;96:2992-2996.
102 Prakash A, Delfaut P, Krol RB, Saksena S. Regional right and left atrial activation patterns during single- and dual-site atrial pacing in patients with atrial fibrillation. Am J Cardiol. 1998;82:1197-1204.
103 Papageorgiou P, Anselme F, Kirchhof CJ, et al. Coronary sinus pacing prevents induction of atrial fibrillation. Circulation. 1997;96:1893-1898.
104 Crystal E, Connolly SJ, Sleik K, et al. Interventions on prevention of postoperative atrial fibrillation in patients undergoing heart surgery: a meta-analysis. Circulation. 2002;106:75-80.
105 Bailin SJ, Adler S, Giudici M. Prevention of chronic atrial fibrillation by pacing in the region of Bachmann’s bundle: results of a multicenter randomized trial. J Cardiovasc Electrophysiol. 2001;12:912-917.
106 Padeletti L, Porciani MC, Michelucci A, et al. Interatrial septum pacing: a new approach to prevent recurrent atrial fibrillation. J Interv Card Electrophysiol. 1999;3:35-43.
107 Hermida JS, Kubala M, Lescure FX, et al. Atrial septal pacing to prevent atrial fibrillation in patients with sinus node dysfunction: results of a randomized controlled study. Am Heart J. 2004;148:312-317.
108 Saksena S, Prakash A, Ziegler P, et al. Improved suppression of recurrent atrial fibrillation with dual-site right atrial pacing and antiarrhythmic drug therapy. J Am Coll Cardiol. 2002;40:1140-1150.
109 D’Allonnes GR, Pavin D, Leclercq C, et al. Long-term effects of biatrial synchronous pacing to prevent drug-refractory atrial tachyarrhythmia: a nine-year experience. J Cardiovasc Electrophysiol. 2000;11:1081-1091.
110 Doi A, Takagi M, Toda I, et al. Acute haemodynamic benefits of biatrial atrioventricular sequential pacing: comparison with single atrial atrioventricular sequential pacing. Heart. 2004;90:411-418.
111 Gammage MD, Marsh AM. Randomized trials for selective site pacing: do we know where we are going? Pacing Clin Electrophysiol. 2004;27:878-882.
112 Madan N, Saksena S. Long-term rhythm control of drug-refractory atrial fibrillation with “hybrid therapy” incorporating dual-site right atrial pacing, antiarrhythmic drugs, and right atrial ablation. Am J Cardiol. 2004;93:569-575.
113 Hermida JS, Kubala M, Lescure FX, et al. Atrial septal pacing to prevent atrial fibrillation in patients with sinus node dysfunction: results of a randomized controlled study. Am Heart J. 2004;148:312-317.
114 De Simone A, Senatore G, Donnici G, et al. Dynamic and dual-site atrial pacing in the prevention of atrial fibrillation: The STimolazione Atrial DInamica Multisito (STADIM) Study. Pacing Clin Electrophysiol. 2007;30(Suppl 1):71-74.
115 Spitzer SG, Wacker P, Gazarek S, et al. PASTA Study Group. Primary prevention of atrial fibrillation: does the atrial lead position influence the incidence of atrial arrhythmias in patients with sinus node dysfunction? Results from the PASTA Trial. Pacing Clin Electrophysiol. 2009;32:1553-1561.
116 Lau CP, Wang CC, Ngarmukos T, et al. SAFE Study Steering Committee for SAFE Study Group. A prospective randomized study to assess the efficacy of rate and site of atrial pacing on long-term development of atrial fibrillation. J Cardiovasc Electrophysiol. 2009;20:1020-1025.
117 Greenspon AJ, Hart RG, Dawson D, et al. MOST Study Investigators. Predictors of stroke in patients paced for sick sinus syndrome. J Am Coll Cardiol. 2004;43:1617-1622.
118 Lamas GA, Ellenbogen KA. Evidence base for pacemaker mode selection: from physiology to randomized trials. Circulation. 2004;109:443-451.
119 Israel CW, Grönefeld G, Ehrlich JR, et al. Long-term risk of recurrent atrial fibrillation as documented by an implantable monitoring device: implications for optimal patient care. J Am Coll Cardiol. 2004;43:47-52.
120 Hohnloser SH, Capucci A, Fain E, et al. ASSERT Investigators and Committees. ASymptomatic atrial fibrillation and Stroke Evaluation in pacemaker patients and the atrial fibrillation Reduction atrial pacing Trial (ASSERT). Am Heart J. 2006;152:442-447.
121 Link MS, Hellkamp AS, Estes NA3rd, et al. MOST Study Investigators. High incidence of pacemaker syndrome in patients with sinus node dysfunction treated with ventricular-based pacing in the Mode Selection Trial (MOST). J Am Coll Cardiol. 2004;43:2066-2071.
122 Newman D, Lau C, Tang AS, et al. CTOPP Investigators. Effect of pacing mode on health-related quality of life in the Canadian Trial of Physiologic Pacing. Am Heart J. 2003;145:430-437.
123 Lamas GA, Knight JD, Sweeney MO, et al. Impact of rate-modulated pacing on quality of life and exercise capacity: evidence from the Advanced Elements of Pacing Randomized Controlled Trial (ADEPT). Heart Rhythm. 2007;4:1125-1132.
124 Sweeney MO, Hellkamp AS, Ellenbogen KA, et al. Mode Selection Trial Investigators. Adverse effect of ventricular pacing on heart failure and atrial fibrillation among patients with normal baseline QRS duration in a clinical trial of pacemaker therapy for sinus node dysfunction. Circulation. 2003;107:2932-2937.
125 Nielsen JC, Kristensen L, Andersen HR, et al. A randomized comparison of atrial and dual-chamber pacing in 177 consecutive patients with sick sinus syndrome: echocardiographic and clinical outcome. J Am Coll Cardiol. 2003;42:614-623.
126 Kristensen L, Nielsen JC, Mortensen PT, et al. Incidence of atrial fibrillation and thromboembolism in a randomised trial of atrial versus dual chamber pacing in 177 patients with sick sinus syndrome. Heart. 2004;90:661-666.
127 Wilkoff BL, Cook JR, Epstein AE, et al. DAVID Trial Investigators. Dual-chamber pacing or ventricular backup pacing in patients with an implantable defibrillator: the Dual Chamber and VVI Implantable Defibrillator (DAVID) Trial. JAMA. 2002;288:3115-3123.
128 Sharma AD, Rizo-Patron C, Hallstrom AP, et al. DAVID Investigators. Percent right ventricular pacing predicts outcomes in the DAVID trial. Heart Rhythm. 2005;2:830-834.
129 Yu CM, Chan JY, Zhang Q, et al. Biventricular pacing in patients with bradycardia and normal ejection fraction. N Engl J Med. 2009;361:2123-2134.
130 Tops LF, Schalij MJ, Bax JJ. The effects of right ventricular apical pacing on ventricular function and dyssynchrony implications for therapy. J Am Coll Cardiol. 2009;54:764-776.
131 Prinzen FW, Hunter WC, Wyman BT, McVeigh ER. Mapping of regional myocardial strain and work during ventricular pacing: experimental study using magnetic resonance imaging tagging. J Am Coll Cardiol. 1999;33:1735-1742.
132 Sweeney MO, Ellenbogen KA, Casavant D, et al. Multicenter prospective randomized safety and efficacy study of a new atrial-based managed ventricular pacing mode (MVP) in dual chamber ICDs. J Cardiovasc Electrophysiol. 2005;16:811-817.
133 Gillis AM, Pürerfellner H, Israel CW, et al. Medtronic Enrhythm Clinical Study Investigators. Reducing unnecessary right ventricular pacing with the managed ventricular pacing mode in patients with sinus node disease and AV block. Pacing Clin Electrophysiol. 2006;29:697-705.
134 Pürerfellner H, Brandt J, Israel C, et al. Comparison of two strategies to reduce ventricular pacing in pacemaker patients. Pacing Clin Electrophysiol. 2008;31:167-176.
135 Murakami Y, Tsuboi N, Inden Y, et al. Difference in percentage of ventricular pacing between two algorithms for minimizing ventricular pacing: results of the IDEAL RVP (Identify the Best Algorithm for Reducing Unnecessary Right Ventricular Pacing) study. Europace. 2010;12:96-102.
136 Savouré A, Fröhlig G, Galley D, et al. A new dual-chamber pacing mode to minimize ventricular pacing. Pacing Clin Electrophysiol. 2005;28(Suppl 1):43-46.
137 Pioger G, Leny G, Nitzsché R, Ripart A. AAIsafeR limits ventricular pacing in unselected patients. Pacing Clin Electrophysiol. 2007;30(Suppl 1):S66-S70.
138 Xue-Jun R, Zhihong H, Ye W, et al. Clinical comparison between a new dual-chamber pacing mode-AAIsafeR and DDD mode. Am J Med Sci. 2010;339:145-147.
139 Sweeney MO, Bank AJ, Nsah E, et al. Search AV Extension and Managed Ventricular Pacing for Promoting Atrioventricular Conduction (SAVE PACe) Trial. Minimizing ventricular pacing to reduce atrial fibrillation in sinus node disease. N Engl J Med. 2007;357:1000-1008.
140 Pascale P, Pruvot E, Graf D. Pacemaker syndrome during managed ventricular pacing mode: what is the mechanism? J Cardiovasc Electrophysiol. 2009;20:574-576.
141 Cheng A, Calkins H, Berger RD. Managed ventricular pacing below the lower rate limit: is it simply caused by ventricular-based pacing? Heart Rhythm. 2009;6:1240-1241.
142 Sweeney MO, Ruetz LL, Belk P, et al. Bradycardia pacing-induced short-long-short sequences at the onset of ventricular tachyarrhythmias: a possible mechanism of proarrhythmia? J Am Coll Cardiol. 2007;50:614-622.
143 Vavasis C, Slotwiner DJ, Goldner BG, Cheung JW. Frequent recurrent polymorphic ventricular tachycardia during sleep due to managed ventricular pacing. Pacing Clin Electrophysiol. 2010;33:641-644.
144 Van Mechelen R, Schoonderwoerd R. Risk of managed ventricular pacing in a patient with heart block. Heart Rhythm. 2006;3:1384-1385.
145 Epstein AE, Dimarco JP, Ellenbogen KA, et al. American College of Cardiology/American Heart Association Task Force on Practice; American Association for Thoracic Surgery; Society of Thoracic Surgeons. ACC/AHA/HRS 2008 guidelines for Device-Based Therapy of Cardiac Rhythm Abnormalities: executive summary. Heart Rhythm. 2008;5:934-955.
146 Gillis AM. Redefining physiologic pacing: lessons learned from recent clinical trials. Heart Rhythm. 2006;3:1367-1372.
147 Wilkoff BL, Kudenchuk PJ, Buxton AE, et al. DAVID-II Investigators. DAVID (Dual Chamber and VVI Implantable Defibrillator) II trial. J Am Coll Cardiol. 2009;53:872-880.
148 Kristensen L, Nielsen JC, Pedersen AK, et al. AV block and changes in pacing mode during long-term follow-up of 399 consecutive patients with sick sinus syndrome treated with an AAI/AAIR pacemaker. Pacing Clin Electrophysiol. 2001;24:358-365.
149 Andersen HR, Nielsen JC, Thomsen PE, et al. Atrioventricular conduction during long-term follow-up of patients with sick sinus syndrome. Circulation. 1998;98:1315-1321.
150 De Sisti A, Leclercq JF, Stiubei M, et al. P-wave duration and morphology predict atrial fibrillation recurrence in patients with sinus node dysfunction and atrial-based pacemaker. Pacing Clin Electrophysiol. 2002;25:1546-1554.
151 Gillis AM, Chung MK. Pacing the right ventricle: to pace or not to pace. Heart Rhythm. 2005;2:201-206.
152 Daoud E, Doshi R, Fellows C, et al. Ablate and pace with cardiac resynchronization therapy for patients with reduced ejection fraction: sub-analysis of PAVE study. Heart Rhythm. 2004;1:s59.
153 Stambler BS, Ellenbogen K, Zhang X, et al. ROVA Investigators. Right ventricular outflow versus apical pacing in pacemaker patients with congestive heart failure and atrial fibrillation. J Cardiovasc Electrophysiol. 2003;14:1180-1186.
154 Victor F, Mabo P, Mansour H, et al. A randomized comparison of permanent septal versus apical right ventricular pacing: short-term results. J Cardiovasc Electrophysiol. 2006;17:238-242.
155 Cho HC, Marbán E. Biological therapies for cardiac arrhythmias: can genes and cells replace drugs and devices? Circ Res. 2010;106:674-685.